What Is Not Considered A Potential Source Of Carbon Monoxide Gas
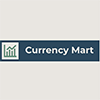
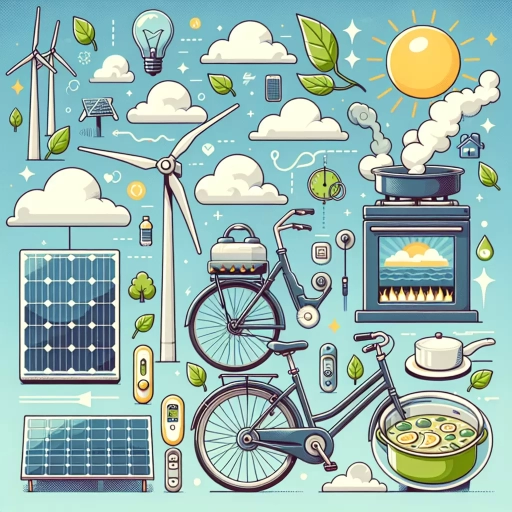
Carbon monoxide (CO) is a colorless, odorless, and highly toxic gas that poses significant health risks when inhaled. While it is commonly associated with combustion processes such as vehicle exhausts and faulty heating systems, there are several sources that are not typically considered potential generators of carbon monoxide. This article delves into three key areas where CO is unlikely to be produced: non-combustion processes, electrical and electronic devices, and natural environments and materials. Understanding these distinctions is crucial for accurately identifying and mitigating CO risks. In non-combustion processes, we explore how certain industrial activities do not involve the burning of fuels and thus do not emit CO. Electrical and electronic devices, by their nature, operate without combustion and are therefore safe from CO emissions. Lastly, natural environments and materials, such as forests and organic substances, do not produce CO under normal conditions. By examining these areas, we can better comprehend the sources that do not contribute to carbon monoxide levels, allowing us to focus on the true culprits. Let's begin by looking at non-combustion processes and how they differ from combustion-based activities in terms of CO production.
Non-Combustion Processes
Non-combustion processes are a diverse and critical set of mechanisms that underpin various natural and industrial phenomena. These processes, which do not involve the burning of materials, are fundamental to understanding several key areas of science and technology. The article delves into three primary categories: Chemical Reactions Not Involving Combustion, Biological Processes, and Geological Activities. Chemical reactions not involving combustion encompass a wide range of transformations that occur without the release of heat and light typical of combustion. These reactions are essential in fields such as pharmaceuticals, materials science, and environmental chemistry. Biological processes, on the other hand, include metabolic pathways, cellular respiration, and photosynthesis, which are vital for life on Earth. These processes ensure the energy and nutrient cycles necessary for sustaining ecosystems. Geological activities, including weathering, erosion, and plate tectonics, shape our planet's surface over millions of years. These non-combustion processes are crucial for understanding Earth's history and predicting future geological events. By exploring these three areas, we gain a comprehensive understanding of how non-combustion processes drive change and sustain life. Let us begin by examining Chemical Reactions Not Involving Combustion, which form the backbone of many industrial and natural chemical transformations.
Chemical Reactions Not Involving Combustion
Chemical reactions not involving combustion are diverse and play crucial roles in various industrial, biological, and environmental processes. These reactions do not involve the oxidation of substances by oxygen, which is a hallmark of combustion reactions. Instead, they encompass a wide range of transformations such as synthesis, decomposition, substitution, and redox reactions that do not release carbon monoxide (CO) as a byproduct. For instance, in the production of ammonia through the Haber-Bosch process, nitrogen and hydrogen gases react under high pressure and temperature in the presence of an iron catalyst to form ammonia without any combustion occurring. This process is vital for the synthesis of fertilizers and other chemicals. Another example is the electrolysis of water, where water molecules are split into hydrogen and oxygen gases using electricity. This reaction is essential for producing hydrogen fuel cells and does not generate CO. In biological systems, cellular respiration involves the breakdown of glucose to produce ATP energy without combustion; instead, it relies on a series of redox reactions within the mitochondria. Similarly, in industrial settings, the production of plastics through polymerization reactions involves the combination of monomers into long chains without any combustion process. These non-combustion chemical reactions are also significant in environmental contexts. For example, the formation of ozone (O3) in the stratosphere occurs through the interaction of ultraviolet radiation with oxygen molecules (O2), a process that protects life on Earth from harmful UV radiation without producing CO. Additionally, photosynthesis in plants involves the conversion of carbon dioxide and water into glucose and oxygen using sunlight, which is another critical non-combustion reaction that supports life on Earth. In contrast to combustion processes that release CO as a harmful byproduct, these non-combustion reactions are generally cleaner and more environmentally friendly. They highlight the versatility and importance of chemical transformations beyond those involving fire or oxidation by oxygen. Understanding these processes is crucial for developing sustainable technologies and ensuring the health and safety of both humans and the environment. Moreover, advancements in catalysts and reaction conditions have enabled the optimization of these non-combustion reactions for higher efficiency and selectivity. This has led to significant improvements in various industries such as pharmaceuticals, where specific chemical transformations can be achieved without generating harmful gases like CO. The study of these reactions also contributes to our broader understanding of chemical kinetics and thermodynamics, allowing scientists to design new materials and processes that minimize environmental impact. In summary, chemical reactions not involving combustion are fundamental to many aspects of modern life, from industrial production to biological processes. They offer cleaner alternatives to combustion-based reactions and are essential for maintaining environmental balance. By focusing on these non-combustion processes, we can develop more sustainable technologies that reduce the risk of carbon monoxide exposure and other environmental hazards associated with combustion.
Biological Processes
Biological processes, unlike combustion processes, do not involve the burning of fuels and therefore are not potential sources of carbon monoxide (CO) gas. These processes are fundamental to life and occur within living organisms, contributing to various essential functions such as metabolism, growth, and reproduction. At the cellular level, biological processes include photosynthesis in plants and certain bacteria, where carbon dioxide and water are converted into glucose and oxygen using sunlight as energy. This process not only produces oxygen but also sequesters carbon dioxide from the atmosphere, making it a crucial component in the global carbon cycle. In animals and humans, biological processes like cellular respiration break down glucose to produce energy in the form of ATP (adenosine triphosphate). While this process involves the breakdown of organic molecules and the release of carbon dioxide as a byproduct, it does not produce carbon monoxide. The absence of high-temperature reactions and incomplete combustion means that CO is not generated during these metabolic pathways. Another significant biological process is fermentation, which occurs in the absence of oxygen and results in the production of ethanol or lactic acid from glucose. This process is vital in certain microorganisms and is also used industrially for the production of alcoholic beverages and biofuels. Despite involving complex biochemical reactions, fermentation does not release carbon monoxide. Additionally, biological processes such as nitrogen fixation by bacteria convert atmospheric nitrogen into forms that can be used by plants, further highlighting the role of these processes in maintaining ecological balance without contributing to CO emissions. In summary, biological processes are essential for life on Earth and play critical roles in energy production, nutrient cycling, and ecosystem health. Unlike combustion processes that can generate harmful gases like carbon monoxide, biological processes operate within the constraints of biochemical pathways that do not produce CO, making them an important aspect of non-combustion processes that do not contribute to carbon monoxide pollution.
Geological Activities
Geological activities, though often overlooked in discussions about carbon monoxide (CO) emissions, play a significant role in understanding the broader context of non-combustion processes. Unlike combustion processes which involve the burning of fossil fuels and are well-known sources of CO, geological activities are natural phenomena that do not involve the combustion of organic materials. These activities include volcanic eruptions, seismic events, and the release of gases from the Earth's crust. During volcanic eruptions, large quantities of gases are released into the atmosphere. While these gases primarily consist of water vapor, sulfur dioxide, and carbon dioxide, they also include trace amounts of other gases such as hydrogen chloride and hydrogen sulfide. However, carbon monoxide is not a significant component of these volcanic emissions. Instead, the primary concern with volcanic gases is their impact on atmospheric chemistry and potential effects on climate and air quality. Seismic activities, such as earthquakes and tremors, can also lead to the release of gases trapped within the Earth's crust. These gases may include methane, radon, and helium but are generally not associated with significant CO emissions. The release of these gases can provide valuable insights into the Earth's internal dynamics and can serve as indicators for seismic activity. Another geological process that involves gas release is the degassing of the Earth's crust. This occurs through various mechanisms including hydrothermal activity and the movement of tectonic plates. While these processes can release a variety of gases, including carbon dioxide and methane, they are not considered potential sources of carbon monoxide. In summary, geological activities such as volcanic eruptions, seismic events, and degassing of the Earth's crust are important non-combustion processes that contribute to our understanding of the Earth's internal dynamics and atmospheric chemistry. However, these processes are not significant sources of carbon monoxide gas. This distinction is crucial when assessing and mitigating CO emissions, as it allows for a more focused approach on identifying and addressing the primary sources of this harmful gas. By understanding the role of geological activities in the Earth's system, we can better differentiate between natural and anthropogenic sources of CO, thereby enhancing our strategies for reducing its impact on human health and the environment.
Electrical and Electronic Devices
In the modern era, electrical and electronic devices have become an integral part of our daily lives, offering a wide range of functionalities that enhance convenience, efficiency, and entertainment. This article delves into the diverse spectrum of these devices, highlighting their unique characteristics and applications. We will explore three key categories: devices without heating elements, battery-powered appliances, and low-voltage electronic components. Each of these categories plays a significant role in shaping our technological landscape. Devices without heating elements, such as fans and air conditioners, are crucial for maintaining comfortable living conditions. Battery-powered appliances, including smartphones and portable tools, offer mobility and independence. Low-voltage electronic components, like microcontrollers and sensors, form the backbone of modern electronics, enabling sophisticated functionalities in various devices. By understanding these different types of electrical and electronic devices, we can better appreciate their contributions to our daily lives. Let us begin by examining devices without heating elements, which are essential for cooling and ventilation systems that keep our homes and workplaces comfortable.
Devices Without Heating Elements
Devices without heating elements are a crucial category within the realm of electrical and electronic devices, particularly when discussing potential sources of carbon monoxide (CO) gas. Carbon monoxide, a colorless, odorless, and highly toxic gas, is often associated with combustion processes involving heating elements. However, devices that operate without these elements are inherently safer in this regard. ### Devices Without Heating Elements Electrical and electronic devices that do not incorporate heating elements are diverse and widespread. These include but are not limited to computers, smartphones, televisions, audio equipment, and various types of sensors and control systems. These devices rely on electrical currents to perform their functions without generating heat through combustion or resistive heating. #### Examples and Applications 1. **Computing Devices**: Computers, laptops, and tablets are prime examples of devices that operate without heating elements. They use semiconductor materials and electronic circuits to process information, store data, and perform tasks without any combustion process. 2. **Communication Devices**: Smartphones, radios, and other communication devices also fall into this category. They utilize electronic circuits and antennas to transmit and receive signals, all while remaining free from heating elements. 3. **Display Devices**: Televisions, monitors, and other display devices use technologies such as LCD (Liquid Crystal Display), OLED (Organic Light-Emitting Diode), or LED (Light Emitting Diode) to display images without generating heat through combustion. 4. **Sensors and Control Systems**: Many industrial and consumer-grade sensors (e.g., temperature sensors, pressure sensors) along with control systems (e.g., programmable logic controllers) operate using electronic signals and do not involve heating elements. #### Safety Considerations The absence of heating elements in these devices significantly reduces the risk of carbon monoxide production. This makes them safer for use in enclosed spaces where ventilation might be limited. For instance, using a computer or smartphone in a small room does not pose the same CO risk as using a gas-powered generator or a fuel-burning appliance. #### Environmental Impact Beyond safety, devices without heating elements often have a lower environmental impact compared to their counterparts that rely on combustion. They typically consume electricity from the grid or batteries, which can be sourced from renewable energy sources like solar or wind power. This aligns with global efforts to reduce greenhouse gas emissions and mitigate climate change. #### Technological Advancements Advancements in technology have further enhanced the efficiency and functionality of these devices. For example, improvements in semiconductor technology have led to more powerful yet energy-efficient computing devices. Similarly, advancements in display technology have resulted in higher resolution displays that consume less power. In conclusion, devices without heating elements play a vital role in modern life by providing essential functionalities while minimizing risks associated with carbon monoxide production. Their widespread use underscores the importance of safe and efficient electrical and electronic engineering practices that prioritize both human safety and environmental sustainability. As technology continues to evolve, it is likely that these devices will become even more prevalent and integral to our daily lives.
Battery-Powered Appliances
Battery-powered appliances are a significant segment within the broader category of electrical and electronic devices, offering numerous advantages that make them an attractive alternative to their traditionally powered counterparts. Unlike devices that rely on direct electrical connections or fuel combustion, battery-powered appliances do not emit carbon monoxide, a colorless, odorless gas that can be lethal in enclosed spaces. This makes them particularly safe for indoor use and ideal for environments where ventilation is limited. One of the primary benefits of battery-powered appliances is their portability and convenience. Devices such as cordless drills, handheld vacuums, and wireless headphones can be used anywhere without the constraint of a power cord. This flexibility is especially valuable in scenarios where mobility is crucial, such as in construction sites or during outdoor activities. Additionally, battery-powered tools often come with rechargeable batteries, reducing the need for frequent battery replacements and thereby minimizing waste. From an environmental perspective, battery-powered appliances can be more eco-friendly compared to gas-powered alternatives. While the production of batteries involves some environmental impact, the overall carbon footprint of battery-powered devices is generally lower when considering the entire lifecycle. Moreover, advancements in battery technology have led to more efficient energy storage and longer battery life, further enhancing their sustainability. In terms of safety, battery-powered appliances eliminate the risk associated with electrical shocks from live wires or sparks from combustion engines. This makes them safer for use around children and pets. Furthermore, many modern battery-powered devices are designed with built-in safety features such as overcharge protection and thermal management systems, which prevent overheating and potential fires. The technology behind battery-powered appliances continues to evolve rapidly. Improvements in lithium-ion batteries have significantly increased their energy density, allowing for more powerful and longer-lasting devices. Innovations in charging technologies, such as fast charging and wireless charging, also enhance user convenience by reducing downtime and making it easier to keep devices powered up. In conclusion, battery-powered appliances represent a safe, convenient, and environmentally friendly option within the realm of electrical and electronic devices. Their absence of carbon monoxide emissions makes them a preferred choice for indoor use, while their portability and safety features add to their appeal. As technology continues to advance, we can expect even more efficient and powerful battery-powered appliances that cater to a wide range of needs without compromising on safety or environmental sustainability.
Low-Voltage Electronic Components
Low-voltage electronic components are crucial elements in the design and operation of modern electrical and electronic devices. These components, which typically operate at voltages below 50 volts, include resistors, capacitors, inductors, diodes, transistors, and integrated circuits. Each type of component plays a specific role in controlling the flow of electrical current and ensuring the proper functioning of electronic systems. **Resistors**, for instance, are used to regulate voltage and current levels within a circuit. They come in various forms such as fixed resistors, variable resistors (potentiometers), and thermistors that change resistance based on temperature. **Capacitors** store electrical energy and are essential for filtering, coupling, and decoupling signals in circuits. They can be classified into different types like ceramic, electrolytic, and film capacitors, each with its own characteristics and applications. **Inductors**, which store energy in a magnetic field when current flows through them, are vital for filtering and resonant circuits. They are often used in power supplies and radio frequency (RF) circuits. **Diodes**, including rectifier diodes and zener diodes, control the direction of current flow and are used for rectification and voltage regulation. **Transistors**, acting as amplifiers or switches, are fundamental in amplifying weak signals and managing power flow in electronic devices. **Integrated Circuits (ICs)** combine multiple components into a single chip of semiconductor material. These ICs can perform complex functions such as microprocessing, memory storage, and signal processing. They are the backbone of modern electronics, enabling the development of compact and efficient devices like smartphones, computers, and medical equipment. The selection and design of low-voltage electronic components must consider factors such as tolerance, temperature stability, and power handling capabilities to ensure reliable operation. Additionally, advancements in technology have led to the development of surface-mount technology (SMT) components that offer higher density and improved performance compared to traditional through-hole components. In summary, low-voltage electronic components are indispensable in the creation of sophisticated electrical and electronic devices. Their precise selection and integration are critical for achieving optimal performance, efficiency, and reliability in a wide range of applications from consumer electronics to industrial control systems. As technology continues to evolve, these components will remain at the forefront of innovation in the field of electrical engineering.
Natural Environments and Materials
Natural environments are intricately woven tapestries of diverse materials and processes, each playing a crucial role in sustaining life and shaping our planet. At the heart of these ecosystems lies the interplay between plant life and photosynthesis, mineral deposits and rocks, and atmospheric gases under normal conditions. Plant life, through the process of photosynthesis, converts sunlight into energy, producing oxygen and organic compounds that support the food chain. This biological activity is deeply connected to the mineral deposits and rocks that form the Earth's crust, providing essential nutrients and habitats for a wide range of species. Meanwhile, atmospheric gases such as nitrogen, oxygen, and carbon dioxide maintain the delicate balance necessary for life to thrive. Understanding these components is vital for appreciating the complexity and beauty of natural environments. By delving into these interconnected elements, we can gain a deeper insight into how they sustain each other and the planet as a whole. Let us begin by exploring the foundational role of plant life and photosynthesis in this intricate web of natural processes.
Plant Life and Photosynthesis
Plant life and photosynthesis are cornerstone components of natural environments, playing a crucial role in the Earth's ecosystem. Photosynthesis, the process by which plants, algae, and some bacteria convert light energy into chemical energy, is fundamental to life on Earth. This intricate process involves the absorption of carbon dioxide (CO₂) from the atmosphere and the release of oxygen (O₂) as a byproduct. This oxygen is essential for the survival of nearly all living organisms, including humans. The mechanism of photosynthesis occurs primarily in the chloroplasts of plant cells, where chlorophyll—a green pigment—absorbs light energy from the sun. This energy is then used to drive a series of chemical reactions that convert CO₂ and water (H₂O) into glucose (a type of sugar) and O₂. The glucose produced serves as a vital source of energy and organic compounds for plant growth and development, while the oxygen released into the atmosphere supports aerobic respiration in other organisms. Beyond its immediate biological significance, photosynthesis has profound implications for Earth's climate and natural environments. By removing CO₂ from the atmosphere, photosynthesis helps regulate the Earth's carbon cycle and mitigate the effects of climate change. Additionally, plant life supports diverse ecosystems by providing habitat, food, and shelter for countless species of animals and microorganisms. Forests, for example, act as carbon sinks, absorbing large amounts of CO₂ and storing it in biomass and soil. In contrast to processes that generate carbon monoxide (CO), such as incomplete combustion of fossil fuels or biomass burning, photosynthesis does not produce CO. Carbon monoxide is a toxic gas that can be harmful to humans and animals at high concentrations. The absence of CO production in photosynthesis underscores its importance as a clean and sustainable biological process that supports life without contributing to air pollution. Furthermore, understanding photosynthesis is crucial for developing sustainable agricultural practices and managing natural resources effectively. By optimizing photosynthetic efficiency through genetic engineering or agronomic techniques, scientists aim to enhance crop yields and improve food security. This knowledge also informs strategies for reforestation and afforestation efforts, which are vital for maintaining healthy ecosystems and combating climate change. In summary, plant life and photosynthesis are essential components of natural environments, driving the Earth's carbon cycle and supporting biodiversity. Unlike processes that generate harmful gases like carbon monoxide, photosynthesis is a clean and vital biological process that underpins life on Earth. Its significance extends beyond biology to impact climate regulation, ecosystem health, and sustainable resource management. As such, it remains a cornerstone of our understanding of natural environments and materials.
Mineral Deposits and Rocks
Mineral deposits and rocks are fundamental components of the Earth's crust, playing a crucial role in shaping natural environments and providing essential materials for human use. These deposits are formed through various geological processes, including magmatic, metamorphic, and sedimentary activities. Magmatic deposits, for instance, arise from the cooling and solidification of magma, resulting in the formation of igneous rocks such as granite and basalt. These rocks can contain valuable minerals like copper, gold, and platinum. Metamorphic deposits are created when existing rocks undergo high pressure and temperature transformations, leading to the formation of minerals like marble and slate. Sedimentary deposits, on the other hand, are formed through the accumulation and compaction of sediments, often containing minerals such as limestone and sandstone. The diversity of mineral deposits is vast, ranging from economic deposits of metals and industrial minerals to non-metallic deposits like coal and oil shale. Economic deposits are those that can be extracted profitably, such as iron ore, copper ore, and gold deposits. These minerals are crucial for various industries, including construction, electronics, and energy production. Non-metallic deposits, while not containing metals, are equally important as they provide resources like coal for energy generation and limestone for cement production. Rocks themselves are classified into three primary types: igneous, sedimentary, and metamorphic. Igneous rocks can be either intrusive (formed below the Earth's surface) or extrusive (formed above the Earth's surface). Sedimentary rocks are formed from the accumulation of sediments and can include organic materials like fossils. Metamorphic rocks result from the alteration of existing rocks under high pressure and temperature conditions. Understanding mineral deposits and rocks is essential for environmental conservation and sustainable resource management. For example, the extraction of mineral resources must be balanced with environmental considerations to avoid pollution and habitat destruction. Additionally, the study of rocks helps in understanding geological hazards such as earthquakes and volcanic eruptions, which are critical for disaster preparedness and mitigation. In the context of natural environments and materials, mineral deposits and rocks also play a significant role in shaping landscapes and ecosystems. For instance, the composition of rocks influences soil formation, which in turn affects plant growth and biodiversity. Furthermore, the presence of certain minerals can impact water quality by affecting pH levels or introducing harmful substances. However, it is important to note that mineral deposits and rocks are not considered potential sources of carbon monoxide gas. Carbon monoxide is primarily produced through incomplete combustion of carbon-containing fuels such as gasoline, natural gas, and wood. It can also be generated in industrial processes involving the reduction of metal oxides. Therefore, while mineral deposits and rocks are vital for various aspects of human life and natural environments, they do not contribute to the production of carbon monoxide gas. This distinction highlights the importance of understanding different sources of environmental pollutants to effectively manage and mitigate their impacts.
Atmospheric Gases Under Normal Conditions
Atmospheric gases under normal conditions are a crucial component of the Earth's natural environment, playing a vital role in sustaining life and regulating climate. The primary gases that make up the Earth's atmosphere include nitrogen (N₂), oxygen (O₂), argon (Ar), carbon dioxide (CO₂), and trace amounts of other gases such as neon (Ne), helium (He), methane (CH₄), and water vapor (H₂O). Under normal conditions, these gases exist in a delicate balance that supports the Earth's ecosystems. Nitrogen and oxygen, which together account for about 99% of the atmosphere, are essential for plant growth and animal respiration. Carbon dioxide, though present in much smaller quantities, is critical for photosynthesis and has significant implications for global climate due to its role as a greenhouse gas. Water vapor, varying in concentration depending on temperature and humidity, influences weather patterns and helps regulate Earth's temperature through its role in the water cycle. Other trace gases like methane and nitrous oxide also contribute to the greenhouse effect, albeit in smaller measures. However, it is important to note that under normal atmospheric conditions, none of these gases are considered potential sources of carbon monoxide (CO). Carbon monoxide is a highly toxic gas produced primarily through incomplete combustion of carbon-containing fuels such as wood, gasoline, and natural gas. It is not a naturally occurring component of the Earth's atmosphere under normal conditions but rather an anthropogenic pollutant resulting from human activities like industrial processes, vehicle emissions, and improper use of fuel-burning appliances. Understanding the composition and behavior of atmospheric gases is essential for managing natural environments and materials sustainably. For instance, monitoring changes in CO₂ levels helps scientists track global warming trends and develop strategies to mitigate climate change. Similarly, managing water vapor levels through irrigation practices can enhance agricultural productivity while minimizing environmental impact. The balance of these gases also affects the durability and performance of materials used in construction and manufacturing; for example, high humidity can accelerate corrosion in metals or degrade certain types of building materials. In summary, atmospheric gases under normal conditions form a complex yet balanced system that supports life on Earth while influencing climate and weather patterns. Recognizing which gases are naturally present and which are not—such as carbon monoxide—helps us better manage our natural environments and materials sustainably, ensuring a healthier planet for future generations. This understanding is pivotal in the broader context of natural environments and materials, guiding us towards more informed decisions in environmental conservation and resource management.