What Is Resistance Measured In
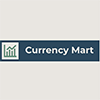
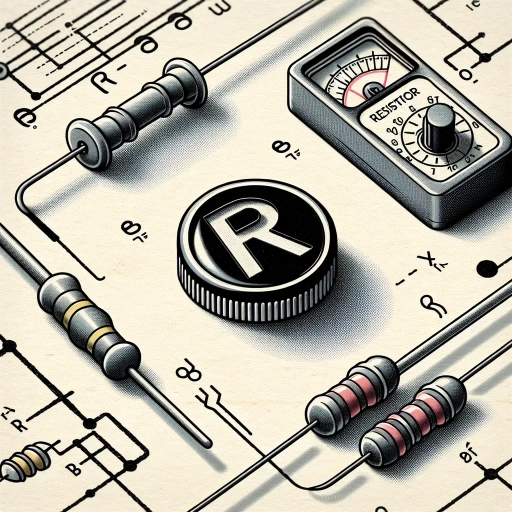
Understanding the Concept of Resistance
In understanding a foundational concept such as resistance, one must first delve into its basic principles, examine its historical development through the years, and discover its significance in electrical engineering. By unearthing these factors, we can comprehend how resistance has evolved and continually influences various aspects of our lives. The power of resistance cannot be underestimated, especially its role in electrical engineering, which revolutionizes our world in uncountable ways. It's pivotal to explore the very roots of its definition and the underlying principles that govern the concept. This broad foundation paves the way to study the historical evolution of resistance measurement and its profound influence on many scientific breakthroughs. Following this journey through time allows us to appreciate even more its undeniable role in electrical engineering. This enlightening path towards understanding sparks from its fundamental definition and principles - a subject that is both intriguing and compelling. Let's venture into the beginning of our journey through the language of resistance, uncovering its Definition and Basic Principles.
Definition and Basic Principles
When discussing resistance, it's integral to first understand its basic definition and fundamental principles. At its core, resistance, often measured in units known as 'Ohms', is the opposition presented to the flow of electric current. Analogous to the way physical obstacles create resistance in water flow, in electrical conductors, such as wires, resistance consists of obstacles that interrupt the free-flow of electrons, which are the carriers of the electric charge. This concept takes root from Ohm's law, one of the fundamental laws in physics. It states that the current passing through a conductor between two points is directly proportional to the voltage across the two points, and inversely proportional to the resistance between them. In other words, resistance is the ratio of voltage difference to the current. It's worth highlighting that resistance always dissipates energy, often in the form of heat, and consequently affects the efficiency of an electrical system. The magnitude of resistance within a conductor depends on several factors. Among these are the type of material, its cross-sectional area, its length, and temperature. Firstly, some materials, known as conductors, such as copper or aluminum, allow electrons to move freely, thereby offering less resistance. In contrast, insulating materials like rubber and ceramics present a high resistance to the flow of electric current. Secondly, the wider or thicker the conductor, the lower the resistance. Conversely, a longer conductor will have a higher resistance. Finally, temperature also has a significant impact on resistance. As temperature increases, so does resistance, due to increased vibration of atoms in the conductor, further impeding the flow of electrons. Understanding these basic principles of resistance is pivotal to several fields such as electronics or electrical engineering. It allows for the prediction and manipulation of current flow in a circuit, the calculation of power loss, and the design of efficient electrical systems. Through continued exploration of the core principles and defining characteristics of resistance, one can steadily gain a more comprehensive understanding of this fundamental concept in physics and its numerous applications.
Historical Development of Resistance Measurement
Although resistance is a concept that we now take as a given in the study of electricity, it took many great minds and advancements over centuries to arrive at the refined understanding we possess today. Research into the nature of resistance dates back to the 18th century, with the works of eminent scholars such as George Ohm and Thomas Edison having particularly notable impacts. The initial defining phase of resistance measurement started with the pioneering work of Georg Simon Ohm in the early 19th century. His research culminated in Ohm’s Law, stating that the current passing through a conductor between two points is directly proportional to the voltage across the two points, introducing resistance as a fundamental concept. He also demonstrated that the resistance of a metal wire is constant regardless of the current, which lent further credibility to the concept in scientific communities. The process of quantifying resistance began to gain momentum by the late 19th century, mainly due to two developments. First, British scientist Sir Charles Wheatstone developed a device that bore his name, the Wheatstone Bridge. This allowed for precise measurements of resistance, by comparing the resistance of an unknown value against known values. Second, the international scientific community agreed upon the standard unit of resistance, the ohm, named in honor of Georg Simon Ohm, cementing his legacy in electrical theory. Into the early 20th century, advances derived from the works of Thomas Edison, particularly his discovery of electric power transmission, played a significant role in further understanding resistance. Edison’s experiments in electricity conduction through different materials forced an understanding that resistance plays a crucial role in determining how much power is lost in transit. Post World War II, with the beginning of the electronics age, resistance took on new facets of importance. Technological breakthroughs and research discovered that not all materials comply with Ohm's Law, enabling the realization of semiconductors, which became essential components of modern electronics. In the contemporary era, the measurement of resistance has become more refined and sophisticated. We now have digital multimeters and LCR meters capable of measuring resistance with a high degree of accuracy. Furthermore, understanding of resistance at the quantum level has opened up exciting possibilities such as superconductivity, and continues to push the boundaries of current knowledge. Consequently, the journey to uncover the true understanding of resistance led manifold researchers through various strides of technological development and scientific insight. Today, the concept of resistance remains as vital to electrical theory as ever, underscored by its historical development.
Importance in Electrical Engineering
Understanding the concept of resistance is paramount in electrical engineering because it profoundly determines how electrical energy transforms and how electronic devices function. Electrical resistance, measured in ohms and symbolized by 'R,' is an essential parameter in Ohm's law. It depicts the opposition or hindrance to the electric current flow throughout a conductor, providing valuable insights into the attributes of the electrical components and systems. Resistance in electrical circuits has a direct bearing on current and voltage. A higher resistance translates to lower current for a given voltage, playing a vital role in filtering the electrical energy passing through components, amplifying signals in electronic devices, and controlling circuit parameters in complex systems. Electrical appliances such as heaters, light bulbs, and toasters leverage resistance to convert electrical energy into heat or light, thereby demonstrating the vital role resistance plays in harnessing the power of electricity. Furthermore, understanding resistance is essential in the design and troubleshooting of circuit-based electronic devices. A higher-than-usual resistance in an electrical circuit could signify a fault like a break in the circuit or degradation of a component, leading to decreased circuit efficiency. Meanwhile, lower resistance is essential for power transmission lines to decrease power losses. In this context, knowing resistance helps devise strategies to enhance efficiency and reliable operation of devices, emphasizing its prominence in electrical engineering. Moreover, the concept of resistance is central to the development of resistive materials and components, such as resistors, and their application in various devices and systems. Having in-depth knowledge of resistance enables engineers to design circuits more efficiently, helping balance loads and prevent overloads. Regulating resistance levels allows safeguarding electrical and electronic devices from potential damage due to excessive current, thus enhancing the longevity of these systems. Finally, resistance measurement provides insights into the quality and safety of electrical installations and equipment. Therefore, electrical engineers employ various tools and techniques to assess resistance, enabling continuous monitoring and control of electrical systems' performance. Understanding resistance ensures that electricity operates as a useful servant rather than a bad master by mitigating safety hazards associated with electricity misuse. In conclusion, resistance plays an essential role in the realm of electrical engineering, from understanding the basic principles of electricity to designing, operating, and safeguarding complex electrical systems. Besides, it helps in developing solutions that transform electrical energy more productively, safely, and efficiently. Hence, the concept of resistance forms an integral part of the foundational knowledge required in electrical engineering.
Units of Measurement for Resistance
underlying the subject of electrical resistance can be completely dissolved once we understand the primary and prevalent units of measurement associated with it. The article aims to deliver a concise yet comprehensive overview of the various units of measurement for resistance, primarily focusing on three supporting pillars - Ohms as the standard unit of resistance, the adoption of subunits and larger units including milliohms and kiloohms for dealing with resistance values at micro or macro levels, and eventually, the fundamental concept of conversion between these units. By sequentially extrapolating these ideas, we shall familiarize ourselves with the relevance and application of each unit, leading to an intrinsic grasp of electrical resistance measurement. As we delve deeper into this significant electrical principle, let's start by elaborating on the standard unit of measurement for resistance, the 'Ohm,' unfolding its integral role in the electrical and electronic world.
Ohms: The Standard Unit of Resistance
The ohm, symbolized with the Greek letter Ω, is the internationally accepted standard unit of electrical resistance. Named after the renowned German physicist Georg Simon Ohm, its value is primarily derived from Ohm's law. This fundamental physics principle emphatically states that the current passing through a conductor, between two points, is directly proportional to the voltage across those two points. Thus, one ohm is defined as the resistance that will allow one ampere of current to pass through when a voltage of one volt is applied. In the broadscenario of practical applications, ohms offer a reliable measure for quantifying resistance in various appliances and electronic devices, including resistors, televisions, radios, and other standard household gadgets. Various ranges of ohm measurements are utilized in different sectors, from high-resistance values in insulation testing, to low readings in telecommunications cable testing. In scientific explorations, the ohm, along with other Binary International (SI) units such as the meter, kilogram, and second, provides an impartial, universal system for global scientific collaboration, fostering consistency and accuracy in experimental results across heterogeneous borders and perspectives. Beyond understanding the ohm as a measure of resistance, many derivatives of the ohm, described on specific ranges of the spectrum, helps further improve measurement accuracy. It includes micro-ohms, used for low resistance measurements in materials like copper cables, megaohms—common in insulation resistance testing, and kilo-ohms, pertinent for gauging resistances in many electronic circuits. Additionally, the ohm isn't just a unit in physics—it is profoundly interwoven into our daily lives. Our world, steeped in technology, thrives on electric power, which invariably involves managing resistance to channel this power efficiently. Whether it is optimizing the sound quality in our headphones or regulating the brightness of our lighting fixtures, managing ohms ultimately leads to progressive hardware and firmware advancements. In essence, the ohm is much more than just a unit of meausrement. It is the cornerstone of technological innovations, shaping advancements from trivial domestic accessories to significant scientific discoveries. Understanding the value of resistance, measured in ohms, is indeed a pivotal step in unraveling the complex, yet fascinating, realm of electricity, where the science of today becomes the technology of tomorrow.
Subunits and Larger Units (Milliohms, Kiloohms, etc.)
The subunits and larger units of Ohm, which is conventionally used to measure resistance, include milliohms, kilohms, and megohms. The resistance of a conductor is determined by the type and amount of material, as well as the temperature and physical dimensions—the longer and thinner the conductor, the greater the resistance. Subunits like milliohms (mΩ) are utilized to measure very small resistances. It is mainly used in the reflective testing of conductive materials or precision circuits because the resistance values are particularly tiny. On the other hand, electricians and technicians mostly use the whole unit (Ohm) as well as the larger units like kilohms (kΩ) and megohms (MΩ). Kiloohms are often used to measure moderate resistances. For example, the resistance of a small light bulb, when measured, falls under the realm of kiloohms. Megohms, being a larger unit, is convenient for measuring high resistance values, such as in insulating materials and some resistive sensors. Minuscule currents are involved when measurements reach up into the megohms, this is often why special tools like high resistance ohmmeters are required. These varying units are all part of the International System of Units (SI) and are used based on the demand of the specific electronic or electrical device under consideration. These different ohmic values can be easily converted between one another, enabling efficient calculation and reading of resistance measurements. It is crucial to choose the appropriate unit of measurement when dealing with resistance to ensure accurate readings and interpretations. Misuse of these units could lead to faulty devices, erroneous scientific data, or, in worst case scenarios, accidents due to electrical malfunction. Therefore, understanding these subunits and larger units is fundamental to any endeavor involving electrical resistance measurement. Efficient management of these measurements contributes to the broader scientific understanding of electronic behavior, culminating in advancements in technology and electronics engineering. From microscopic circuitry components to massive power grids, the resistance units of milliohms, kilohms, and megohms make possible the precise quantification and management of electrical resistance in countless applications worldwide.
Conversion Between Units
The concept of resistance can sometimes seem elusive without the proper units of measurement to give it context. The standard unit for measuring resistance is the ohm, symbolized by the Greek letter omega (Ω). When we dive into the world of physics and electronics, this measure becomes incredibly important, as it helps us understand how much a certain object or material will resist, or oppose, the flow of electric current. However, similar to how we can convert kilometers into miles or pounds into kilos, it is possible to convert between different units of resistance. This process, known as conversion, requires a solid understanding of not only the units themselves but also of the relationships between them. For instance, 1 kilo-ohm (kΩ) is equal to 1000 ohms, and 1 mega-ohm (MΩ) is equivalent to 1 million ohms. Smaller units of resistance include milliohms (mΩ), which are one thousandth of an ohm, and micro-ohms (µΩ), which are one millionth of an ohm. These conversions are important, particularly in the field of electronics, because they enable professionals to speak the same 'language' and understand each other, regardless of their specific scientific background. When you're working with different components of a circuit, for instance, it's important to maintain consistency in the units of measurement being used to provide a straightforward comparison of resistance levels. The conversion between units can be achieved through simple mathematical divisions or multiplications, but in some occasions, the converters available online can make this process easier and more efficient. These tools automatically convert one unit of resistance to another, offering instant results and saving you from potential calculation mistakes. Altogether, the flexibility provided by the conversion between the units of resistance underpins various technological developments and advancements; it is what allows us to design and understand the intricate workings of various electronic devices, from simple circuit boards to complex supercomputers. It's crucial to understand that each unit has its context, for instance, ohms are generally used for smaller appliances, while kilohms or mega-ohms might be referenced when dealing with larger electrical systems. The knowledge of conversion between units of resistance is hence not just crucial for professionals in the field, but also for everyday users who wish to understand the functionality of their devices better, and make informed choices about their use and maintenance. Ultimately, these myriad units and their successful conversion form the backbone of understanding resistance, serving as essential building blocks in our ongoing exploration of the realms of electricity and electronics.
Practical Applications and Measurement Techniques
As we delve into the realm of electronics and engineering, the measurement of resistance becomes an essential aspect to consider. This exploration will not just pique our interest but will also broaden our understanding of this vital component of the technological world. We will first assess the various methods available for measuring resistance, focusing specifically on the utility and methodological details of multimeters and ohmmeters. We will then turn our attention to the practical implications of these techniques in real-world applications in electronics and engineering. This will ensure a holistic appreciation of theoretical approaches coupled with their concrete applications. Pursuing this further, our exploration will also touch upon the common challenges one might face and the key considerations to bear in mind while measuring resistance. While these might seem daunting at times, they provide us valuable insights into the execution of resistance measurement. As we approach the practical procedures, let's introduce ourselves to the fundamentals with the primary method: the use of multimeters and ohmmeters in measuring resistance. Prepared with this knowledge, we can explore, analyze, and appreciate the complexity and intricate aspects of this crucial technical field.
Methods for Measuring Resistance (Multimeters, Ohmmeters)
Methods for measuring electrical resistance are critical in various practical applications such as electronics repair, manufacturing, and research. Two of the most commonly used tools for this purpose are the multimeter and the ohmmeter. Both these devices operate on the principle of Ohm's law which states that the current through a conductor between two points is directly proportional to the voltage applied across the two points, and inversely proportional to the resistance between them. An ohmmeter is a specific type of multimeter that is solely devoted to measuring resistance in ohms (Ω). This device works by running a small amount of current through the component or circuit being measured. By observing the amount of current that is able to pass through, the device is then able to calculate the resistance. It's important to note that ohmmeters must only be used on unpowered components or circuits. Applying power while testing with an ohmmeter can lead to the device getting damaged. On the other hand, a multimeter is a versatile tool that can measure not just resistance, but also voltage (in volts), and current (in amperes). Multimeters come in two varieties: analog and digital. An analog multimeter uses a mechanical needle movement to give a reading, while a digital multimeter provides a digital reading. When measuring resistance, you connect the test probes to two points on the component or circuit. The multimeter then pushes a small known current through, measures the voltage drop that occurs, and calculates the resistance using Ohm's law. While both multimeters and ohmmeters are incredibly useful tools, their measurements are not always 100% accurate. Factors such as internal resistance, contact resistance at the probe points, and even the resistance of the leads themselves can affect readings. Therefore, while these tools provide a good estimate of resistance, for highly precise measurements more sophisticated techniques may be needed. In essence, multimeters and ohmmeters are invaluable instruments in practical applications and measurement techniques involving resistance. Their simplicity, ease of use, and versatility make them highly popular in the fields of electronics and electric engineering. Apart from troubleshooting component and circuit failures, these tools also enable technicians and engineers to perform preventive maintenance and design new electrical systems more efficiently. Therefore, understanding the methods for measuring resistance and the instruments involved is fundamental in our technological world.
Real-World Applications in Electronics and Engineering
Electronics and engineering play a diverse role in multiple real-world applications. As technology advances, these applications become more extensive and intricate, positively impacting various sectors. Integral to these advancements is understanding concepts such as resistance and the units it's measured in - Ohms (Ω), which signifies a vital component in circuit design and analysis. One practical application of ohm law, a principle in electronics, is in the designing and optimization of circuit boards used in several technologies, from computers to smartphones, and even electric vehicles. Engineers must understand the concept of resistance and use Ohm's Law to ensure efficient electric flow and prevent overheating that could potentially result in component damage. Similarly, in traffic engineering, ohm's law is applied in traffic signals to manage resistances in wires to provide optimal lighting. High resistance would mean low current flow, leading to dim lights, which can cause a safety hazard. Accurate resistance measurement ensures bright, efficient lighting, catering for safety needs. In the realm of civil engineering, understanding resistance is essential when working with materials like concrete, particularly when used for constructing buildings or bridges. Ensuring the materials can resist electrical charges can prevent potential accidents and helps to maintain the integrity of the structure. Another quintessential application is in the healthcare sector, where Bioimpedance analysis machines used to measure body composition, such as fat and muscle mass, operate based on principles of resistance. These machines send small electrical currents through the body, measuring the opposition (resistance) met by this current to determine the composition. Even in automotive engineering, resistance plays a crucial role. For instance, fuel sensors in vehicles are essentially potentiometers - devices measuring electrical resistance. They help detect how much fuel is left in the tank, enabling drivers to monitor and refill as necessary. Moreover, the successful operation of several household appliances, like refrigerators, televisions, and ovens, depends on resistance. These appliances contain numerous resistors that control voltage and current flow, ensuring they function correctly without overheating or causing electric shocks. Thus, understanding resistance, its measurement, and application is essential in the effective functioning of these devices. Modern measurement techniques allow for accurate assessments of resistances in various applications. From using multimeters to measure resistance in an electronic circuit to more complex devices employed in industries for large-scale applications, precise readings are crucial. Sophisticated techniques such as Thermostatic Hot Wire Anemometry calculate the resistance of heated wires used in wind tunnels for aerodynamic testing, further exemplifying the importance of resistance measurement. In summary, various sectors, from technology, healthcare, civil, traffic to automotive engineering, apply resistance measurements. These applications necessitate a broad and profound understanding of resistance and the units it's measured in - ohms. Consequently, the grasp of resistance in electronics and engineering is essential for understanding technological advancements, building strong structures, developing efficient transportation networks, improving health assessments, and ensuring effective household devices functionality.
Common Challenges and Considerations in Measuring Resistance
Measuring resistance may seem like a straightforward process; however, it comes with its own set of challenges and considerations. An understanding of these potential difficulties is critical for scientists and engineers working on electrical components' design, operation, and maintenance. One common challenge is the resistance value's fluctuation depending on the external environment. Temperature greatly impacts a material's resistance. As we know, most conductive materials have a positive temperature coefficient. This means as the temperature rises, so does the resistance. Thus, the ambient temperature needs to be accounted for when trying to obtain accurate resistance readings. Another concern is the profound effect of the resistive material's physical properties, such as length, cross-sectional area, and the intrinsic resistivity, contributing to the overall resistance of the component. Only when these variables remain constant, you get consistent measurements of the resistance, which does not happen often in real-life applications. Contact resistance also plays a significant role in measurement accuracy. Incomplete or imperfect electrical connections at the interface between two conductors can lead to additional resistance and inaccurate readings. High contact resistance can lead to significant errors in measurement, causing misunderstandings and faulty calibrations in devices. Furthermore, the apparatus used to measure resistance comes into question as well. Different devices have varying levels of precision, and each has its own limitations. Digital multimeters and ohmmeters, for instance, can yield rapid readings but may not hold up under extremely high resistance values. Wheatstone bridges offer more precision but are more complex to set up and interpret. Therefore, choosing the correct device is another important consideration in resistance measurements. Lastly, the selected technique to measure resistance also impacts the results. Methods like 4-wire resistance measurement can significantly lower errors caused by lead wire resistance, while the voltage-division method suits higher resistance components better. Overall, the considerations in measuring resistance go beyond the basic principles of Ohm's Law. Understanding the various factors complicating resistance measurement is crucial for reliable and accurate results, ensuring the correct interpretation of the data and its subsequent use in practical applications.