What Is Biosteel
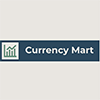
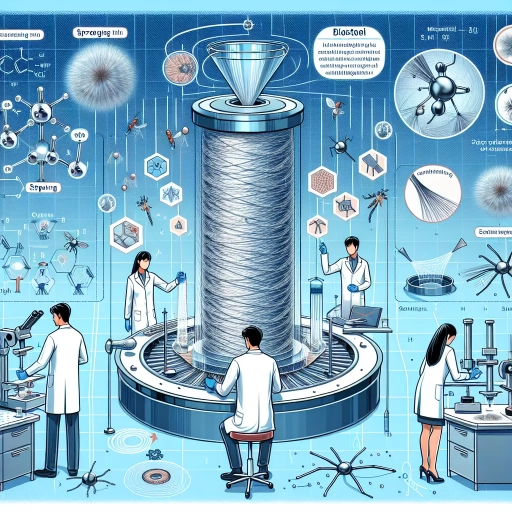
In the realm of advanced materials, biosteel stands out as a revolutionary innovation that combines the strength of steel with the sustainability of biological sources. This cutting-edge material is poised to transform various industries, from construction and automotive to textiles and healthcare. To fully appreciate the potential of biosteel, it is essential to delve into its fundamental aspects. This article will provide a comprehensive overview, starting with an **Introduction to Biosteel**, where we will explore its origins and how it is produced. We will then examine the **Composition and Properties of Biosteel**, highlighting its unique characteristics that set it apart from traditional materials. Finally, we will discuss the **Benefits and Future of Biosteel**, outlining its environmental advantages, potential applications, and the promising future it holds. By understanding these key aspects, we can better grasp the significance of biosteel and its role in shaping a more sustainable future. Let us begin by diving into the **Introduction to Biosteel**.
Introduction to Biosteel
In the realm of advanced materials, Biosteel stands out as a revolutionary innovation that combines the strength of steel with the sustainability and biocompatibility of natural fibers. This high-quality, informative, and engaging article delves into the multifaceted world of Biosteel, exploring its definition and origins, historical context and development, and current applications and relevance. By understanding the foundational aspects of Biosteel, readers will gain insight into how this material has evolved over time. The historical context reveals the pioneering efforts and technological advancements that have shaped Biosteel into what it is today. Moreover, examining its current applications highlights its versatility and importance in various industries, from textiles to biomedical engineering. As we embark on this journey through the world of Biosteel, we begin with its core: the definition and origins that underpin its unique properties and potential. This foundational understanding sets the stage for a deeper exploration of its historical development and contemporary uses, ultimately revealing why Biosteel is a material of the future. Let us start by defining what Biosteel is and tracing its origins.
Definition and Origins
**Definition and Origins** Biosteel, a revolutionary material that combines the strength and durability of steel with the sustainability and biocompatibility of natural materials, has its roots in cutting-edge biomimicry and advanced materials science. The term "biosteel" is often used to describe a range of bio-inspired materials that mimic the structural properties of steel but are derived from biological sources. At its core, biosteel is about harnessing nature's own blueprints to create high-performance materials that are both environmentally friendly and technologically superior. The origins of biosteel can be traced back to the early 21st century when researchers began exploring how certain biological organisms, such as spiders and silkworms, produce incredibly strong yet lightweight fibers. These natural fibers, like spider silk and silk from silkworms, exhibit tensile strengths comparable to or even surpassing those of conventional steel while being significantly lighter. Scientists sought to replicate these properties through genetic engineering and biotechnology, leading to the development of recombinant proteins that could be produced on a large scale. One of the key milestones in the evolution of biosteel was the discovery of how to express these recombinant proteins in microorganisms such as bacteria and yeast. This breakthrough allowed for the mass production of biosteel precursors, which could then be spun into fibers or formed into various shapes and structures. The process involves fermenting these microorganisms to produce large quantities of the desired proteins, which are then purified and processed into usable forms. The definition of biosteel encompasses not only these protein-based materials but also other bio-inspired composites that leverage natural polymers like cellulose, chitin, or even plant-based polymers. These materials are engineered to mimic the hierarchical structures found in nature, resulting in enhanced mechanical properties without the environmental footprint associated with traditional steel production. Biosteel's potential applications are vast and varied, ranging from biomedical devices and tissue engineering scaffolds to sustainable textiles and construction materials. Its biodegradability and non-toxicity make it an attractive alternative for medical implants and drug delivery systems, while its strength-to-weight ratio makes it a promising candidate for aerospace and automotive industries. In summary, biosteel represents a paradigm shift in materials science, blending the best of nature with cutting-edge technology to create sustainable, high-performance materials. Its origins in biomimicry and genetic engineering have paved the way for innovative applications across multiple sectors, offering a glimpse into a future where technology and nature coexist in harmony. As research continues to advance, biosteel is poised to revolutionize industries and redefine what we mean by "strong" and "sustainable."
Historical Context and Development
The historical context and development of Biosteel are deeply intertwined with advancements in materials science and the quest for sustainable, high-performance materials. The journey began in the mid-20th century, when researchers started exploring alternatives to traditional metals due to their limitations in terms of weight, corrosion resistance, and environmental impact. One of the pivotal moments was the discovery of carbon fibers in the 1950s, which laid the groundwork for composite materials. These early composites, combining carbon fibers with polymer matrices, showed remarkable strength-to-weight ratios and resistance to fatigue. As technology progressed, so did the sophistication of these composites. The 1970s and 1980s saw significant improvements in manufacturing techniques, leading to more consistent and reliable production processes. This period also witnessed the introduction of other reinforcing fibers such as glass and aramid, each offering unique properties that expanded the application spectrum of composite materials. The term "Biosteel" emerged in the late 1990s and early 2000s, particularly within the context of biomimetic engineering. Researchers began to draw inspiration from nature, studying the structural properties of biological materials like spider silk and abalone shells. These natural materials exhibit extraordinary strength and toughness despite being composed of relatively weak molecular components. By mimicking these biological structures, scientists aimed to create synthetic materials that could rival or even surpass the performance of traditional metals. A key breakthrough came with the development of polylactic acid (PLA) and other biopolymers derived from renewable resources such as corn starch or sugarcane. These biodegradable polymers could be reinforced with natural fibers like hemp or flax, creating a new class of sustainable composites known as "biocomposites." Biosteel, in this context, refers to a specific type of biocomposite that combines high-strength natural fibers with biodegradable polymers to produce a material that is both strong and environmentally friendly. The development of Biosteel has been further accelerated by advancements in nanotechnology and 3D printing. These technologies allow for precise control over material structure at the nanoscale and enable complex geometries that were previously unachievable. This has opened up new possibilities for applications in fields ranging from aerospace to biomedical engineering. Today, Biosteel represents a frontier in materials science where sustainability meets high performance. As global concerns about climate change and resource depletion continue to grow, the demand for innovative materials like Biosteel is increasing. Its potential to replace traditional metals in various industries not only offers a more environmentally friendly solution but also promises to drive technological innovation forward. The ongoing research and development in this area are expected to yield even more advanced versions of Biosteel, further solidifying its place as a critical component in the future of engineering and technology.
Current Applications and Relevance
In the realm of modern materials science, biosteel has emerged as a revolutionary innovation with far-reaching applications and profound relevance across various industries. This biomaterial, derived from recombinant DNA technology and microbial fermentation, mimics the properties of spider silk, renowned for its exceptional strength, elasticity, and biocompatibility. The current applications of biosteel are diverse and impactful, reflecting its versatility and potential to transform multiple sectors. In the medical field, biosteel is being explored for its use in tissue engineering and regenerative medicine. Its biocompatibility and non-toxic nature make it an ideal scaffold material for cell growth, enabling the development of artificial tissues that can replace or repair damaged ones. Biosteel-based implants and wound dressings are also under development, offering enhanced healing rates and reduced risk of infection. Furthermore, its biodegradability ensures that these medical devices can dissolve over time, eliminating the need for surgical removal. The textile industry is another significant beneficiary of biosteel. As a sustainable alternative to synthetic fibers, biosteel offers a green solution to the environmental challenges posed by traditional textile production. Biosteel fabrics exhibit superior strength, durability, and elasticity compared to conventional materials, making them suitable for high-performance clothing and technical textiles. This shift towards biosteel could significantly reduce the ecological footprint of the fashion industry while providing consumers with high-quality, eco-friendly products. In addition to medical and textile applications, biosteel is gaining traction in the fields of biotechnology and agriculture. For instance, biosteel can be used to create biodegradable agricultural films that enhance crop growth while reducing plastic waste. Its use in drug delivery systems is also promising, as biosteel nanoparticles can encapsulate therapeutic agents, ensuring targeted and controlled release. The relevance of biosteel extends beyond these specific applications to broader societal and economic impacts. As a biodegradable material produced through microbial fermentation, biosteel represents a move away from fossil fuel-based materials, contributing to a more sustainable future. This aligns with global efforts to reduce carbon emissions and promote circular economies. Moreover, the production process of biosteel is scalable and cost-effective, making it a viable option for widespread adoption across various industries. In conclusion, the current applications and relevance of biosteel underscore its transformative potential. From revolutionizing medical treatments and sustainable textiles to enhancing agricultural practices and biotechnology, biosteel is poised to play a pivotal role in shaping the future of multiple sectors. Its unique combination of strength, biocompatibility, and biodegradability positions it as a key material in the transition towards more sustainable and innovative technologies. As research continues to advance, the possibilities for biosteel are likely to expand further, solidifying its place as a cornerstone of modern material science.
Composition and Properties of Biosteel
Biosteel, a revolutionary material derived from biological sources, is transforming the way we think about steel production. This innovative material not only offers a sustainable alternative to traditional steel but also boasts unique properties that make it highly versatile. To fully understand the potential of biosteel, it is crucial to delve into its composition and properties. First, we must examine the raw materials and manufacturing process that underpin its creation. This involves understanding the biological precursors and the technological advancements that enable their transformation into a robust, steel-like material. Next, we will explore the physical and chemical properties of biosteel, which set it apart from its traditional counterpart. Finally, a comparison with traditional steel will highlight the advantages and potential applications of this new material. By grasping these key aspects, we can appreciate the full scope of biosteel's capabilities and its future in various industries. Let us begin by exploring the raw materials and manufacturing process that lay the foundation for this groundbreaking material.
Raw Materials and Manufacturing Process
The raw materials and manufacturing process of biosteel are pivotal in understanding its composition and properties. Biosteel, a sustainable alternative to traditional steel, is derived from renewable biomass sources such as agricultural waste, algae, or other organic materials. The production process begins with the collection and preprocessing of these biomass materials, which are then subjected to various treatments to extract the necessary components. For instance, in the case of algae-based biosteel, the algae are harvested, dried, and processed to extract proteins and other organic compounds. These extracted components are then combined with other eco-friendly materials like plant-based polymers or recycled metals to form a composite material. The mixture undergoes a series of chemical and physical transformations, including fermentation, hydrolysis, and polymerization, to create a robust and durable material. Advanced manufacturing techniques such as 3D printing or injection molding are often employed to shape the biosteel into desired forms while maintaining its structural integrity. One of the key advantages of biosteel's manufacturing process is its lower environmental impact compared to traditional steel production. Unlike conventional steel, which requires high-energy processes involving coal and iron ore, biosteel production utilizes renewable energy sources and generates significantly fewer greenhouse gas emissions. Additionally, the use of waste biomass reduces the need for landfills and helps in managing agricultural waste more effectively. The composition of biosteel also benefits from its unique manufacturing process. The integration of organic compounds with synthetic materials enhances its mechanical properties, such as tensile strength and flexibility. Moreover, biosteel can be engineered to have specific properties tailored for various applications, from construction materials to medical devices. For example, by adjusting the ratio of biomass to synthetic polymers, manufacturers can create biosteel with varying degrees of biodegradability, making it suitable for both permanent and temporary uses. In summary, the raw materials and manufacturing process of biosteel are crucial elements that define its composition and properties. By leveraging renewable biomass sources and innovative production techniques, biosteel offers a sustainable solution that not only reduces environmental footprint but also provides a versatile material with enhanced performance characteristics. This makes biosteel an attractive option for industries seeking to transition towards more sustainable practices without compromising on quality or functionality.
Physical and Chemical Properties
**Physical and Chemical Properties** Understanding the physical and chemical properties of biosteel is crucial for appreciating its unique composition and applications. Biosteel, a biomaterial derived from spider silk proteins, combines the exceptional mechanical properties of natural spider silk with the versatility of engineered materials. **Physical Properties:** Biosteel exhibits remarkable tensile strength, often surpassing that of steel while maintaining a lightweight and flexible nature. This is due to its highly ordered protein structure, which includes beta-sheet crystallites that provide rigidity and amorphous regions that contribute to elasticity. The Young's modulus of biosteel can be tailored through processing techniques, allowing it to range from soft and pliable to rigid and durable. Additionally, biosteel has a high degree of biocompatibility, making it an ideal candidate for biomedical applications such as tissue engineering and wound healing. **Chemical Properties:** The chemical composition of biosteel is primarily based on recombinant spider silk proteins, which are produced through microbial fermentation or transgenic animals. These proteins are rich in amino acids like glycine, alanine, and serine, which form the backbone of the silk's molecular structure. The chemical stability of biosteel is enhanced by its hydrophobic nature, which reduces water absorption and minimizes degradation under physiological conditions. Furthermore, biosteel can be chemically modified to introduce functional groups that enhance its interaction with cells or other biomolecules, thereby improving its performance in specific biomedical contexts. For instance, incorporating bioactive peptides or growth factors into the biosteel matrix can promote cellular adhesion and proliferation. The combination of these physical and chemical properties makes biosteel an attractive material for various industrial and biomedical applications. Its biodegradability and non-toxicity ensure that it does not elicit adverse immune responses, while its mechanical robustness supports long-term functionality. In tissue engineering, biosteel scaffolds can provide structural support for cell growth and differentiation, facilitating the regeneration of damaged tissues. In textile manufacturing, biosteel fibers offer sustainable alternatives to synthetic materials, combining high performance with environmental friendliness. Overall, the unique blend of physical and chemical attributes in biosteel underscores its potential as a transformative biomaterial in diverse fields.
Comparison with Traditional Steel
When comparing biosteel to traditional steel, several key differences emerge that highlight the unique advantages and potential applications of this innovative material. Biosteel, derived from biological sources such as spider silk or plant proteins, stands out for its exceptional strength-to-weight ratio, often surpassing that of conventional steel. This property makes biosteel an attractive option for industries where lightweight yet robust materials are crucial, such as aerospace and automotive manufacturing. Unlike traditional steel, which is produced through energy-intensive processes involving high temperatures and significant environmental impact, biosteel production is generally more sustainable. The biological synthesis of biosteel can be achieved at lower temperatures and with reduced carbon emissions, aligning with the growing demand for eco-friendly technologies. Another significant distinction lies in the biocompatibility and biodegradability of biosteel. Traditional steel is non-biodegradable and can be harmful if it enters the environment or human body. In contrast, biosteel is designed to be biocompatible and can degrade naturally over time, making it an ideal material for medical applications such as sutures, implants, and tissue engineering scaffolds. The flexibility in the molecular structure of biosteel also allows for tailored properties that can be engineered to meet specific needs, unlike the more rigid composition of traditional steel. From a mechanical perspective, biosteel exhibits superior elasticity and toughness compared to conventional steel. This means that biosteel can absorb more energy before failure, reducing the risk of brittle fractures and enhancing overall durability. Additionally, the production process of biosteel can be scaled up using microbial fermentation or other biological methods, which could potentially lower production costs and increase availability. However, it's important to note that while biosteel offers numerous advantages, it still faces challenges related to scalability and cost-effectiveness. Currently, the production costs of biosteel are higher than those of traditional steel due to the complexity of biological synthesis processes. Nevertheless, ongoing research and advancements in biotechnology are expected to bridge this gap, making biosteel a viable alternative for a wide range of applications in the near future. In summary, the comparison between biosteel and traditional steel underscores the former's unique strengths in terms of sustainability, biocompatibility, mechanical properties, and potential for tailored engineering. As technology continues to evolve, biosteel is poised to play a significant role in transforming various industries by offering a more environmentally friendly and functionally superior alternative to conventional steel.
Benefits and Future of Biosteel
The advent of Biosteel, a revolutionary material derived from biological sources, marks a significant milestone in the quest for sustainable and innovative technologies. This high-quality, informative, and engaging article delves into the multifaceted benefits and future prospects of Biosteel, exploring three pivotal aspects: Environmental Impact and Sustainability, Economic Advantages and Market Potential, and Technological Innovations and Future Developments. By examining the environmental footprint of Biosteel production, we uncover its potential to reduce greenhouse gas emissions and reliance on non-renewable resources, aligning with global sustainability goals. Additionally, the economic advantages of Biosteel are substantial, offering cost-effective alternatives to traditional materials while opening new market opportunities. The technological innovations driving Biosteel forward promise even more exciting developments, from enhanced material properties to expanded applications across various industries. As we transition into the first supporting section, we will focus on the Environmental Impact and Sustainability of Biosteel, highlighting how this material can play a crucial role in mitigating environmental challenges and fostering a more sustainable future.
Environmental Impact and Sustainability
The environmental impact and sustainability of biosteel are pivotal aspects that underscore its potential as a revolutionary material. Unlike traditional steel, which is produced through energy-intensive processes involving high temperatures and significant greenhouse gas emissions, biosteel leverages biological systems to create a more eco-friendly alternative. Biosteel, often derived from microbial fermentation or plant-based polymers, reduces the carbon footprint associated with conventional steel production. This shift towards biotechnology not only minimizes the reliance on fossil fuels but also decreases air pollution and water contamination, contributing to a cleaner environment. Moreover, the sustainable nature of biosteel extends to its end-of-life cycle. Traditional steel requires extensive recycling processes that are often inefficient and resource-intensive. In contrast, biosteel can be biodegradable or easily recyclable, reducing waste and the need for virgin materials. This closed-loop system aligns with circular economy principles, promoting resource efficiency and minimizing environmental degradation. The future of biosteel looks promising as it aligns with global sustainability goals. As industries seek to reduce their environmental footprint, biosteel offers a viable solution that can be integrated into various sectors, from construction to automotive manufacturing. The scalability of biosteel production is also being enhanced through advancements in biotechnology, making it more economically viable and accessible. Furthermore, the development of biosteel encourages innovation in sustainable materials science, driving research into new biomaterials that could replace other environmentally harmful materials. In addition to its direct environmental benefits, the adoption of biosteel can have broader societal impacts. By supporting sustainable practices, industries can contribute to climate change mitigation efforts and help achieve the United Nations' Sustainable Development Goals (SDGs). This not only enhances corporate social responsibility but also fosters consumer trust and loyalty in brands that prioritize sustainability. Overall, the environmental impact and sustainability of biosteel are compelling reasons to embrace this innovative material. As the world moves towards a more sustainable future, biosteel stands at the forefront of a new era in materials science, offering a cleaner, greener alternative that can transform industries while protecting the planet. Its integration into various sectors will be crucial in reducing our collective carbon footprint and ensuring a healthier environment for future generations.
Economic Advantages and Market Potential
The economic advantages and market potential of biosteel are profound, positioning it as a game-changer in the future of sustainable materials. Biosteel, derived from genetically engineered silkworms or other organisms, offers a compelling alternative to traditional steel due to its superior strength-to-weight ratio, biodegradability, and reduced environmental footprint. This innovative material can significantly lower production costs by eliminating the need for high-energy processes associated with conventional steel manufacturing. For instance, biosteel production requires less energy and generates fewer greenhouse gas emissions, making it an attractive option for industries seeking to reduce their carbon footprint and comply with increasingly stringent environmental regulations. Moreover, the market potential for biosteel is vast and diverse. It can be integrated into various sectors such as construction, automotive, aerospace, and textiles, where its unique properties can enhance performance while reducing material weight. In the construction industry, biosteel could revolutionize building practices by providing stronger yet lighter materials that reduce structural loads and enhance durability. In the automotive sector, it could lead to the development of lighter vehicles with improved fuel efficiency and reduced emissions. The aerospace industry stands to benefit from biosteel's high strength-to-weight ratio, enabling the creation of lighter aircraft components that enhance fuel efficiency and reduce operational costs. Additionally, biosteel's biodegradable nature opens up new avenues in the textile industry. Unlike synthetic fibers that contribute to microplastic pollution, biosteel-based fabrics can decompose naturally at the end of their life cycle, offering a sustainable solution for fashion and apparel. This aligns with the growing consumer demand for eco-friendly products, thereby expanding market opportunities for companies adopting biosteel technology. From an economic standpoint, the adoption of biosteel could stimulate significant economic growth. By reducing production costs and enhancing product performance, companies can achieve higher profit margins while contributing to a more sustainable future. Furthermore, the development of biosteel industries can create new job opportunities in fields such as genetic engineering, biomaterials science, and sustainable manufacturing. This not only boosts local economies but also fosters innovation and technological advancement. In conclusion, the economic advantages and market potential of biosteel are substantial. As the world moves towards a more sustainable future, biosteel stands poised to play a pivotal role in transforming various industries while offering a compelling economic proposition. Its unique blend of superior performance, reduced environmental impact, and cost-effectiveness makes it an indispensable material for the future, underscoring its critical role in the benefits and future of biosteel.
Technological Innovations and Future Developments
Technological innovations are revolutionizing various industries, and one of the most promising areas is the development of biosteel. This cutting-edge material, derived from microbial fermentation processes, offers a sustainable alternative to traditional steel production. Biosteel's future is bright, with several key developments on the horizon that will further enhance its benefits. Firstly, advancements in genetic engineering are expected to improve the efficiency and yield of biosteel production. By optimizing the genetic makeup of microorganisms involved in fermentation, scientists can increase the rate at which these microbes produce the necessary proteins and polymers. This not only reduces production time but also lowers costs, making biosteel more competitive with conventional steel. Secondly, ongoing research in biotechnology is likely to expand the range of applications for biosteel. Currently, biosteel is being explored for use in textiles, medical devices, and even construction materials. Future innovations could see biosteel integrated into advanced composites for aerospace and automotive industries, where its unique combination of strength, lightness, and sustainability would be highly valuable. Moreover, the integration of artificial intelligence (AI) and machine learning (ML) into biosteel production processes promises significant improvements. AI can optimize fermentation conditions in real-time, ensuring optimal protein production and minimizing waste. ML algorithms can predict and prevent potential issues during the manufacturing process, leading to higher quality and consistency in the final product. Sustainability is another critical area where biosteel excels and will continue to evolve. Unlike traditional steel production, which is energy-intensive and generates substantial greenhouse gas emissions, biosteel production is carbon-neutral and uses renewable resources such as biomass. As global efforts to combat climate change intensify, biosteel's eco-friendly profile makes it an increasingly attractive option for industries seeking to reduce their environmental footprint. In addition to these technological advancements, regulatory support and market demand are driving the future of biosteel. Governments worldwide are implementing policies that favor sustainable materials and practices, creating a favorable environment for biosteel to flourish. Consumer awareness about environmental issues is also on the rise, leading companies to seek out sustainable alternatives like biosteel to meet customer expectations. Finally, collaborative research between academia, industry, and government is accelerating the development of biosteel. Joint initiatives are focusing on scaling up production while maintaining quality standards. This collaborative approach ensures that the benefits of biosteel are realized sooner rather than later. In summary, technological innovations are propelling biosteel forward by enhancing its production efficiency, expanding its applications, integrating advanced technologies like AI and ML, improving sustainability, and garnering regulatory and market support. As these developments continue to unfold, biosteel stands poised to become a cornerstone material in various industries, offering a sustainable future that aligns with global environmental goals.