What Does Atp Stand For In Text
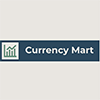
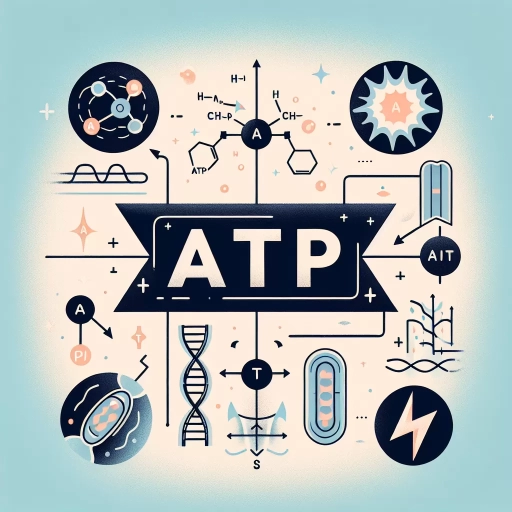
Understanding the Basics of ATP
Introducing the concept of Adenosine Triphosphate (ATP), this far-reaching article embarks on a comprehensive exploration aimed at understanding the basics of this highly significant molecule. ATP, often considered as the molecular currency of intracellular energy transfer, plays a monumental role in nearly all aspects of cell physiology. Our discussion revolves around three pivotal subtopics: Definition and Full Form of ATP, its Historical Context and Discovery, and the Chemical Structure and Composition of ATP. We launch this intriguing journey, soaring from the simple acronyms to the complex nature of ATP's composition, emitting the wealth of knowledge accumulated over years of painstaking research. Our narrative will demystify ATP, guiding you with ease through the labyrinth of advanced biochemistry into the magical world of cellular activities. And so, we head first into our primary discussion point- the Definition and Full Form of ATP, armoring you with the necessary knowledge to appreciate the subsequent understanding of ATP's discovery and chemical composition.
Definition and Full Form
ATP, an abbreviation for Adenosine Triphosphate, is a significant biomolecule that serves as the primary energy currency of the cell. Just like how countries have their currencies that enable various transactions, the cells in our bodies have ATP to process energy-consuming reactions. The full form of ATP, Adenosine Triphosphate, sheds light on its structure. 'Adenosine' refers to the molecule's base consisting of adenine and ribose sugar, while 'Triphosphate' reflects the three phosphate groups attached. Each phosphate group is bonded to the next with high-energy bonds, ready to release energy when required. Understanding the functionality and importance of ATP is pivotal as it plays a central role in the biochemical reactions within cells. Each of the tasks carried out by an organism, from the strokes of a hummingbird's wings to the complex mental processes of a human being, requires ATP. The very existence and operation of life depend significantly on ATP's ability to store and deliver energy in living cells. The ATP molecule stores energy in its phosphate bonds, ready to power various biological processes. The energy contained within ATP is released when the molecule is broken down in a process called hydrolysis - where a water molecule is used to remove a phosphate group from ATP, yielding an ADP (Adenosine Diphosphate) molecule and inorganic phosphate. The removal of this phosphate group releases the energy stored up in the ATP molecule, thus powering the cellular processes which are quite numerous and diverse. In essence, the ATP molecule is like a small, rechargeable battery that powers the machinery of life. When ATP powers a biological process, it gets converted into ADP and inorganic phosphate, ready to be charged again into ATP through cellular respiration, getting equipped with high energy to start the process anew. Though the explanation might seem complex, understanding the ATP's full form and its definition helps to comprehend its primary role as the energy currency of the cell. ATP acts as a liaison between the energy-yielding and energy-consuming reactions in organisms, explicitly maintaining an efficient energy balance for the organism's growth, development, and survival. By providing fuel for essential cellular functions such as protein synthesis, muscle contraction, nerve impulse propagation, and chemical synthesis, ATP justifies its indispensable value in life's existence and continuity.
Historical Context and Discovery
Understanding the Basics of ATP is crucial for unraveling the complex processes of energy transfer within the cell. To fully comprehend this, let's delve into the historical context and discovery of ATP. The knowledge about ATP and its paramount role in energy transfer was not a sudden revelation but a product of numerous contributions from various scientists over many years. Its eventual discovery in the late 1920s marked the beginning of a new era, catapulting the realms of biochemistry and molecular biology forward. The concept of ATP was first put forward by German biochemist Fritz Albert Lipmann, who coined the term 'energy currency' of the cell. In the 1920s, Dr. Cyrus Fiske and Dr. Yellapragada Subbarow initially isolated ATP and identified it as a significant compound in muscle. They found that during chemical reactions in the body, ATP breaks down, releasing energy necessary for the body's function, implying that ATP is the chief molecule for storing and transferring energy in cells. E.P. Kennedy improved upon this information during the 1940s, elucidating that ATP transfers energy via its high-energy phosphate bonds. Later, the pivotal role of ATP in cellular respiration was recognized. When glucose breaks down in cells during glycolysis and oxidative phosphorylation, ATP molecules get charged - this is akin to charging a battery. Subsequently, whenever energy is needed, these ATP molecules discharge, much like batteries powering an appliance. Undoubtedly, the gradual understanding and discovery of ATP has been instrumental, profoundly changing our comprehension of cellular energetics. The elucidation of its structure and function has paved the way for further exploration of many biological phenomena, including muscle contraction, nerve impulse propagation, and chemical synthesis. It's intriguing to recognize that this tiny molecule, ATP, stands as a cornerstone of life, stringing along innumerable biological processes. Grasping the historical underpinnings of its discovery can render a profound appreciation of its essential role in our existence. In essence, ATP is the lifeblood of the cellular world, breathing life into the most fundamental units of living organisms. Today, the ATP molecule stands at the heart of any discourse on cellular energetics, making it impossible to understand life mechanisms without considering ATP's integral role. Conclusively, tracing the origins and development of the discovery of ATP is like reading a riveting novel in the annals of scientific history. Each scientist involved has left behind a legacy of enlightenment, contributing piece by piece to our understanding of this essential molecule. As we continue to deepen our understanding of ATP, we simultaneously heighten our awe at the complexity of life and the powerhouses that are our cells.
Chemical Structure and Composition
Understanding the basic principles of ATP entails a comprehensive grasp of its chemical structure and composition. ATP, or Adenosine Triphosphate, is a complex organic chemical that is found in every cell of every living organism. Its primary purpose in the biological schema is to store and transmit energy, primarily through the breakdown of glucose. The molecule is composed of three main structural components: an adenine ring, a ribose sugar, and three phosphate groups. Each part of the ATP molecule has a critical role in its functionality. The adenine ring, a nitrogenous base, is the building block of DNA and RNA, highlighting the importance of ATP in genetics. The ribose sugar, a five carbon sugar that constitutes a fundamental part of nucleic acids, connects the adenine base to the chain of phosphate groups. The bond between each phosphate group within this chain is known as a high-energy bond. When ATP is hydrolyzed, or broken down, energy is released. This is primarily due to the strong repulsive force among negatively charged phosphate groups, which, once the bond is broken, allows them to move apart, resulting in a release of energy. The energy potential of ATP is primarily contained within the bonds between its phosphate groups. The cleavage of the bond between the second and third phosphate groups is particularly significant— this process, known as hydrolysis, releases a substantial amount of energy. This energy is then largely employed in various biological processes within the organism, such as DNA replication, protein synthesis, muscle contraction, and more. A unique aspect of ATP is its ability to be continually recycled within the organism. After energy has been released through hydrolysis, the ATP molecule is reduced to ADP (Adenosine Diphosphate) and an inorganic phosphate. However, through the process of cellular respiration, especially during the Krebs cycle and oxidative phosphorylation, the energy derived from the breakdown of glucose can be used to reform ATP from ADP. This perpetual cycle of breakdown and reformation characterizes ATP as the primary energy currency within living cells. In conclusion, understanding the unique chemical structure and composition of ATP is essential for any further discussion on its role in living organisms. It is the fundamental energy transfer molecule in the cell, involved in a huge range of biological processes. It is central to life, providing the energy for everything from molecular reactions to muscle contractions, and is constantly being recycled in a system that perfectly encapsulates the efficiency and elegance of biological design.
The Role of ATP in Cellular Processes
Adenosine Triphosphate or ATP plays a pivotal role in a host of cellular processes that underpin the biological functions and life of organisms. This energizing molecule is the cornerstone of three critical sub-topics that underscore its central role in life as we know it. The first is ATP's dynamic involvement in energy transfer and metabolism where it facilitates the change of energy from its packaged form into a workable form - a process critical for the sustenance of all metabolic actions. Second, ATP plays a significant role in cellular respiration and production, which is the process that degrades glucose and other food molecules to produce ATP. Lastly, ATP's role extends to muscle contraction and movement, where its energy fuels the muscle proteins to slide past one another. A closer inspection of these three areas will elucidate why ATP is often called the 'currency of cells.' Let's delve first into ATP's role in energy transfer and metabolism to help us unravel how ATP contributes to life on a molecular level.
Energy Transfer and Metabolism
Energy transfer and metabolism both revolve around the central molecule ATP, short for Adenosine Triphosphate. This form of chemical energy is utilised by cells in various life-sustaining reactions. Its structure comprises a nucleotide, attached to three phosphate groups. When demanding physiological processes occur, ATP transfers its energy by detaching a phosphate group, creating Adenosine Diphosphate (ADP). Energy metabolism primarily includes two kinds of processes - catabolism, where complex molecules are broken down into simpler ones, and anabolism, where these simpler molecules are used to build up complex structures. During catabolism, the energy stored in nutrients like glucose and fats is released and used to reform ATP from ADP. This is called ATP synthesis and mostly occurs in the mitochondria of eukaryotic cells and the cytoplasm of prokaryotic cells. This ATP then provides the energy for various anabolic processes, including protein synthesis, cell growth, and repair. The importance of ATP is highlighted in its versatility- it acts as a source of energy for mechanical work, like muscle contractions during physical activity, and transport work, such as the movement of ions across cell membranes, which is essential in nerve conduction. It also powers chemical work, contributing to the synthesis of complex molecules that cells necessitate for their functions. The process of ATP formation and utilisation can be summarised in the ATP-ADP cycle. As a cell uses ATP for energy, a phosphate molecule is detached, becoming ADP. The process of energy transfer doesn't stop there – ADP is not wasted but undergoes a process called phosphorylation, which combines it with a phosphate to regenerate ATP. So the body manages its energy needs in a very thrifty and efficient way. In conclusion, ATP plays an absolutely critical role in energy transfer and metabolism, serving as the currency for most cellular activities. It is a vital cog that powers all processes of life, from the smallest intracellular activity to the overall functioning of an organism. The dynamic balance between ATP creation and consumption forms the heartbeat of bioenergetics. It clearly illustrates how elegantly and effectively the energy management system functions at the cellular level.
Cellular Respiration and ATP Production
Cellular respiration is a fundamental biological process that is carried out by every cell in the body to generate Adenosine Triphosphate (ATP). ATP, also known as the 'molecular currency of intracellular energy transfer,' is remarkably integral, enabling cells to perform nearly all of their functions, including movement, growth, and division. Cellular respiration combines oxygen and glucose to produce carbon dioxide, water, and ATP – the workhorse that powers almost all the cellular activities. This process comes in three stages: Glycolysis, the Krebs Cycle (also known as the Citric Acid Cycle), and Electron Transport Chain (ETC). During Glycolysis, a small amount of ATP is generated as glucose is split into two molecules of pyruvate, and this occurs in the cytosol of the cell. The Pyruvate molecules then enter the mitochondrion, where the Krebs Cycle happens. In the Krebs Cycle, the Pyruvates are broken down and oxidized, forming two ATP molecules and releasing carbon dioxide as a waste product. Finally, the ETC produces the most significant amount of ATP, about 32-34 molecules. The electrons are transported through a series of proteins and finally combine with molecular oxygen to produce water, and this is where most ATP is made, through a process commonly known as oxidative phosphorylation. Cellular respiration's efficiency is evident in the ability to produce approximately 36 molecules of ATP from one molecule of glucose. This substantial amount of ATP is crucial in driving most of the cell's metabolic processes. It is the biologically available form of energy stored in the chemical bonds of ATP that cells use to perform their functions. The ATP synthesized during cellular respiration serves many roles in the proper functioning of cells. One of the primary roles of ATP is to provide the energy for active transport, which enables the movement of molecules across membranes. ATP also powers cell division, DNA replication, and protein synthesis - all of which are fundamental to an organism's growth and development. Furthermore, ATP is vital in the neural communication process, including transmission of nerve impulses and muscle contraction. In summary, cellular respiration is not just a process by which cells generate water, carbon dioxide, and ATP. It underpins life itself. The ATP produced during this process is the energy currency of the cell, powering many cellular activities. It is a tangible demonstration of the intricate elegance and remarkable efficiency of cellular metabolic processes. Understanding the mechanics of cellular respiration and ATP production is essential to deciphering the intricacies of life at a molecular level. Indeed, the role of ATP in cellular processes is a testament to the intricate dance of life carried out in every cell of every organism, every moment of its life. As such, cellular respiration and ATP production continue to be at the heart of biological research and understanding.
ATP in Muscle Contraction and Movement
Adenosine Triphosphate, commonly referred to as ATP, plays a critical role in muscle contraction and movement - a significant cellular process in our bodies. As a core source of energy, ATP enables the necessary biochemical reactions for these movements, facilitating our ability to control our physical mobility consciously. The process begins when a signal from the nervous system triggers the release of calcium ions into the muscle fibers. The higher concentration of calcium ions prompts the myosin - a motor protein within the muscle fiber - to bind with Actin, another important protein constituting the thin filaments in our muscles. The interaction between myosin and actin causes a ratcheting action, shortening the muscle fiber and resulting in contraction. However, the fundamental catalyst in this process is ATP, without which myosin and actin could not disengage themselves, stalling the contraction process. Essentially, ATP binds to myosin, facilitating the separation of the myosin and actin fibers. Once hydrolized to ADP (Adenosine Diphosphate) and an inorganic phosphate, the ATP provides the myosin with the energy needed to make a power stroke – a change in the myosin head's angle, resulting in the pulling of the actin filament. Moreover, ATP's contribution extends beyond muscle contraction—it is also central to muscle relaxation. After a muscle has contracted - or shortened - it must relax or return to its initial length. For this to occur, the calcium ions within the muscle fibers must be pumped back into an area of the cell known as the sarcoplasmic reticulum. This process also requires energy, which, once again, comes from ATP. When ATP interacts with a pump in the cell membrane, the energy provided by ATP is used to move the calcium ions against their gradient back into the sarcoplic reticulum, inducing relaxation and preparing the muscle for the next contraction. Whether it be raising an arm, blinking an eye, or sprinting down a track, each movement relies on the intricate system of muscle contraction and relaxation - processes heavily reliant on ATP. Without ATP, the continuous cycle of muscle movement would not be possible. And although unseen and often overlooked, ATP lies at the heart of life's most fundamental processes, including muscle contraction and relaxation, making it a truly remarkable molecule.
Applications and Importance of ATP
Adenosine Triphosphate (ATP) is at the heart of life's most fundamental operations. It serves as the primary energy currency of all organisms, ranging from bacteria to humans. Due to its universal and crucial role in biological systems, ATP has been the center of intensive studies across various scientific sectors, as highlighted in this article's three main areas: Biological Significance in Living Organisms, Medical and Pharmaceutical Applications, and Technological Innovations Utilizing ATP. First, we will explore how ATP performs indispensable functions in living entities, contributing vitality to every cell, every moment. It promotes metabolism, contracts muscles, transmits nerve impulses, and fuels immune responses, among many others — a microscopic marvel indeed. Then, we unravel the fascinating world of medical and pharmaceutical industries that bank on ATP, opening possibilities for novel treatments to numerous health conditions. Finally, we dive into the exciting innovations that harness ATP's energy potential in various technological applications. With this, we begin our deep dive into the biological significance of ATP in living organisms, offering us a profound understanding about life's intricate machineries at play, and how ATP serves as its tireless power plant.
Biological Significance in Living Organisms
Biological Significance in Living Organisms plays a key role in explaining the importance and applications of ATP (Adenosine triphosphate). Primarily found in every living cell, ATP serves as the fundamental currency of energy, driving many crucial metabolic processes, thus, its significance is exceptional. ATP molecules provide the impetus required for biochemical reactions, including DNA replication, protein synthesis, muscle contraction, nerve impulse propagation, and transportation of substances across cell membranes; demonstrating an indispensable role in supporting life. The chemical structure of ATP-consisting of an adenosine molecule (adenine plus ribose), and three phosphate groups represents its ability to house potential energy within the chemical bonds of the phosphate groups. The energy stored is then released when the bond between the second and third phosphate group is broken, producing ADP (adenosine diphosphate) and a free phosphate ion, a process known as 'ATP hydrolysis'. The resulting energy is subsequently used to power 'endergonic' reactions- those that require energy. This ATP/ADP cycle, besides being a continuous process, is also extremely swift - a single ATP molecule can be recycled thousands of times within a day. Moreover, the significant role of ATP does not end with being a provider of metabolic energy. It acts as a regulator in signaling pathways, playing crucial roles in transferring signals in intracellular signaling pathways and intricacies of cell communication. A critical example is signal transduction where ATP essentially 'translates' a certain signal inside or outside the cell bringing about a specific cellular response. ATP's relevance also extends to the field of thermoregulation, occupying a central position in regulating body temperature. Through its role in muscle contraction, ATP facilitates shivering, a vital response aimed at generating heat when the body is exposed to colder temperatures, further signifying its importance. The tremendous biological significance of ATP in living organisms cannot be overstated as it underlines life's fundamental processes. Recognizing its omnipresence and multi-faceted roles in cells, it is accurate to term ATP as ‘the molecular unit of currency’ for energy transfer. Accordingly, the sustained study of ATP not only enhances our understanding of life processes but potentially offers avenues in developing strategies for disease treatment where ATP or its pathways are compromised. Regulating ATP production or utilization could be key in managing diseases such as cancer, heart disease, and autoimmune disorders. Thus, ATP underlies broader applications and importance in the realms of biology and medicine, reinstating its fundamental role in human life.
Medical and Pharmaceutical Applications
The diverse range of ATP's applications in the medical and pharmaceutical fields cannot be overstated. This vital molecule plays a pivotal role not just in the cell's energy management but also in various significant biological interactions, becoming an object of significant interest among researchers and physicians. An evident example of ATP’s vital role in medical applications can be seen in chemotherapy for cancer treatment. Drugs used in this therapeutic method often impede tumor growth by targeting and altering the ATP-dependent pathways of cancer cells. This interruption reduces the energy supply to the rapidly-multiplying cells, ultimately hindering their replication process. In conjunction, innovative efforts in pharmaceuticals are currently centered on exploring the concept of ATP-dependent antibiotics as a countermeasure against antibiotic resistance—a global health concern. These antibiotics function by inhibiting ATP-binding transporters, invariably impeding the functionality of harmful bacteria. This rapidly expanding study offers a promising approach to developing new generation drugs to combat a wide array of drug-resistant microbial infections. ATP's application also spills over to cardiovascular medicine, where it serves as a discriminatory tool in heart-attack diagnosis. Upon damage to cardiac muscles, ATP is released into the bloodstream, its heightened levels indicating the severity of damage. This provides physicians with real-time, crucial insights to promptly initiate necessary intervention measures. As the substrate for numerous enzymes and ATP-dependent pumps, ATP’s dysfunction has also been linked to various genetic conditions such as NARP syndrome (neuropathy, ataxia, and retinitis pigmentosa) and Leigh syndrome, offering a ground for manipulative therapies. Therefore, investigations regarding ATP's role in these diseases could open doors to potential curative approaches. Moreover, recent studies indicate that ATP can also be used to improve vaccine efficacy. Scientists are exploring ATP's potential as an adjuvant—a substance that enhances the body's immune response to an antigen. The notion revolves around the pretense that ATP infusion could ‘awake’ dormant immune cells, amplifying the immune response hence allowing vaccines to exert better defense against infectious diseases. Overall, ATP’s exemplary applications in the medical and pharmaceutical realms are a testament to its core influence in biological processes. This knowledge powerfully drives our continuous understanding of human health and encourages the pursuit of revolutionary treatment methods. The state-of-the-art investigations on and around ATP elucidate its diverse, critical roles that go far beyond energy supply, cementing its stature as a molecular lynchpin in the field of medicine and pharmaceuticals.
Technological Innovations Utilizing ATP
Technological innovations that utilize Adenosine Triphosphate (ATP) have revolutionized multiple fields, displaying the profound impact this molecule has beyond the confines of biological organisms. Particularly, the field of nanotechnology has found a novel approach by synthesizing ATP-powered nanomotors. These nanodevices move and function by triggering ATP-mediated chemical reactions, which produce energy for the nanomotors to operate. This mechanism has the potential to revolutionize the medical field as self-propelled nanorobots could be used in targeted drug delivery, minimizing the risk of collateral damages often associated with conventional treatments. Furthermore, by harnessing ATP as a source of power, there lies a potential for engineering renewable energy technologies. This is particularly appealing as it mimics nature's own methods of storing and using energy, thus promising higher sustainability. An additional technology harnessing ATP is biosensors, which measures biological or chemical reactions by generating signals proportional to the concentration of a specific substance in the reaction. Biosensors employing ATP detection find applications in various areas, including food safety, environmental monitoring, and clinical diagnostics. By gauging ATP concentrations, these biosensors can determine the level of microbial contamination in a sample. This provides a rapid, convenient, and sensitive method for detecting even a low level of pathogenic contaminations, ensuring food safety and public health. There is a growing interest also in using ATP in computing technology, engineering bio-computers that use ATP and biochemical reactions to perform computational calculations. Though still in fledgling stages, this technological direction holds promising prospects for enhancing computational power and reducing energy use. This is because bio-computers can possibly process complex and extensive calculations more swiftly than traditional silicon-based computers can, all while consuming less energy, as they use the same molecular computing that living cells employ to rapidly process genetic information. Therefore, ATP, while being fundamentally vital for the survival and functioning of every living cell, has broad, impactful applications that extend into innovative technologies. From nanotechnology to biosensing and even radical approaches to computation, ATP is undeniably an essential molecule that can be exploited for numerous technologies. This clearly elevates the importance of understanding ATP's role and functionalities, not just in a biological context, but also within a broader, technological perspective. The future of ATP utilization in technology is replete with potential for far-reaching advances, reiterating the remarkable versatility of this molecule.