What Humans Can See That Whales And Sharks Cannot
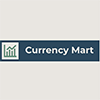
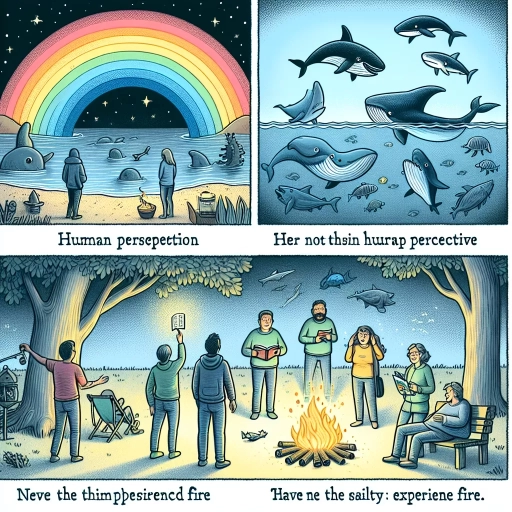
Humans and marine creatures like whales and sharks inhabit vastly different environments, leading to distinct adaptations in their visual capabilities. While humans enjoy a broad range of visual acuity and color perception, whales and sharks have evolved unique visual systems tailored to their aquatic worlds. This article explores the key differences in what humans can see compared to these marine animals, focusing on three critical aspects: differences in visual spectrum, depth and distance perception, and adaptations to their respective environments. By examining these areas, we can gain a deeper understanding of how each species' visual system is optimized for its specific ecological niche. Let's begin by delving into the first of these topics: differences in visual spectrum.
Differences in Visual Spectrum
The visual spectrum, a fundamental aspect of how we perceive the world, varies significantly across different species. This diversity in visual perception is a fascinating topic that highlights the unique adaptations of various creatures. For instance, humans possess a trichromatic vision system, allowing us to see a wide range of colors. In contrast, whales have monochromatic vision, which is limited to shades of gray but is highly sensitive to light and dark contrasts. Sharks, on the other hand, have limited color perception, primarily seeing the world in shades of blue and yellow. Understanding these differences not only sheds light on the evolutionary pressures that have shaped these visual systems but also underscores the remarkable complexity of vision in the natural world. Let's delve into the intricacies of human color vision first, exploring how our eyes and brains work together to create the rich tapestry of colors we experience daily.
Human Color Vision
Human color vision is a complex and highly specialized sensory capability that allows us to perceive and distinguish a wide range of colors. This is primarily facilitated by the presence of cone cells in the retina, which are sensitive to different wavelengths of light. Humans possess trichromatic vision, meaning we have three types of cone cells that respond to red, green, and blue light, respectively. This trichromatic system enables us to see a broad spectrum of colors by combining the signals from these three types of cones. In contrast to some marine animals like whales and sharks, which have limited or no color vision, humans can perceive a vast array of colors. Whales, for instance, are believed to have monochromatic vision, meaning they see the world in shades of gray only. Sharks, while having some form of dichromatic vision (sensitivity to two primary colors), do not possess the same level of color acuity as humans. This difference is crucial because it reflects the distinct evolutionary pressures and environments in which these species have developed. The human visual spectrum spans approximately 380 to 780 nanometers, encompassing the visible light range from violet to red. Within this range, our eyes can detect subtle variations in hue and saturation, allowing for detailed color perception. This capability is essential for various aspects of human life, including recognizing ripe fruits, distinguishing between different species of plants and animals, and even facilitating social interactions through facial expressions and clothing. Moreover, the structure of the human eye itself contributes significantly to our color vision. The lens focuses light onto the retina, where the cone cells are densely packed in the central area known as the fovea. This concentration of cone cells in the fovea enhances our ability to see fine details and colors in the center of our visual field. Additionally, the presence of a reflective layer called the tapetum lucidum in some animals (though not in humans) can enhance low-light vision but does not contribute to color perception. The brain also plays a critical role in processing color information. Signals from the cone cells are transmitted to the brain, where they are interpreted and combined to create our subjective experience of color. This neural processing allows for complex color constancy and context-dependent color perception, enabling us to recognize colors under varying lighting conditions. In summary, human color vision is a sophisticated system that allows us to perceive a wide range of colors due to our trichromatic visual system and the specialized structure of the human eye. This capability sets us apart from many marine animals like whales and sharks, which have more limited visual spectrums adapted to their aquatic environments. Understanding these differences highlights the unique evolutionary adaptations that have shaped the visual abilities of different species.
Whale Monochromatic Vision
Whale monochromatic vision is a fascinating aspect of marine biology that highlights the unique visual adaptations of these marine mammals. Unlike humans, who possess trichromatic vision with three types of color receptors allowing us to see a wide range of colors, whales have dichromatic or even monochromatic vision. This means they likely see the world in shades of gray or possibly with limited color perception. The primary reason for this difference lies in the structure of their eyes and the evolutionary pressures they have faced. Whales, particularly those in deep-sea environments, do not require the same level of color vision as humans. Their visual system is optimized for detecting movement and changes in brightness rather than distinguishing between various colors. This is because their natural habitat often lacks the vibrant colors present in terrestrial environments. Instead, they rely on high sensitivity to light and dark contrasts to navigate and hunt in their dimly lit surroundings. The retina of a whale contains only one or two types of cones, which are the photoreceptors responsible for color vision. In contrast, humans have three types of cones sensitive to different parts of the visible spectrum (red, green, and blue), enabling us to perceive a broad range of colors. The limited number of cone types in whales restricts their ability to see colors but enhances their sensitivity to low light levels, which is crucial for their survival in deep waters. Additionally, the lens and cornea of a whale's eye are adapted to focus light efficiently in water, where light travels differently than in air. This adaptation further supports their monochromatic vision by ensuring that any available light is maximally utilized for detecting movement and changes in brightness rather than for color perception. In summary, whale monochromatic vision is an evolutionary adaptation tailored to their aquatic environment. While it limits their ability to see colors as humans do, it enhances their ability to navigate and hunt in the dimly lit ocean depths. This difference in visual spectrum underscores the unique visual strategies employed by different species to thrive in their respective environments.
Shark Limited Color Perception
Sharks possess a unique visual system that differs significantly from that of humans and other mammals. Unlike humans, who have trichromatic vision and can perceive a wide range of colors across the visible spectrum, sharks have limited color perception. This is due to their dichromatic vision, meaning they have only two types of cones in their retina, which are sensitive to different parts of the visual spectrum. Sharks primarily see in shades of blue and yellow, with some species possibly having sensitivity to ultraviolet light as well. This dichromatic vision is adapted to their aquatic environment, where water absorbs and scatters light in ways that make certain wavelengths more or less visible. For instance, blue light penetrates deeper into water than red light, making it more useful for detecting prey or predators in the ocean's depths. The limited color perception of sharks is compensated by their highly developed sense of motion detection and contrast sensitivity. Their retinas contain a high concentration of rod cells, which are more sensitive to movement and changes in brightness than cone cells. This allows sharks to detect the slightest movements of potential prey or threats even in low-light conditions. Additionally, sharks have a reflective layer called the tapetum lucidum in the back of their eyes, which enhances their ability to see in dim light by reflecting any available light back onto the retina. This adaptation is crucial for their nocturnal and deep-sea hunting behaviors. In contrast to humans, who rely heavily on color vision for tasks such as recognizing objects and navigating complex environments, sharks rely more on spatial vision and motion cues. Their visual system is optimized for detecting and tracking prey in an environment where color is less relevant due to water's filtering effects on light. Overall, the limited color perception of sharks highlights the diverse ways in which different species adapt their visual systems to their specific ecological niches. While humans and some other terrestrial animals benefit from a broader range of color vision, sharks thrive with a visual system tailored to the unique demands of their aquatic environment.
Depth and Distance Perception
Depth and distance perception are fundamental aspects of how we navigate and understand our environment. These complex processes involve multiple visual cues and mechanisms that allow us to accurately judge the spatial relationships between objects. In this article, we will delve into three key areas that illuminate the intricacies of depth and distance perception. First, we will explore **Human Binocular Vision**, which highlights how the slight difference in images seen by each eye allows our brain to calculate depth. Next, we will examine **Whale and Shark Monocular Vision**, revealing how these marine animals, despite having only one eye visible at a time, manage to perceive their surroundings effectively. Finally, we will discuss **Human Ability to Judge Distances**, focusing on the various monocular cues such as perspective, shading, and texture that humans use to estimate distances when binocular vision is not available. By understanding these different approaches to depth and distance perception, we gain a deeper appreciation for the remarkable adaptability of visual systems across species. Let's begin by exploring the unique advantages of **Human Binocular Vision**.
Human Binocular Vision
Human binocular vision is a unique and sophisticated visual system that significantly enhances our ability to perceive depth and distance, setting us apart from many other animals, including whales and sharks. This binocular vision is made possible by the positioning of our eyes, which are placed side by side on the front of our head, allowing for overlapping fields of view. When light enters each eye, it creates two slightly different images due to the slight difference in perspective between the two eyes. The brain then processes these images to create a single, three-dimensional representation of the world. This stereoscopic vision enables humans to judge distances with remarkable accuracy. The disparity between the images seen by each eye provides the brain with crucial depth cues, allowing us to perceive the world in three dimensions rather than just two. This is particularly useful for tasks that require precise spatial awareness, such as catching a ball, navigating through crowded spaces, or even driving a car. In contrast, whales and sharks have eyes that are positioned on the sides of their heads, providing them with a wide field of view but limited binocular overlap. While this arrangement is beneficial for detecting movement and monitoring their surroundings in their respective aquatic environments, it does not allow for the same level of depth perception as human binocular vision. The brain's ability to integrate the information from both eyes also allows humans to detect subtle differences in depth that would be imperceptible to animals with monocular or limited binocular vision. This integration process involves complex neural pathways and cognitive processing, highlighting the intricate and highly developed nature of human visual perception. Furthermore, human binocular vision is not just about seeing the world in 3D; it also plays a critical role in tasks that require fine motor skills and hand-eye coordination. For example, surgeons rely heavily on their binocular vision to perform delicate procedures, and athletes use it to track and catch fast-moving objects. In summary, human binocular vision is a key factor in our ability to perceive depth and distance accurately. This unique aspect of our visual system, which is not shared by whales and sharks, significantly enhances our spatial awareness and contributes to our overall visual acuity and coordination. It underscores the remarkable adaptability and complexity of human vision, making it one of the most advanced sensory systems in the animal kingdom.
Whale and Shark Monocular Vision
Whales and sharks, despite their impressive adaptations for underwater navigation, possess monocular vision, which significantly differs from the binocular vision of humans. This fundamental difference impacts their depth and distance perception. Unlike humans, who have overlapping fields of vision that allow for stereoscopic vision and accurate depth perception, whales and sharks have eyes positioned on either side of their heads. This lateral placement means each eye sees a distinct, non-overlapping field of view, limiting their ability to perceive depth in the same way humans do. For whales, this monocular vision is compensated by other sensory mechanisms. Echolocation, a biological sonar system, allows them to emit high-frequency sounds and interpret the echoes to determine the distance, size, and shape of objects around them. This complex system is particularly effective in murky or dark waters where visual cues are minimal. However, even with echolocation, whales do not have the same level of depth perception as humans; their understanding of spatial relationships is more auditory than visual. Sharks, on the other hand, rely heavily on their lateral line system and electroreception (ampullae of Lorenzini) to navigate their environment. The lateral line detects vibrations in the water, helping sharks locate prey and avoid predators. Electroreception allows them to sense the electrical fields generated by all living creatures, providing additional spatial information. While these sensory systems are highly effective for detecting movement and locating targets, they do not provide the same level of visual depth perception as binocular vision. In contrast, humans' binocular vision enables precise depth perception through the principle of parallax. The slight difference in images seen by each eye allows the brain to calculate distances accurately. This capability is crucial for tasks requiring spatial awareness, such as driving, sports, and even simple actions like reaching for objects. The disparity between human binocular vision and the monocular vision of whales and sharks highlights a key difference in how these species interact with their environments and underscores the unique adaptations that have evolved to compensate for these visual limitations. In summary, while whales and sharks have evolved remarkable sensory systems to navigate their underwater world, their monocular vision restricts their ability to perceive depth in the same manner as humans. This difference underscores the specialized nature of each species' visual and sensory capabilities, each optimized for their specific ecological niches.
Human Ability to Judge Distances
Human ability to judge distances is a complex and multifaceted skill that relies on various visual cues and cognitive processes. This capability is crucial for navigating our environment, interacting with objects, and ensuring safety. Unlike whales and sharks, which primarily rely on echolocation and lateral lines to perceive their surroundings, humans use binocular vision and monocular cues to estimate distances. **Binocular Vision:** One of the most significant advantages humans have is binocular vision, which allows us to see the world with both eyes. The slight difference in the images seen by each eye, known as binocular disparity, helps our brain calculate depth and distance. This stereoscopic vision is particularly effective for judging distances within a few meters. **Monocular Cues:** When binocular vision is not available or sufficient, humans rely on monocular cues. These include **linear perspective**, where parallel lines appear to converge in the distance; **atmospheric perspective**, where objects appear lighter and less detailed as they recede into the distance; **size constancy**, where familiar objects are perceived as being a standard size regardless of their distance; **shading and shadow**, which provide clues about the three-dimensional structure of objects; and **motion parallax**, where the apparent motion of objects against a background helps in estimating distance. **Cognitive Factors:** Beyond these visual cues, cognitive factors also play a significant role. **Experience and learning** help refine our ability to judge distances. For instance, a person who frequently drives or plays sports may develop a better sense of spatial awareness and distance estimation. **Attention and focus** are also critical, as they allow us to selectively process relevant visual information and ignore irrelevant details. **Technological Enhancements:** In recent years, technology has further enhanced human ability to judge distances. Tools like rangefinders, GPS devices, and even smartphone apps use various methods such as laser measurement or triangulation to provide precise distance measurements. These tools are particularly useful in contexts where accurate distance estimation is critical, such as in construction, surveying, or military operations. In summary, the human ability to judge distances is a sophisticated blend of binocular vision, monocular cues, cognitive processing, and technological aids. This multifaceted approach allows humans to navigate and interact with their environment in ways that are distinct from and often superior to those of whales and sharks. Understanding these mechanisms not only highlights the remarkable adaptability of human vision but also underscores the importance of depth and distance perception in everyday life.
Adaptations to Environment
Adaptations to the environment are a cornerstone of evolutionary biology, showcasing how diverse species have evolved to thrive in various ecosystems. This article delves into three compelling examples of such adaptations, each highlighting a unique set of physiological and behavioral changes that enable survival and success in different environments. We begin by examining **Human Adaptation to Terrestrial Environment**, where humans have developed remarkable physical and cultural adaptations to inhabit a wide range of terrestrial habitats. Next, we explore **Whale Adaptation to Aquatic Environment**, detailing the extraordinary transformations that have allowed whales to dominate marine ecosystems. Finally, we look at **Shark Adaptation to Marine Predation**, revealing the specialized traits that make sharks apex predators in their domain. By understanding these adaptations, we gain insight into the intricate relationships between organisms and their environments, and how these relationships shape the evolution of life on Earth. This journey starts with an in-depth look at **Human Adaptation to Terrestrial Environment**, where we uncover the fascinating ways humans have adapted to live on land.
Human Adaptation to Terrestrial Environment
Human adaptation to the terrestrial environment is a complex and multifaceted process that has evolved over millions of years. Unlike whales and sharks, which are adapted to aquatic environments, humans have developed unique physiological, anatomical, and behavioral adaptations that enable them to thrive on land. One of the most critical adaptations is the development of lungs, which allow humans to breathe air efficiently. This contrasts with whales and sharks, which use gills to extract oxygen from water. The human respiratory system includes a diaphragm and a ribcage that facilitate the expansion and contraction of the lungs, making it possible to take in large volumes of air. Another key adaptation is the skin, which serves as a barrier against water loss and protects against external factors such as temperature fluctuations and physical damage. Human skin is relatively impermeable compared to the skin of aquatic animals, preventing excessive water loss through transpiration. Additionally, sweat glands help regulate body temperature by evaporating water from the skin surface, a mechanism not found in whales or sharks. The skeletal system of humans is also highly adapted for terrestrial life. The upright posture supported by a robust pelvis, strong leg bones, and a flexible spine allows for efficient locomotion on land. This contrasts with the streamlined bodies of whales and sharks, which are optimized for swimming. Human hands and feet are versatile, enabling activities such as walking, running, and manipulating tools—abilities that are not present in aquatic mammals. Furthermore, human vision has adapted to the terrestrial environment in ways that differ significantly from those of whales and sharks. The human eye is capable of perceiving a wide range of colors and has a high resolution for detecting details in air-filled environments. This is in contrast to the vision of aquatic animals, which often rely on low-light sensitivity and motion detection rather than color vision. Behavioral adaptations also play a crucial role in human survival on land. Humans have developed complex social structures and cultures that facilitate cooperation, communication, and the sharing of knowledge. These social behaviors enable humans to adapt to various terrestrial environments around the globe, from deserts to forests to tundras. In contrast, while whales and sharks do exhibit some social behaviors, they do not possess the same level of cognitive complexity or cultural transmission as humans. In summary, human adaptation to the terrestrial environment involves a suite of physiological, anatomical, and behavioral changes that distinguish humans from aquatic animals like whales and sharks. These adaptations have allowed humans to thrive in diverse land-based ecosystems worldwide, highlighting the remarkable flexibility and resilience of the human species.
Whale Adaptation to Aquatic Environment
Whales have evolved a suite of adaptations that enable them to thrive in the aquatic environment, showcasing remarkable specialization to their marine habitat. One of the most critical adaptations is their streamlined body shape, which minimizes drag and allows for efficient swimming. This hydrodynamic form is complemented by a thick layer of blubber, providing insulation against the cold water and serving as an energy reserve during periods of food scarcity. Their flippers, modified forelimbs, are powerful and flexible, allowing for precise maneuverability and propulsion through the water. Whales also possess a unique respiratory system adapted for life underwater. They have a blowhole on top of their head that can be closed during dives, preventing water from entering their lungs. This blowhole is connected to a highly efficient gas exchange system in the lungs, enabling them to extract oxygen from the air quickly and efficiently during brief surfacing intervals. Additionally, their heart rate slows down dramatically during dives, conserving energy and extending dive duration. Their sensory systems are equally adapted to the aquatic environment. Whales rely heavily on echolocation, using a series of clicks emitted through their melon (a fatty organ in the forehead) to navigate and locate prey in the dark depths of the ocean. This biological sonar system allows them to build detailed mental maps of their surroundings and track prey with precision. Furthermore, whales have adapted to the high pressures found at great depths. Their bodies are capable of withstanding these pressures due to the presence of flexible ribcages and lungs that can collapse without causing damage. This flexibility prevents the lungs from rupturing under pressure, allowing whales to dive to considerable depths in search of food or to escape predators. In terms of feeding adaptations, different species of whales have evolved specialized feeding mechanisms. For example, baleen whales use baleen plates to filter small organisms from the water, while toothed whales (odontocetes) employ sharp teeth to catch larger prey such as fish and squid. These feeding strategies are often accompanied by complex social behaviors, with many whale species forming large groups that cooperate in hunting and foraging activities. Overall, the adaptations of whales to their aquatic environment are a testament to the incredible diversity and resilience of life on Earth. These adaptations not only highlight the unique challenges faced by marine mammals but also underscore the remarkable evolutionary solutions that have allowed them to thrive in one of the planet's most demanding ecosystems.
Shark Adaptation to Marine Predation
Sharks have evolved a myriad of adaptations to thrive in the marine environment, where predation is a constant threat. One of the most significant adaptations is their streamlined body shape, which allows for efficient swimming and maneuverability. This sleek design enables sharks to chase down prey quickly and evade predators with agility. Additionally, their dermal denticles—small, tooth-like scales—reduce drag and enhance speed, making them formidable hunters and difficult prey. Sharks also possess exceptional sensory capabilities. Their lateral line system, a network of sensory organs along their sides, detects vibrations in the water, allowing them to locate prey and avoid predators even in murky or dark waters. The ampullae of Lorenzini, specialized electroreceptors found on their snouts, can detect the electrical fields generated by all living creatures, providing a unique form of navigation and hunting aid. Their vision is another critical adaptation; many shark species have excellent eyesight that helps them spot prey from a distance. Some species, like the great white shark, have reflective layers in the back of their eyes called tapetum lucidum, which enhance low-light vision by reflecting light back onto the retina. Furthermore, sharks' skin is covered with mucus that reduces friction and makes it harder for parasites and bacteria to adhere, thereby protecting them from infections. Their powerful jaws and teeth are designed for efficient predation; different species have specialized dentition suited to their specific diets, whether it be fish, seals, or other marine mammals. In terms of defense mechanisms, some shark species have developed spines or thorns that deter predators. For example, the spined pygmy shark has bioluminescent spots that confuse or distract potential predators. Other species exhibit camouflage strategies; the wobbegong shark blends into its surroundings with a mottled appearance that makes it nearly invisible on the seafloor. Lastly, sharks' ability to regulate their buoyancy through a liver filled with low-density oils (squalene) allows them to conserve energy while cruising or resting at depth. This adaptation is crucial for long-term survival in environments where food may be scarce. These multifaceted adaptations collectively enable sharks to dominate various marine ecosystems as apex predators while minimizing their own vulnerability to predation. Unlike whales, which rely more on size and social behavior for protection, sharks' diverse array of physical and sensory adaptations makes them uniquely suited to their role as marine predators.