What Does Ion Mean In Text
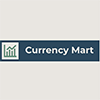
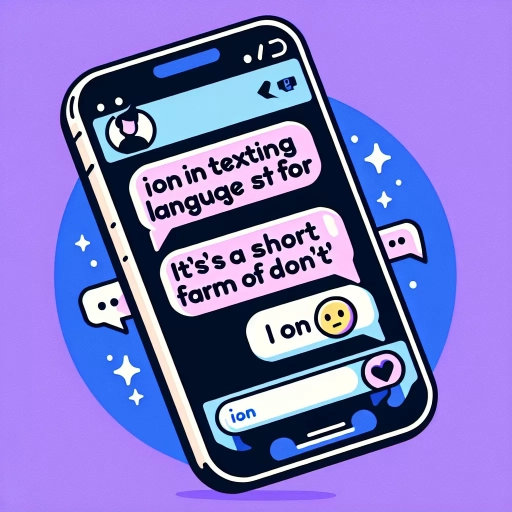
In the vast and intricate world of science, the term "ion" plays a pivotal role across multiple disciplines, from the microscopic realms of chemistry to the practical applications in everyday life. An ion, fundamentally, is an atom or molecule that has gained or lost electrons, resulting in a net positive or negative charge. This simple yet powerful concept has far-reaching implications. In chemistry, understanding ions is crucial for grasping chemical reactions and the formation of compounds. In biology and medicine, ions are essential for various physiological processes, including nerve function and muscle contraction. Moreover, ions find significant use in technology and everyday life, influencing everything from the batteries that power our devices to the water purification systems that ensure our drinking water is safe. This article will delve into these aspects, starting with a foundational exploration of the term "ion" in chemistry, which sets the stage for understanding its broader applications in biology, medicine, and technology. By examining the concept of ions through these lenses, we can appreciate the profound impact this tiny charged particle has on our world. Let's begin by understanding the term "ion" in chemistry.
Understanding the Term "Ion" in Chemistry
Understanding the term "ion" in chemistry is crucial for grasping the fundamental principles of chemical reactions and the behavior of atoms and molecules. An ion is a charged particle formed when an atom or group of atoms gains or loses electrons, resulting in a net positive or negative charge. This concept is multifaceted and can be explored through several key aspects. First, it is essential to delve into the **Definition and Formation of Ions**, which explains how ions are created through the gain or loss of electrons. This understanding sets the stage for comprehending the different types of ions that exist. Next, **Types of Ions: Cations and Anions** highlights the distinction between positively charged cations and negatively charged anions, each playing unique roles in chemical processes. Finally, **Role of Ions in Chemical Reactions** illustrates how ions participate in and influence various chemical interactions, from ionic bonding to complex biochemical processes. By starting with the basics of ion formation, we can build a solid foundation for exploring these critical concepts in depth. Therefore, let us begin by examining the **Definition and Formation of Ions** to lay the groundwork for our comprehensive exploration of ions in chemistry.
Definition and Formation of Ions
**Definition and Formation of Ions** In the realm of chemistry, ions are fundamental entities that play a crucial role in understanding various chemical processes. An ion is an atom or molecule that has gained or lost electrons, resulting in a net positive or negative charge. This alteration in electron count transforms the neutral atom into an ion, which is either cationic (positively charged) if it loses electrons or anionic (negatively charged) if it gains electrons. The formation of ions is a direct consequence of the electron configuration of atoms. According to the octet rule, atoms tend to achieve a stable electron configuration by having eight electrons in their outermost shell, similar to the noble gases. To achieve this stability, atoms can either gain or lose electrons. For instance, sodium (Na) has one electron in its outermost shell and can easily lose this electron to form a positively charged sodium ion (Na⁺). Conversely, chlorine (Cl) has seven electrons in its outermost shell and can gain one electron to form a negatively charged chloride ion (Cl⁻). The process of ion formation is often driven by the desire of atoms to achieve a full outer energy level. When sodium and chlorine react, sodium loses its single outer electron to become Na⁺, while chlorine gains this electron to become Cl⁻. This reaction results in the formation of sodium chloride (NaCl), commonly known as table salt. The electrostatic attraction between the oppositely charged ions holds them together in a crystalline lattice structure. In addition to simple ionic compounds like NaCl, ions can also form from molecules. For example, when hydrogen chloride gas (HCl) dissolves in water, it dissociates into hydrogen ions (H⁺) and chloride ions (Cl⁻). This dissociation is crucial for understanding acid-base chemistry and various biological processes. The properties of ions are significantly different from those of their parent atoms or molecules. Ions exhibit unique chemical reactivity due to their charge, which influences their interactions with other ions and molecules. For instance, positively charged cations are attracted to negatively charged anions, forming ionic bonds that are strong and stable. Understanding the concept of ions is essential for grasping numerous chemical phenomena, including electrolysis, acid-base reactions, and the behavior of ionic compounds in solution. In biological systems, ions such as sodium, potassium, and calcium play critical roles in maintaining cellular functions and nerve signal transmission. The study of ions not only deepens our knowledge of chemical principles but also has practical applications across various fields, from medicine to materials science. In summary, ions are charged particles formed through the gain or loss of electrons by atoms or molecules. Their formation is driven by the quest for stability and adherence to the octet rule. The unique properties and behaviors of ions underpin many fundamental chemical processes and have significant implications for both theoretical understanding and practical applications in chemistry.
Types of Ions: Cations and Anions
In the realm of chemistry, ions are atoms or molecules that have gained or lost electrons, resulting in a net positive or negative charge. This fundamental concept is crucial for understanding various chemical processes and reactions. When delving into the types of ions, it is essential to distinguish between cations and anions. **Cations** are positively charged ions formed when an atom loses one or more electrons. This loss of electrons typically occurs in metals, which tend to have a relatively low number of electrons in their outermost energy level. For instance, sodium (Na) loses one electron to form a sodium ion (Na⁺), while calcium (Ca) loses two electrons to form a calcium ion (Ca²⁺). These cations are highly reactive and often participate in ionic bonding, where they are attracted to negatively charged ions. On the other hand, **anions** are negatively charged ions created when an atom gains one or more electrons. This gain of electrons is common in nonmetals, which often have a high number of electrons in their outermost energy level but are still eager to achieve a stable electron configuration. For example, chlorine (Cl) gains one electron to form a chloride ion (Cl⁻), while oxygen (O) gains two electrons to form an oxide ion (O²⁻). Anions are also integral to ionic bonding, as they are attracted to positively charged cations. The formation of cations and anions is not limited to single atoms; polyatomic ions can also be formed. These are groups of atoms that collectively carry a charge. For instance, the ammonium ion (NH₄⁺) is a polyatomic cation formed when ammonia (NH₃) gains a hydrogen ion (H⁺), while the carbonate ion (CO₃²⁻) is a polyatomic anion formed from carbon dioxide and water. Understanding the distinction between cations and anions is vital for comprehending chemical reactions, such as acid-base reactions and precipitation reactions. In acid-base chemistry, cations like hydrogen ions (H⁺) play a central role in defining acids, while anions like hydroxide ions (OH⁻) are key in defining bases. In precipitation reactions, the combination of cations and anions leads to the formation of insoluble salts. Moreover, the properties of cations and anions influence the physical and chemical characteristics of compounds. For example, the size and charge of ions affect the melting and boiling points of ionic compounds. Smaller ions with higher charges tend to form stronger ionic bonds, resulting in higher melting and boiling points. In summary, cations and anions are fundamental components of ionic chemistry, each playing a unique role in the formation of compounds and the facilitation of chemical reactions. Recognizing the differences between these positively and negatively charged ions is essential for a deeper understanding of chemical principles and processes. This knowledge not only enhances our comprehension of basic chemistry but also underpins more advanced concepts in fields such as biochemistry, materials science, and environmental science.
Role of Ions in Chemical Reactions
In the realm of chemical reactions, ions play a pivotal role that is both fundamental and multifaceted. An ion, as defined in chemistry, is an atom or molecule that has gained or lost electrons, resulting in a net positive or negative charge. This alteration in charge state significantly influences the ion's chemical behavior and reactivity. When ions are involved in chemical reactions, they can act as reactants, products, or even catalysts, facilitating the transformation of substances in various ways. One of the primary roles of ions in chemical reactions is their ability to participate in ionic bonding. In ionic compounds, positively charged cations (typically metal ions) and negatively charged anions (often nonmetal ions) are attracted to each other due to electrostatic forces. This attraction leads to the formation of strong ionic bonds, which are crucial for the structure and stability of many inorganic compounds. For instance, sodium chloride (NaCl), or common table salt, is formed when sodium ions (Na⁺) combine with chloride ions (Cl⁻), illustrating how ionic interactions can lead to the creation of stable and widely used substances. Ions also play a critical role in aqueous solutions, where they can dissolve and move freely. In such environments, ions can participate in various types of chemical reactions, including acid-base reactions and redox (reduction-oxidation) reactions. For example, in acid-base chemistry, hydrogen ions (H⁺) and hydroxide ions (OH⁻) are key players. The concentration of hydrogen ions determines the acidity of a solution, while hydroxide ions influence its basicity. These ionic species can react with each other to form water, a process that is essential for maintaining pH balance in biological systems and industrial processes. Furthermore, ions can act as catalysts or intermediates in complex reaction pathways. In enzymatic reactions, metal ions such as zinc (Zn²⁺), magnesium (Mg²⁺), and iron (Fe²⁺ or Fe³⁺) often serve as cofactors that enhance the catalytic activity of enzymes. These metal ions can stabilize transition states, facilitate electron transfer, or directly participate in the chemical transformation of substrates into products. For instance, the enzyme carbonic anhydrase relies on a zinc ion to catalyze the rapid interconversion of carbon dioxide and bicarbonate, a process vital for respiration and blood pH regulation. Additionally, ions are integral to electrochemical processes. In batteries and fuel cells, ions move through electrolytes to facilitate the flow of electrical charge. For example, in a lithium-ion battery, lithium ions (Li⁺) migrate between the anode and cathode during charge and discharge cycles, enabling the storage and release of electrical energy. This ionic movement is crucial for powering modern electronic devices and vehicles. In summary, the role of ions in chemical reactions is multifaceted and indispensable. Whether forming ionic bonds, participating in aqueous reactions, acting as catalysts, or facilitating electrochemical processes, ions are central to understanding and predicting the outcomes of various chemical transformations. Their unique properties and behaviors make them essential components in both natural biological systems and industrial applications, underscoring their significance in the broader field of chemistry.
The Concept of Ions in Biology and Medicine
The concept of ions in biology and medicine is a multifaceted and crucial aspect of understanding how living organisms function and maintain health. Ions, charged atoms or molecules, play pivotal roles in various cellular processes, ion channels and transport mechanisms, and have significant medical applications. At the cellular level, ions are essential for maintaining proper membrane potential, regulating fluid balance, and facilitating nerve and muscle function. Ion channels and transport mechanisms are intricate systems that govern the movement of ions across cell membranes, ensuring that cells operate within optimal conditions. Beyond their cellular roles, ions have profound implications in medicine; their imbalance can lead to a range of disorders, from neurological conditions to cardiovascular diseases. Understanding these aspects is vital for developing treatments and therapies aimed at restoring ion balance and promoting health. This article delves into these critical areas, starting with the fundamental role of ions in cellular processes, which sets the stage for exploring their broader biological and medical significance.
Ions in Cellular Processes
Ions play a pivotal role in various cellular processes, serving as essential components that facilitate numerous biological functions. In the context of cellular biology, ions are atoms or molecules that have gained or lost electrons, resulting in a net positive or negative charge. These charged particles are crucial for maintaining cellular homeostasis and enabling the proper functioning of cells. One of the most significant roles of ions is in the regulation of membrane potential. The cell membrane, which separates the intracellular environment from the extracellular space, is selectively permeable and allows certain ions to pass through while restricting others. Potassium (K+), sodium (Na+), calcium (Ca2+), and chloride (Cl-) ions are particularly important in this regard. For instance, the resting membrane potential is primarily maintained by the differential distribution of potassium and sodium ions across the cell membrane, with potassium ions being more concentrated inside the cell and sodium ions outside. This ionic gradient is established and maintained by ion pumps and channels, such as the sodium-potassium pump, which actively transports sodium out of the cell and potassium into the cell against their concentration gradients. Ions also play a critical role in neuronal signaling and muscle contraction. In neurons, the rapid influx of sodium ions during an action potential allows for the propagation of electrical signals along the axon, enabling communication between neurons. Following this depolarization phase, potassium channels open to allow potassium ions to leave the cell, repolarizing the membrane and returning it to its resting state. Similarly, in muscle cells, calcium ions are key regulators of muscle contraction. When a muscle cell is stimulated by a nerve impulse, calcium ions are released from intracellular stores into the cytoplasm, where they bind to troponin and tropomyosin proteins on actin filaments, causing muscle contraction. In addition to their roles in electrical signaling and muscle function, ions are involved in various metabolic processes within cells. For example, magnesium ions (Mg2+) are cofactors for many enzymes involved in energy metabolism, such as those participating in glycolysis and the citric acid cycle. Phosphate ions (PO42-) are integral components of ATP (adenosine triphosphate), the primary energy currency of cells. Furthermore, ions influence cellular volume regulation and osmotic balance. Cells must maintain an appropriate balance between water and solutes to prevent excessive swelling or shrinkage. Ions help regulate this balance by influencing water movement across cell membranes according to osmotic gradients. In medical contexts, disturbances in ion balance can lead to significant health issues. For instance, hyperkalemia (elevated potassium levels) can cause cardiac arrhythmias due to its effect on heart muscle function, while hypocalcemia (low calcium levels) can lead to muscle cramps and tetany due to impaired neuromuscular transmission. In conclusion, ions are indispensable for a wide range of cellular processes including membrane potential regulation, neuronal signaling, muscle contraction, metabolic pathways, and osmotic balance. Their precise regulation is vital for maintaining cellular homeostasis and overall health. Understanding the roles of ions in biology not only deepens our appreciation for cellular mechanisms but also provides insights into potential therapeutic strategies for treating ion-related disorders.
Ion Channels and Transport Mechanisms
Ion channels and transport mechanisms are crucial components in the intricate dance of cellular communication and homeostasis, playing a pivotal role in the broader concept of ions in biology and medicine. Ion channels are specialized proteins embedded within cell membranes that facilitate the selective passage of ions across these barriers, thereby regulating various cellular processes. These channels can be voltage-gated, ligand-gated, or mechanically gated, each type responding to different stimuli to open or close, allowing ions such as sodium, potassium, calcium, and chloride to flow through. For instance, voltage-gated sodium channels are essential for the initiation and propagation of action potentials in neurons, enabling rapid electrical signaling that underpins neural function. Similarly, potassium channels help repolarize the cell membrane after an action potential, restoring the resting membrane potential. In addition to ion channels, cells employ various transport mechanisms to manage ion concentrations. Active transport involves the use of energy, typically in the form of ATP, to move ions against their concentration gradient. The sodium-potassium pump is a classic example of active transport; it expels three sodium ions out of the cell while importing two potassium ions, maintaining the electrochemical gradient necessary for proper cellular function. Passive transport, on the other hand, relies on diffusion down concentration gradients and can occur through facilitated diffusion via carrier proteins or ion channels. For example, the glucose-sodium cotransporter uses the energy from sodium ions flowing down their concentration gradient to transport glucose into cells against its concentration gradient. The balance of ions within cells is vital for maintaining proper cellular function and overall health. Dysregulation of ion channels and transport mechanisms can lead to a myriad of diseases. For instance, mutations in ion channel genes can result in conditions such as cystic fibrosis (due to defective chloride channels) or long QT syndrome (resulting from abnormal potassium channels). Moreover, ion imbalances are implicated in neurological disorders like epilepsy and muscular dystrophy. Understanding these mechanisms not only sheds light on the fundamental biology of cells but also opens avenues for therapeutic interventions. Drugs targeting specific ion channels or transporters can be designed to treat various conditions; for example, calcium channel blockers are used to manage hypertension by reducing vascular smooth muscle contraction. In medicine, the study of ion channels and transport mechanisms has led to significant advancements in diagnostics and treatments. Electrophysiology techniques such as electrocardiograms (ECGs) and electromyograms (EMGs) rely on measuring the electrical activity generated by ion fluxes across cell membranes. These tools are indispensable for diagnosing cardiac arrhythmias and muscle disorders. Furthermore, research into ion channelopathies—diseases caused by dysfunctional ion channels—has expanded our understanding of disease pathophysiology and guided the development of targeted therapies. In conclusion, ion channels and transport mechanisms are essential for maintaining cellular homeostasis and facilitating communication between cells. Their dysregulation can lead to various diseases, making them critical targets for medical research and therapeutic intervention. As our understanding of these complex systems evolves, so too do our capabilities to diagnose and treat a wide range of conditions, underscoring the importance of ions in biology and medicine.
Medical Applications and Ion Imbalance Disorders
In the realm of biology and medicine, ions play a crucial role in maintaining the delicate balance necessary for optimal physiological function. Ion imbalance disorders, therefore, can have profound implications on health and well-being. One of the most significant medical applications of understanding ions is in the diagnosis and treatment of electrolyte imbalances. Electrolytes, such as sodium, potassium, calcium, and magnesium, are essential ions that regulate various bodily functions including nerve and muscle function, hydration levels, and pH balance. For instance, hypernatremia (elevated sodium levels) can lead to severe dehydration and neurological symptoms, while hyponatremia (low sodium levels) can cause seizures and coma. Similarly, hyperkalemia (high potassium levels) can lead to cardiac arrhythmias and even heart failure if left untreated. Hypokalemia (low potassium levels), on the other hand, can result in muscle weakness and respiratory difficulties. Medical professionals use ion-selective electrodes and other diagnostic tools to measure electrolyte levels accurately, enabling timely interventions such as intravenous fluid therapy or medication adjustments. Ion imbalances are also closely linked to several chronic conditions. For example, patients with kidney disease often suffer from electrolyte disturbances due to impaired renal function. In diabetes mellitus, hyperglycemia can lead to osmotic shifts that disrupt ion balances. Additionally, certain medications like diuretics can cause electrolyte imbalances as a side effect. Understanding these relationships allows healthcare providers to monitor patients more effectively and adjust treatment plans accordingly. Moreover, advances in medical technology have enabled the development of ion-sensitive devices that aid in real-time monitoring of electrolyte levels. For instance, implantable cardioverter-defibrillators (ICDs) use ion-sensitive sensors to detect abnormal heart rhythms caused by ion imbalances. Similarly, continuous glucose monitoring systems rely on ion-sensitive electrodes to measure glucose levels in diabetic patients. In therapeutic applications, ion channels and pumps are targets for various medications. For example, beta-blockers work by inhibiting calcium channels in the heart to reduce blood pressure and heart rate. Similarly, certain antidepressants modulate ion channels in neurons to stabilize mood. The precise manipulation of ions through pharmacological means underscores the critical role these charged particles play in maintaining health. In conclusion, the concept of ions in biology and medicine is fundamental to understanding numerous physiological processes and diagnosing/treating a wide range of medical conditions. Ion imbalance disorders highlight the importance of maintaining electrolyte homeostasis for optimal health outcomes. By leveraging advanced diagnostic tools and therapeutic strategies that target ion channels and balances, healthcare providers can offer more effective care for patients suffering from these disorders. This intricate interplay between ions and biological systems underscores why a deep understanding of ion dynamics is essential in modern medicine.
Ions in Everyday Life and Technology
Ions play a pivotal role in various aspects of our daily lives and technological advancements. From ensuring the purity of our drinking water to powering the devices we rely on, ions are integral components that drive innovation and sustainability. In water purification systems, ions are crucial for removing contaminants and minerals, making water safe for consumption. This process highlights the importance of ionic interactions in maintaining public health. Additionally, ions are at the heart of battery technology and energy storage, enabling the efficient charging and discharging of batteries that power everything from smartphones to electric vehicles. Their role in environmental science and pollution control is equally significant, as ions help in monitoring and mitigating the effects of pollutants in our ecosystems. Understanding these applications not only underscores the versatility of ions but also emphasizes their critical impact on our daily lives. As we delve into these topics, let's first explore how ions in water purification systems contribute to our health and well-being.
Ions in Water Purification Systems
In the realm of water purification systems, ions play a crucial role in ensuring the quality and safety of drinking water. Ions are atoms or molecules that have gained or lost electrons, resulting in a net positive or negative charge. In water purification, these charged particles are leveraged to remove contaminants and improve water quality. One of the primary methods involving ions is ion exchange, where resins are used to capture and replace ions in the water. For instance, sodium ions in the resin can be exchanged for calcium and magnesium ions in the water, reducing hardness and scaling potential. This process is particularly effective in removing heavy metals and other inorganic compounds that can be harmful to human health. Another significant application of ions in water purification is the use of reverse osmosis (RO) systems. RO membranes have tiny pores that allow water molecules to pass through while blocking larger particles, including many ions. However, some ions can still pass through these membranes, necessitating additional treatment steps such as deionization. Deionization involves passing the water through resins that remove virtually all ions, resulting in highly purified water suitable for various industrial and laboratory applications. Electrolysis is another technique that utilizes ions to purify water. By applying an electric current, electrolysis can split water into hydrogen and oxygen gases, a process that also helps in breaking down organic pollutants. Additionally, electrocoagulation uses electrical currents to generate ions that coagulate suspended particles and contaminants, making them easier to remove from the water. The presence of certain ions can also affect the taste and odor of water. For example, high levels of chloride ions can give water a salty taste, while sulfate ions can impart a bitter taste. Therefore, ion removal or adjustment is often necessary to achieve palatable drinking water. Moreover, advanced technologies such as nanofiltration and ultrafiltration membranes rely on the selective permeability of ions to filter out impurities. These membranes have specific pore sizes that allow certain ions to pass through while blocking others, ensuring that the purified water meets stringent quality standards. In summary, ions are integral to various water purification processes due to their ability to interact with contaminants and facilitate their removal. Whether through ion exchange, reverse osmosis, electrolysis, or advanced filtration techniques, the manipulation of ions is a critical step in producing clean and safe drinking water. This underscores the importance of understanding and managing ions in everyday life and technology, particularly in ensuring access to high-quality water resources.
Ions in Battery Technology and Energy Storage
In the realm of battery technology and energy storage, ions play a pivotal role in enabling the efficient and reliable operation of devices that power our modern world. At the heart of this technology are ion batteries, such as lithium-ion (Li-ion) batteries, which dominate the market due to their high energy density, long cycle life, and relatively low self-discharge rate. Here’s how ions contribute to this critical field: 1. **Ion Movement and Electrochemical Reactions**: In a Li-ion battery, lithium ions (Li+) are the key players. During discharge, these ions move from the anode (typically graphite) through an electrolyte to the cathode (often a lithium metal oxide). This movement facilitates an electrochemical reaction that generates electricity. Conversely, during charging, the process is reversed, with lithium ions returning to the anode. This continuous flow of ions between electrodes allows for the storage and release of electrical energy. 2. **Energy Storage Mechanism**: The efficiency of ion movement is crucial for battery performance. The electrolyte, which can be a liquid, gel, or solid, must be highly conductive to ions while being electronically insulating to prevent short circuits. Advanced materials and designs, such as solid-state electrolytes and nanostructured electrodes, are being developed to enhance ion mobility and reduce internal resistance, thereby improving overall battery efficiency and safety. 3. **Safety and Stability**: Ion behavior also impacts battery safety. For instance, thermal runaway in Li-ion batteries can occur if ions become trapped or if there is an imbalance in ion distribution, leading to overheating and potential fires. To mitigate this risk, manufacturers incorporate safety features like separators that prevent physical contact between electrodes and thermal management systems that regulate temperature. 4. **Future Innovations**: Research into new ion chemistries and materials is driving innovation in battery technology. For example, sodium-ion batteries are being explored as a potentially more cost-effective alternative to lithium-ion batteries, given the abundance of sodium. Similarly, solid-state batteries promise higher energy density and improved safety by replacing the liquid electrolyte with a solid material that enhances ion conductivity while reducing the risk of leakage or explosion. 5. **Impact on Everyday Life**: The impact of ions in battery technology extends far beyond the devices themselves; it influences how we live and work. From powering smartphones and laptops to enabling electric vehicles and renewable energy systems, ion-based batteries are integral to modern life. They support remote work, global communication, and sustainable energy solutions, making them a cornerstone of contemporary technology. In summary, ions are the linchpin of advanced battery technology and energy storage. Their movement and interaction within electrochemical systems underpin the functionality of devices that are essential to our daily lives. As research continues to advance, we can expect even more efficient, safer, and sustainable ion-based solutions that will further transform how we harness and utilize energy.
Ions in Environmental Science and Pollution Control
In the realm of environmental science and pollution control, ions play a crucial role in understanding and mitigating the impact of pollutants on ecosystems. Ions are atoms or molecules that have gained or lost electrons, resulting in a net positive or negative charge. This property makes them highly reactive and influential in various environmental processes. For instance, ions such as sodium (Na+), chloride (Cl-), and sulfate (SO4^2-) are common in natural waters but can become problematic when their concentrations are altered by human activities. Excessive levels of these ions can lead to water pollution, affecting aquatic life and human health. In soil science, ions are essential for nutrient cycling and plant growth. Nutrient ions like nitrate (NO3-), phosphate (PO4^3-), and potassium (K+) are vital for plant nutrition but can also contribute to soil degradation if overused in agricultural practices. The leaching of these ions into groundwater can lead to eutrophication in water bodies, causing harmful algal blooms that deplete oxygen levels and harm aquatic ecosystems. Ion exchange processes are also critical in environmental remediation. For example, ion exchange resins are used to remove heavy metal ions such as lead (Pb^2+) and mercury (Hg^2+) from contaminated water sources. These resins can selectively bind to specific ions, allowing for their removal and subsequent safe disposal. Similarly, natural systems like wetlands and forests utilize ion exchange mechanisms to filter out pollutants from water. In air pollution control, ions are involved in the formation of acid rain. Sulfur dioxide (SO2) and nitrogen oxides (NOx) emitted from industrial activities react with water vapor in the atmosphere to form sulfuric acid (H2SO4) and nitric acid (HNO3), leading to acidic precipitation. These acidic ions can have devastating effects on forests, lakes, and soil chemistry. Furthermore, advanced technologies such as electrochemical treatment rely on ions to clean polluted water. Electrocoagulation, for example, uses electrical currents to generate ions that coagulate pollutants, making them easier to remove from wastewater. This method is particularly effective for treating industrial effluents containing heavy metals and organic contaminants. In summary, ions are integral to understanding and addressing environmental pollution. Their reactivity and mobility make them key players in water, soil, and air quality issues. By leveraging ion chemistry through various technologies and natural processes, scientists and policymakers can develop more effective strategies for pollution control and environmental conservation. This underscores the importance of ions not only in everyday life but also in the broader context of environmental science and technology.