What Does Rpm Mean
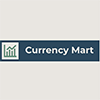
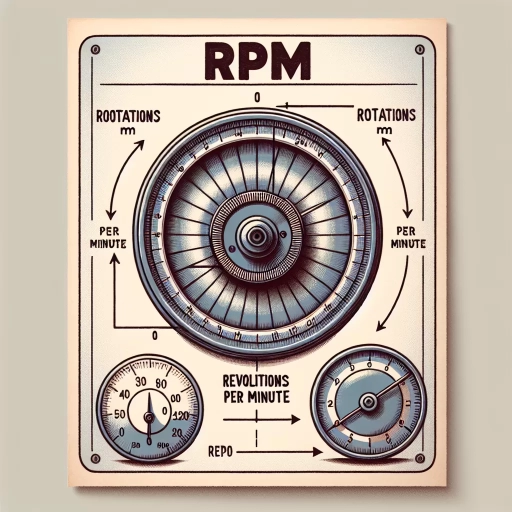
In the realm of mechanics and engineering, few terms are as ubiquitous yet misunderstood as "RPM," or revolutions per minute. This metric, which measures the rotational speed of a shaft or other rotating component, is crucial in various fields, from automotive and aerospace to industrial machinery and consumer electronics. Understanding RPM is not just about grasping a simple definition; it involves delving into its technical aspects, practical applications, and broader implications. This article will explore the concept of RPM in depth, starting with the basics of what RPM is and how it is calculated. We will then delve into the technical aspects that make RPM a vital parameter in engineering and design. Finally, we will examine the practical uses and implications of RPM in real-world scenarios. By the end of this journey, readers will have a comprehensive understanding of RPM, beginning with **Understanding the Basics of RPM**.
Understanding the Basics of RPM
Understanding the basics of RPM (Revolutions Per Minute) is crucial for grasping various mechanical and technological concepts. RPM is a fundamental measure that quantifies the rotational speed of an object, such as a wheel, gear, or engine. To delve into the intricacies of RPM, it is essential to explore three key aspects: its definition and origin, measurement units and contexts, and common applications in everyday life. By defining what RPM is and tracing its origins, we can establish a solid foundation for understanding its significance. This knowledge will then be expanded upon by examining the different measurement units and contexts in which RPM is used, highlighting its versatility across various fields. Finally, exploring common applications in everyday life will illustrate how RPM impacts our daily interactions with technology and machinery. Let us begin by uncovering the definition and origin of RPM, setting the stage for a comprehensive exploration of this vital metric.
Definition and Origin
**Understanding the Basics of RPM: Definition and Origin** RPM, or revolutions per minute, is a fundamental metric used to measure the rotational speed of an object, particularly in machinery and automotive contexts. At its core, RPM quantifies how many complete rotations an object makes around its axis within a single minute. This measurement is crucial for assessing performance, efficiency, and operational health in various mechanical systems. The origin of RPM can be traced back to the early days of industrialization when precise measurement of rotational speeds became essential for optimizing machinery performance. As factories began to adopt more complex machinery during the Industrial Revolution, engineers needed a standardized way to gauge and compare the speeds of different machines. The term "revolutions per minute" emerged as a straightforward and intuitive unit that could be easily understood and applied across different industries. In modern times, RPM is used in a wide range of applications. For instance, in automotive engineering, RPM is a key indicator of an engine's performance and efficiency. Car manufacturers often specify optimal RPM ranges for different driving conditions to ensure fuel efficiency and engine longevity. Similarly, in industrial settings, RPM is critical for maintaining the optimal operation of machinery such as pumps, generators, and motors. The definition of RPM is straightforward yet versatile. It is calculated by counting the number of complete rotations an object makes in one minute. This can be done using various methods, including mechanical counters, electronic sensors, and even software tools. For example, a car's tachometer displays the engine's RPM in real-time, allowing drivers to adjust their driving habits accordingly. Understanding RPM is not just about knowing its definition; it also involves recognizing its practical implications. For instance, running machinery at incorrect RPMs can lead to inefficiency, increased wear and tear, and even safety hazards. Conversely, operating within the recommended RPM range ensures that machinery runs smoothly, efficiently, and safely. In summary, RPM is a vital metric that has evolved from a simple measurement tool during the Industrial Revolution to a critical component in modern engineering and technology. Its widespread use underscores its importance in ensuring optimal performance across various mechanical systems. By grasping the definition and origin of RPM, individuals can better appreciate its role in maintaining efficiency and safety in both everyday life and industrial operations. This foundational understanding sets the stage for deeper exploration into the intricacies of RPM and its applications in different fields.
Measurement Units and Contexts
Understanding the basics of RPM (Revolutions Per Minute) necessitates a solid grasp of measurement units and their contexts. Measurement units are fundamental in quantifying physical quantities, and they vary widely depending on the context in which they are used. For instance, in mechanics, units such as meters for length, kilograms for mass, and seconds for time are standard. However, when discussing rotational motion, RPM becomes a critical unit because it measures the number of complete rotations a shaft or wheel makes in one minute. To appreciate RPM fully, it's essential to understand its relationship with other measurement units. For example, angular velocity is often measured in radians per second (rad/s), but converting this to RPM provides a more intuitive understanding of rotational speed. The conversion involves multiplying the angular velocity by 60 seconds (to convert from seconds to minutes) and dividing by \(2\pi\) radians (to convert from radians to revolutions). This conversion highlights how different units can represent the same physical quantity but offer different insights depending on the context. In various fields, different measurement units are preferred due to their relevance and ease of interpretation. In engineering and manufacturing, RPM is crucial for specifying motor speeds, gear ratios, and other mechanical performance metrics. In automotive contexts, RPM gauges are standard on dashboards to help drivers monitor engine performance and optimize fuel efficiency. In medical devices like centrifuges or dental drills, precise control over RPM ensures safe and effective operation. Moreover, understanding measurement units in different contexts helps in making accurate comparisons and calculations. For instance, comparing the RPM of a car engine with that of a helicopter rotor requires considering the specific application and environment. The same RPM value can have vastly different implications depending on factors such as load, torque, and intended use. In scientific research and experimentation, precise measurement units are vital for reproducibility and accuracy. Researchers often use standardized units to ensure that results are comparable across different studies and experiments. This consistency allows for meaningful analysis and interpretation of data, which is particularly important when studying phenomena involving rotational motion. In conclusion, mastering measurement units and their contexts is indispensable for comprehending RPM and its applications. By recognizing how different units relate to each other and understanding their specific uses in various fields, one can gain a deeper appreciation for the intricacies of rotational motion and its measurement. This foundational knowledge not only enhances technical skills but also fosters better decision-making and problem-solving in both practical and theoretical scenarios involving RPM.
Common Applications in Everyday Life
Understanding the basics of RPM (Revolutions Per Minute) is crucial for grasping its widespread applications in everyday life. RPM is a measure of the rotational speed of an object, typically expressed in revolutions per minute. This metric is fundamental across various industries and daily activities, making it an integral part of our lives. In **automotive engineering**, RPM is a critical parameter for vehicle performance. Car engines operate within specific RPM ranges to optimize power output, fuel efficiency, and engine longevity. For instance, shifting gears in a manual transmission vehicle involves adjusting the RPM to match the driving conditions, ensuring smooth acceleration and preventing engine strain. Similarly, in **aviation**, aircraft engines are monitored closely for their RPM to ensure safe takeoff, cruising, and landing. **Industrial machinery** also relies heavily on RPM. Manufacturing processes such as milling, drilling, and grinding require precise control over the rotational speed of tools to achieve desired outcomes without damaging materials or equipment. In **medical devices**, RPM is essential for the operation of equipment like centrifuges used in laboratories to separate blood components or other biological samples. In **consumer electronics**, fans and motors operate at specific RPMs to maintain optimal cooling and efficiency. For example, computer fans and air conditioners use RPM to regulate airflow and temperature control. Even **household appliances** like washing machines and blenders utilize RPM to perform their functions effectively. **Fitness and sports** also incorporate RPM measurements. Stationary bicycles and elliptical machines display RPM to help users monitor their workout intensity and progress. Cyclists use cadence meters to track their pedaling speed in revolutions per minute, which aids in optimizing performance and reducing fatigue. Moreover, **music and audio equipment** often involve RPM considerations. Turntables for vinyl records rotate at precise speeds (typically 33 1/3 or 45 RPM) to ensure accurate playback of music. Similarly, in **video production**, cameras may use RPM to control the speed of film reels or digital recording mechanisms. In **agriculture**, tractors and other farm machinery rely on RPM to manage tasks such as plowing, harvesting, and irrigation efficiently. The correct RPM ensures that these machines operate at optimal levels without wasting fuel or causing mechanical stress. Lastly, **scientific research** frequently employs RPM in various experiments. Centrifuges in laboratories spin at high RPMs to separate substances based on density, while spectrometers may use rotating components at specific speeds to analyze samples accurately. In conclusion, understanding RPM is not just about grasping a technical term; it's about recognizing its pervasive influence across multiple facets of modern life. From the engines of our cars to the fans in our computers, from industrial machinery to medical devices, RPM plays a vital role in ensuring efficiency, safety, and performance. This fundamental concept underscores the interconnectedness of technology and everyday life, highlighting how a simple measure can have far-reaching implications.
Technical Aspects of RPM
Understanding the technical aspects of RPM (Revolutions Per Minute) is crucial for comprehending the intricate mechanics of engines and machinery. RPM is a fundamental metric that influences various aspects of engine performance and efficiency. This article delves into three key areas: how RPM is calculated and measured, its relationship with other mechanical metrics, and its importance in engine performance and efficiency. Firstly, grasping how RPM is calculated and measured provides a foundational understanding of the metric itself. This involves understanding the principles behind tachometers and other measurement tools, as well as the mathematical formulas used to derive RPM from rotational speed data. Secondly, exploring the relationship between RPM and other mechanical metrics such as torque, horsepower, and gear ratios reveals how these interrelated components collectively impact overall system performance. This relationship is vital for optimizing engine design and ensuring optimal operation under various conditions. Lastly, the importance of RPM in engine performance and efficiency cannot be overstated. It directly affects fuel consumption, power output, and the longevity of engine components. By understanding these dynamics, engineers and technicians can make informed decisions to enhance engine reliability and efficiency. Transitioning to the first supporting paragraph, we will examine **How RPM is Calculated and Measured**, providing a detailed look into the methods and tools used to accurately determine this critical metric.
How RPM is Calculated and Measured
**How RPM is Calculated and Measured** Calculating and measuring revolutions per minute (RPM) is a fundamental aspect of understanding the operational efficiency and performance of rotating machinery, such as engines, motors, and gears. RPM is essentially a measure of how many times a shaft or wheel completes a full rotation in one minute. To calculate RPM, you typically need to know the rotational speed in revolutions per second (RPS) and then convert it to revolutions per minute. This can be done using the simple formula: RPM = RPS * 60. For instance, if a motor completes 10 revolutions in one second, its RPM would be 10 RPS * 60 = 600 RPM. In practical applications, RPM is often measured using various types of sensors and instruments. One common method involves using a tachometer, which can be mechanical or digital. Mechanical tachometers typically use a dial and needle to display the RPM reading, while digital tachometers provide more precise readings through electronic displays. Another method for measuring RPM involves the use of optical or magnetic sensors. Optical tachometers work by detecting the passage of a reflective mark on the rotating shaft as it passes through a light beam, converting this into an electrical signal that is then processed to display the RPM. Magnetic tachometers, on the other hand, use a magnet attached to the rotating shaft and a sensor coil to detect changes in magnetic field strength as the shaft rotates. In modern industrial settings, advanced technologies like laser Doppler velocimetry (LDV) and encoder-based systems are also employed for precise RPM measurement. LDV measures the frequency shift of laser light reflected from moving surfaces, allowing for highly accurate measurements even at very high speeds. Encoder-based systems use digital encoders that generate pulses as the shaft rotates; these pulses are then counted and processed to determine the exact RPM. The accuracy of RPM measurement is crucial in various industries such as automotive, aerospace, and manufacturing where even slight deviations can impact performance, efficiency, and safety. For example, in automotive engineering, precise control over engine RPM is essential for optimizing fuel efficiency and reducing emissions. Similarly, in manufacturing processes involving high-speed machinery, accurate RPM measurements help maintain product quality and prevent equipment damage. In summary, calculating and measuring RPM involves understanding basic mathematical conversions and utilizing appropriate measurement tools ranging from simple tachometers to advanced technologies like LDV and digital encoders. The precision of these measurements directly impacts the efficiency, safety, and overall performance of rotating machinery across diverse industrial applications.
Relationship Between RPM and Other Mechanical Metrics
The relationship between RPM (Revolutions Per Minute) and other mechanical metrics is fundamental to understanding the performance and efficiency of various mechanical systems. RPM, a measure of rotational speed, is intricately linked with other key metrics such as torque, power, and efficiency. **Torque**, for instance, is the rotational force that causes an object to rotate. The relationship between RPM and torque is inversely proportional in many applications; as RPM increases, torque typically decreases, and vice versa. This is evident in engines where high RPMs often result in lower torque but higher power output due to the increased number of revolutions. **Power**, another critical metric, is directly related to RPM. Power output is calculated as the product of torque and angular velocity (which is proportional to RPM). Therefore, an increase in RPM can lead to an increase in power output if the torque remains constant or does not decrease significantly. This principle is crucial in designing engines and gearboxes where optimizing power output at different RPM ranges is essential for performance. **Efficiency** also plays a significant role in the context of RPM. Mechanical efficiency is often maximized at specific RPM ranges where frictional losses are minimized and the system operates within its optimal design parameters. For example, in internal combustion engines, there is typically an optimal RPM range where fuel efficiency and power output are balanced. Operating outside this range can lead to reduced efficiency and increased wear on the engine. Additionally, **gear ratios** are closely tied to RPM in mechanical systems that use gearing to adjust speed and torque. Gear ratios allow for the adjustment of RPM to suit different operating conditions. For instance, in vehicles, lower gear ratios are used for starting from a standstill or climbing steep inclines (where high torque is needed), while higher gear ratios are used for cruising at high speeds (where lower torque but higher RPMs are beneficial). Furthermore, **vibration** and **noise** levels are also influenced by RPM. Higher RPMs can lead to increased vibration and noise due to the increased frequency of rotational cycles. This is particularly relevant in machinery design where balancing high performance with low vibration and noise levels is a key challenge. In **industrial applications**, such as pumps and compressors, the relationship between RPM and flow rate or pressure is critical. For example, in centrifugal pumps, the flow rate is directly proportional to the RPM, while the pressure head is proportional to the square of the RPM. Understanding these relationships is essential for selecting the right equipment for specific tasks. In summary, the relationship between RPM and other mechanical metrics such as torque, power, efficiency, gear ratios, vibration, and noise levels is complex and multifaceted. Understanding these interdependencies is crucial for designing, optimizing, and operating mechanical systems efficiently and effectively. By leveraging these relationships, engineers can create systems that balance performance, efficiency, and durability across a wide range of applications.
Importance in Engine Performance and Efficiency
The importance of engine performance and efficiency cannot be overstated, particularly when considering the technical aspects of RPM (Revolutions Per Minute). Engine performance is a critical factor in determining the overall functionality and reliability of a vehicle or machinery. It encompasses various parameters such as power output, torque, and fuel efficiency, all of which are intricately linked to RPM. At its core, RPM measures how many times the engine's crankshaft rotates in one minute, which directly influences the engine's ability to generate power and torque. Higher RPMs typically result in increased power output but may also lead to higher fuel consumption and increased wear on engine components. Conversely, lower RPMs can enhance fuel efficiency but may compromise on power delivery, especially during demanding driving conditions. Efficiency in engine performance is equally crucial as it affects both the economic and environmental impact of the vehicle. Modern engines are designed with advanced technologies such as variable valve timing, direct fuel injection, and turbocharging to optimize performance across a wide range of RPMs. These technologies allow engines to achieve better fuel economy without sacrificing significant power output. Moreover, the relationship between RPM and engine efficiency is complex and multifaceted. For instance, operating an engine within its optimal RPM range can significantly reduce emissions and improve overall engine longevity. This is because engines are typically tuned to run most efficiently within specific RPM bands where combustion is most complete and stress on components is minimized. In addition to these technical considerations, driver behavior also plays a pivotal role in maximizing engine performance and efficiency. Drivers who maintain a steady speed, avoid sudden accelerations, and use appropriate gear shifts can help the engine operate within its optimal RPM range more consistently. This not only enhances fuel efficiency but also reduces wear and tear on the engine. From a maintenance perspective, monitoring and maintaining optimal RPM levels is essential for extending the lifespan of the engine. Regular tune-ups, oil changes, and ensuring proper air-fuel mixture all contribute to keeping the engine running smoothly across various RPM ranges. In conclusion, understanding the importance of engine performance and efficiency in relation to RPM is vital for both vehicle owners and engineers. By optimizing engine operation within its optimal RPM range, one can achieve a balance between power delivery and fuel economy while ensuring the longevity of the engine. This synergy underscores the critical role that RPM plays in the broader context of technical aspects related to engine performance and efficiency.
Practical Uses and Implications of RPM
The Practical Uses and Implications of RPM (Revolutions Per Minute) are multifaceted and far-reaching, impacting various sectors with significant consequences. In the realm of automotive and industrial settings, RPM plays a crucial role in optimizing performance and efficiency. This article delves into three key areas: the application of RPM in automotive and industrial environments, its impact on fuel efficiency and vehicle maintenance, and the critical safety considerations and operational guidelines associated with it. By understanding how RPM influences these aspects, we can better appreciate its importance in modern machinery. For instance, in automotive settings, RPM directly affects engine performance, fuel consumption, and overall vehicle longevity. Similarly, in industrial contexts, precise control over RPM is essential for maintaining equipment health and ensuring operational safety. As we explore these dimensions, it becomes clear that RPM is not just a technical metric but a vital component of efficient and safe operation. Let us begin by examining the role of RPM in automotive and industrial settings, where its practical applications are most evident.
RPM in Automotive and Industrial Settings
In both automotive and industrial settings, RPM (Revolutions Per Minute) is a critical metric that measures the rotational speed of engines, motors, and other machinery. This measurement is essential for ensuring optimal performance, efficiency, and longevity of equipment. In automotive contexts, RPM gauges are standard on vehicle dashboards, providing drivers with real-time feedback on engine speed. This information helps drivers manage gear shifts to avoid over-revving or under-revving the engine, which can lead to reduced fuel efficiency, increased wear on components, and potential engine damage. For instance, shifting gears at the right RPM range can improve acceleration and reduce fuel consumption. In industrial settings, RPM monitoring is equally crucial. Industrial machinery such as pumps, compressors, and conveyor belts operate within specific RPM ranges to maintain efficiency and prevent overheating or mechanical failure. For example, a centrifugal pump must operate within its designated RPM range to ensure it delivers the required flow rate without excessive vibration or energy consumption. Similarly, in manufacturing processes, precise control over RPM is necessary for tasks like cutting, drilling, and grinding to achieve consistent quality and avoid tool wear. Moreover, understanding RPM is vital for predictive maintenance strategies. By monitoring changes in RPM over time, operators can detect early signs of mechanical issues such as bearing wear or misalignment. This proactive approach allows for scheduled maintenance rather than emergency repairs, reducing downtime and operational costs. Additionally, advancements in technology have enabled the integration of IoT sensors and data analytics to monitor RPM in real-time, providing insights into equipment health and performance trends. The implications of RPM extend beyond operational efficiency; they also impact safety. In high-speed applications like power generation or aerospace engineering, deviations from optimal RPM can lead to catastrophic failures. Therefore, strict adherence to specified RPM ranges is mandated by regulatory standards to ensure public safety and prevent accidents. In summary, RPM is a fundamental parameter in both automotive and industrial environments. It influences performance, efficiency, maintenance schedules, and safety protocols. By understanding and effectively managing RPM, operators can optimize their equipment's lifespan, reduce operational costs, and enhance overall productivity. As technology continues to evolve with more sophisticated monitoring systems and data analytics tools, the practical uses and implications of RPM will only become more pronounced and critical in various sectors.
Impact on Fuel Efficiency and Vehicle Maintenance
The impact of RPM (Revolutions Per Minute) on fuel efficiency and vehicle maintenance is a critical aspect to consider for any vehicle owner. Understanding how RPM affects your car's performance can significantly influence your driving habits, maintenance routines, and overall cost of ownership. When it comes to fuel efficiency, RPM plays a pivotal role. Operating your vehicle within the optimal RPM range can maximize fuel economy. For instance, driving at lower RPMs (typically between 1,500 to 2,500 RPM) during city driving or when cruising on the highway can help reduce fuel consumption. This is because engines are generally more efficient at lower RPMs, where they produce sufficient power without over-revving. Conversely, high RPMs (above 3,000 RPM) are usually reserved for situations requiring rapid acceleration or climbing steep inclines, as they consume more fuel and increase wear on engine components. Moreover, maintaining optimal RPM levels is crucial for vehicle maintenance. Consistently running your engine at high RPMs can lead to increased wear on engine parts such as pistons, valves, and bearings. This accelerated wear can result in premature engine failure and higher maintenance costs over time. Additionally, frequent high-RPM driving can also affect other components like the transmission and drivetrain, leading to potential issues such as overheating or reduced lifespan. On the other hand, adhering to recommended RPM ranges helps in prolonging the life of your vehicle's engine and other critical components. For example, avoiding extreme RPM fluctuations during normal driving conditions reduces stress on the engine block and cylinder head, thereby minimizing the risk of cracks or other structural damages. Furthermore, maintaining a steady RPM during routine driving helps in ensuring that the engine oil circulates efficiently throughout the system, which is essential for lubricating moving parts and preventing overheating. In practical terms, drivers can implement several strategies to optimize their vehicle's performance based on RPM considerations. One effective method is to use the correct gear for the speed you are traveling at; this ensures that the engine operates within its optimal RPM range. Another approach is to avoid sudden accelerations and hard braking, which can cause unnecessary strain on the engine and other components. Regular tune-ups and oil changes are also essential in maintaining engine health and ensuring that it runs smoothly within its recommended RPM parameters. In conclusion, understanding the impact of RPM on fuel efficiency and vehicle maintenance is vital for any driver seeking to maximize their car's performance while minimizing costs. By operating within optimal RPM ranges and adopting mindful driving habits, drivers can significantly enhance their fuel economy, reduce wear on critical components, and extend the overall lifespan of their vehicle. This knowledge not only contributes to better driving practices but also underscores the practical implications of RPM in everyday vehicle use.
Safety Considerations and Operational Guidelines
When delving into the practical uses and implications of RPM (Revolutions Per Minute), it is crucial to emphasize the importance of safety considerations and operational guidelines. RPM, a measure of rotational speed, is pivotal in various industrial, automotive, and mechanical applications. However, the high-speed nature of these operations necessitates stringent safety protocols to prevent accidents and ensure optimal performance. Firstly, **personal protective equipment (PPE)** is paramount. Operators should always wear appropriate gear such as gloves, safety glasses, and ear protection to mitigate risks associated with flying debris or loud machinery. Additionally, **training and education** are essential; personnel must be thoroughly instructed on the proper use of equipment, understanding the significance of RPM in their specific tasks. This includes recognizing warning signs of malfunctioning machinery and knowing emergency shutdown procedures. **Regular maintenance** is another critical aspect. Equipment should be inspected frequently for wear and tear, with particular attention to components that could fail at high RPMs. Lubrication systems must be checked regularly to prevent overheating and mechanical failure. Moreover, **operational guidelines** should be strictly adhered to; this includes following manufacturer recommendations for maximum RPM limits and ensuring that all safety features are functioning correctly. In industrial settings, **environmental factors** also play a significant role. For instance, working in confined spaces or areas with poor ventilation can increase the risk of accidents due to reduced visibility or inhalation of harmful fumes. Therefore, ensuring good ventilation and using appropriate lighting can significantly enhance safety. Furthermore, **electrical safety** is a key consideration when dealing with high-RPM machinery. Proper grounding and insulation of electrical components are vital to prevent electrical shocks or fires. The use of **safety interlocks** on machinery ensures that it cannot be started unless all guards are in place, protecting operators from moving parts. In automotive contexts, understanding RPM is crucial for both performance and safety. Drivers need to be aware of their vehicle's optimal RPM range to avoid engine damage while also ensuring safe driving practices such as not over-revving in low gear or ignoring warning signs like unusual vibrations. Lastly, **emergency preparedness** is indispensable. Having a well-rehearsed emergency response plan in place can significantly reduce the impact of any potential incident. This includes knowing first aid procedures for common injuries related to high-speed machinery and having access to emergency shutdown mechanisms. By adhering to these safety considerations and operational guidelines, individuals can maximize the benefits of RPM while minimizing risks. This holistic approach not only ensures the longevity of equipment but also safeguards human life, making it an integral part of any operation involving rotational speed measurements.