What Do Stars Look Like Up Close
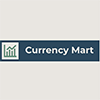
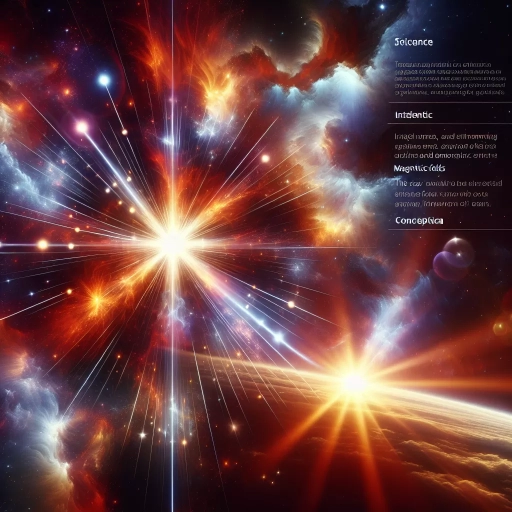
Observations of Stars Through Telescopes
Observing stars through telescopes has revolutionized our understanding of the cosmos, offering insights into the vast expanse of celestial bodies that populate our universe. This endeavor is multifaceted, involving several key techniques that collectively enhance our knowledge. First, the resolution and detail provided by advanced telescopes allow astronomers to discern finer features of stars, such as their size, temperature, and composition. Second, spectral analysis enables scientists to study the light emitted by stars, revealing their chemical makeup and evolutionary stages. Lastly, sophisticated imaging techniques capture high-quality images of stars, facilitating detailed studies of their environments and behaviors. By combining these approaches, astronomers can paint a comprehensive picture of stellar life cycles and interactions within the galaxy. In this article, we will delve into these methodologies, beginning with the critical role of resolution and detail in modern astronomical observations. --- **Resolution and Detail**
Resolution and Detail
When observing stars through telescopes, two critical factors that significantly enhance our understanding and appreciation of these celestial bodies are resolution and detail. **Resolution** refers to the telescope's ability to distinguish between two closely spaced objects, such as stars or features on a planet. This capability is crucial for astronomers because it allows them to study the fine details of stellar structures, including surface features, binary star systems, and even the presence of exoplanets. High resolution enables scientists to gather precise data on the physical properties of stars, such as their size, temperature, and composition. For instance, advanced telescopes like the Hubble Space Telescope and ground-based telescopes equipped with adaptive optics can achieve resolutions that allow us to see details that would otherwise be indistinguishable with lower-quality instruments. **Detail**, on the other hand, pertains to the clarity and richness of information obtained from these observations. It encompasses not only visual sharpness but also the depth of data collected across various wavelengths of light. Modern telescopes are designed to capture a wide range of electromagnetic radiation, from visible light to infrared and ultraviolet spectra. This multi-spectral approach provides a comprehensive view of stellar phenomena, revealing aspects such as stellar atmospheres, magnetic fields, and even the presence of stellar winds. The level of detail achieved through advanced telescopic observations has revolutionized our understanding of star formation, evolution, and death. For example, detailed spectroscopic analysis can reveal the chemical composition of a star's atmosphere, while high-resolution imaging can capture the dynamic processes occurring within stellar nurseries. The interplay between resolution and detail is particularly evident in the study of binary and multiple star systems. High-resolution imaging allows astronomers to separate closely spaced stars that would appear as a single point of light through lesser telescopes. This separation is essential for understanding the dynamics of these systems, including orbital periods and masses of the individual stars. Furthermore, detailed observations of these systems can provide insights into the formation mechanisms of stars and planetary systems. In addition to these scientific benefits, the enhanced resolution and detail offered by modern telescopes also enrich our aesthetic appreciation of stars. High-quality images captured by these instruments often reveal breathtaking beauty, showcasing vibrant colors and intricate structures that were previously invisible. These images not only inspire public interest in astronomy but also serve as powerful tools for educational outreach, helping to bridge the gap between professional research and public understanding. In summary, the combination of high resolution and detailed observations through telescopes is pivotal for advancing our knowledge of stars. By enabling scientists to discern fine details and gather comprehensive data, these capabilities transform our understanding from mere observations into profound insights into the nature of celestial bodies. As technology continues to evolve, we can expect even greater resolutions and more detailed observations, further illuminating the mysteries of the universe and deepening our connection with the stars that populate it.
Spectral Analysis
Spectral analysis is a cornerstone of modern astrophysics, allowing scientists to unravel the mysteries of celestial bodies, including stars, with unprecedented precision. When observing stars through telescopes, astronomers often rely on spectral analysis to gather detailed information about these distant luminaries. This technique involves breaking down the light emitted or absorbed by a star into its constituent colors, or spectrum, which can be analyzed to reveal a wealth of data. The process begins with the collection of light from a star using a telescope. This light is then directed through a spectrograph, an instrument that disperses the light into its spectrum. The resulting spectrum is akin to a fingerprint, unique to each star and containing vital clues about its composition, temperature, and motion. By examining the spectral lines—bright or dark bands within the spectrum—astronomers can identify specific elements present in the star's atmosphere. For instance, hydrogen, helium, and heavier elements like iron and calcium leave distinct signatures that can be detected. Spectral analysis also provides insights into a star's temperature and luminosity. Different elements emit or absorb light at specific wavelengths, which are influenced by the star's surface temperature. By analyzing these spectral lines, scientists can determine whether a star is hot and blue or cool and red. Additionally, the Doppler shift of spectral lines indicates whether a star is moving towards or away from Earth, offering valuable information about its velocity and potential companions. Furthermore, spectral analysis aids in understanding stellar evolution. By comparing the spectra of various stars at different stages of their life cycles, astronomers can trace the evolutionary paths of these celestial bodies. For example, the presence of certain spectral lines may indicate that a star is nearing the end of its life, preparing to explode as a supernova or shed its outer layers as a planetary nebula. In the context of observing stars up close, spectral analysis complements direct visual observations by providing a deeper layer of understanding. While telescopes can reveal the size, shape, and brightness of stars, spectral analysis delves into their internal workings and external behaviors. This dual approach allows astronomers to construct a comprehensive picture of what stars look like not just visually but also in terms of their physical and chemical properties. In summary, spectral analysis is an indispensable tool for astronomers seeking to understand the intricate details of stars. By deciphering the spectral signatures of these celestial bodies, scientists gain insights into their composition, temperature, motion, and evolutionary stages. This method, when combined with direct observations through telescopes, enriches our understanding of what stars look like up close and furthers our knowledge of the universe's most luminous inhabitants.
Imaging Techniques
Imaging techniques play a crucial role in our observations of stars through telescopes, allowing us to capture detailed and high-resolution images that reveal the intricate characteristics of these celestial bodies. At the heart of modern astronomical imaging are advanced technologies such as Charge-Coupled Devices (CCDs) and Complementary Metal-Oxide-Semiconductor (CMOS) sensors, which convert light into digital signals. These detectors are highly sensitive and can capture faint light from distant stars, enabling astronomers to study their luminosity, color, and spectral properties with unprecedented precision. One of the most powerful imaging techniques is Adaptive Optics (AO), which compensates for the distortion caused by Earth's atmosphere. AO systems use deformable mirrors and sophisticated algorithms to correct for atmospheric aberrations in real-time, resulting in images that are sharper and more detailed than those obtained without such correction. This technology has revolutionized ground-based astronomy, allowing telescopes like the Keck Observatory and the Very Large Telescope (VLT) to produce images that rival those from space-based telescopes. Another significant advancement is the use of interferometry, where multiple telescopes are combined to form a virtual telescope with a diameter equal to the distance between the individual telescopes. This technique, known as Very Long Baseline Interferometry (VLBI), enables astronomers to achieve angular resolutions that are orders of magnitude better than what can be achieved with a single telescope. For instance, VLBI has been used to study the environment around black holes and to image the surface of nearby stars with remarkable detail. Space-based telescopes like the Hubble Space Telescope and the James Webb Space Telescope offer additional advantages by avoiding atmospheric interference altogether. These telescopes can observe stars in wavelengths that are blocked by Earth's atmosphere, such as ultraviolet and infrared light, providing insights into stellar formation, evolution, and death. The Hubble Space Telescope's Wide Field Camera 3, for example, has captured stunning images of star-forming regions like the Carina Nebula, revealing intricate structures and processes that shape the lives of stars. Furthermore, spectroscopic imaging techniques allow astronomers to analyze the light emitted by stars in detail. By breaking down the light into its component wavelengths (spectrum), scientists can determine a star's chemical composition, temperature, and velocity. This information is crucial for understanding stellar evolution and the dynamics of star clusters and galaxies. In addition to these technological advancements, data processing and analysis play a vital role in extracting meaningful information from the vast amounts of data generated by modern imaging techniques. Advanced software tools and machine learning algorithms help in enhancing image quality, correcting for noise and artifacts, and identifying patterns that may not be immediately apparent. In summary, the combination of cutting-edge detectors, adaptive optics, interferometry, space-based observations, spectroscopy, and sophisticated data analysis has transformed our ability to observe stars up close. These imaging techniques collectively provide a comprehensive view of stellar properties and behaviors, enriching our understanding of the universe and its many mysteries. By leveraging these technologies, astronomers continue to push the boundaries of what we know about stars and their roles within the cosmos.
The Structure and Composition of Stars
The structure and composition of stars are intricate and multifaceted, involving various layers that each play a crucial role in the star's life cycle. At the heart of any star lies its core, where nuclear reactions occur, driving the star's energy output. Surrounding the core is the photosphere and atmosphere, which are responsible for the star's visible light and spectral characteristics. Finally, the outer layers and corona extend far beyond the photosphere, influencing the star's interaction with its environment. Understanding these components is essential for grasping how stars form, evolve, and eventually die. This article delves into these critical aspects, starting with the core and nuclear reactions that power a star's existence. By examining these processes, we can gain insight into the fundamental mechanisms that sustain stellar life. Let us begin by exploring the core and nuclear reactions, the central engine that drives all stellar activity.
Core and Nuclear Reactions
At the heart of stars lies a complex and dynamic process known as core and nuclear reactions, which are crucial for understanding the structure and composition of these celestial bodies. These reactions occur in the star's core, where incredibly high temperatures and pressures create an environment conducive to nuclear fusion. In main-sequence stars like our Sun, the primary process is the proton-proton chain reaction, where hydrogen nuclei (protons) fuse to form helium, releasing a tremendous amount of energy in the form of light and heat. This energy is what sustains the star's luminosity and supports its structure against gravitational collapse. The core of a star is a region of intense nuclear activity, with temperatures often exceeding millions of degrees Celsius. Here, atomic nuclei collide with such force that they overcome their mutual repulsion, allowing them to fuse into heavier elements. For example, in more massive stars, the CNO cycle becomes significant, involving carbon, nitrogen, and oxygen as catalysts in the fusion process. These reactions not only generate energy but also synthesize heavier elements from lighter ones, contributing to the star's chemical evolution. As stars age and their hydrogen fuel is depleted, they undergo significant changes. Red giant stars, for instance, expand their outer layers while contracting their cores, leading to higher core temperatures that initiate the fusion of helium into carbon and oxygen. This phase marks a critical transition in a star's life cycle, influencing its overall structure and composition. In even more advanced stages, such as those of white dwarfs or neutron stars, nuclear reactions cease, leaving behind remnants that are primarily composed of heavier elements forged during earlier phases of stellar evolution. Understanding core and nuclear reactions is essential for grasping the lifecycle of stars. These processes dictate how stars evolve from their birth in molecular clouds to their eventual demise as supernovae or other remnants. The energy output from these reactions determines a star's luminosity and surface temperature, which in turn affect its color and size. Moreover, the elements synthesized in stellar cores are dispersed into space during supernova explosions, enriching the interstellar medium with heavy elements necessary for the formation of planets and life. In summary, core and nuclear reactions are the driving force behind a star's existence, influencing its structure, composition, and lifecycle. These intricate processes not only sustain a star's luminosity but also shape its evolutionary path, ultimately contributing to the cosmic cycle of element formation and distribution. By studying these reactions, astronomers gain insights into the fundamental nature of stars and their role in the universe's chemical and physical evolution.
Photosphere and Atmosphere
When delving into the intricate structure and composition of stars, it is crucial to understand the distinct layers that comprise these celestial bodies. Two of the most critical layers are the photosphere and the atmosphere, which play pivotal roles in our understanding of stellar dynamics and appearance. The **photosphere** is the outermost layer of a star that we can see, often referred to as the "surface" of the star. It is here that the light we observe from stars is produced through a process known as radiative diffusion. The photosphere is where photons, which have been generated by nuclear reactions in the star's core, finally escape into space after being absorbed and re-emitted multiple times by the dense plasma of the star. This layer is relatively cool compared to the core, with temperatures ranging from about 5,500 degrees Celsius for stars like our Sun to tens of thousands of degrees for hotter stars. The photosphere's temperature and composition determine the star's spectral type and color, providing astronomers with valuable information about its age, size, and evolutionary stage. Above the photosphere lies the **atmosphere**, which includes several sublayers such as the chromosphere, transition region, and corona. The **chromosphere** is a thin layer just above the photosphere where temperature increases with altitude, causing it to emit light in various wavelengths, particularly in the ultraviolet and visible spectrum. This layer is visible during solar eclipses as a reddish glow around the Sun. The **transition region** is a narrow zone where temperatures rise dramatically from about 10,000 to 1 million degrees Celsius over a very short distance. Finally, the **corona**, the outermost part of the atmosphere, extends millions of kilometers into space and has temperatures of millions of degrees Celsius—far hotter than the photosphere below it. The corona is visible during total solar eclipses as a white halo around the Sun and is a key area of study for understanding solar wind and coronal heating mechanisms. Together, these layers provide a comprehensive view of what stars look like up close. The photosphere offers insights into a star's surface conditions and overall energy output, while the atmosphere reveals dynamic processes such as magnetic activity, stellar winds, and coronal heating. By studying these layers in detail, astronomers can reconstruct a star's life history, from its formation through its main sequence life to its eventual demise. This understanding not only enriches our knowledge of stellar evolution but also sheds light on the broader context of galaxy formation and the universe's overall structure. In summary, the photosphere and atmosphere are essential components in understanding the structure and composition of stars. They serve as windows into the internal workings of these celestial bodies, allowing us to decipher their physical properties, evolutionary stages, and roles within the cosmos. As we continue to explore these layers with advanced telescopes and observational techniques, we gain deeper insights into what stars look like up close—insights that are fundamental to advancing our understanding of the universe itself.
Outer Layers and Corona
The outer layers of a star, particularly the photosphere, chromosphere, and corona, play crucial roles in understanding its structure and composition. The **photosphere**, often referred to as the "surface" of the star, is the layer from which light is emitted that we can see. It is here that the star's energy, produced by nuclear reactions in the core, is finally released into space. Below the photosphere lies the **chromosphere**, a region where the temperature increases with altitude, causing it to emit light in various wavelengths, including ultraviolet and X-rays. This layer is visible during solar eclipses as a reddish glow around the Sun. Above the chromosphere extends the **corona**, a vast, tenuous outer atmosphere that can be millions of degrees hotter than the photosphere. This extreme temperature difference is still not fully understood and remains one of the most intriguing puzzles in astrophysics. The corona is composed of plasma, a gas-like state of matter where atoms are ionized, and it extends far beyond the visible disk of the star. During solar eclipses, the corona appears as a white halo around the Sun due to its scattering of sunlight. The corona's high temperature and its extension into interstellar space are key factors in understanding stellar winds and coronal mass ejections (CMEs). These phenomena involve the expulsion of charged particles and magnetic fields from the star, influencing its immediate environment and potentially affecting nearby planets. For instance, solar flares and CMEs from the Sun can cause geomagnetic storms on Earth, disrupting communication systems and satellite operations. In addition to these dynamic processes, the outer layers also provide valuable insights into a star's magnetic field and its impact on stellar activity. Magnetic fields in these regions can lead to the formation of sunspots, prominences, and other features that are indicative of a star's internal dynamics. By studying these outer layers, astronomers can infer details about a star's age, composition, and evolutionary stage. Observational techniques such as spectroscopy and imaging allow scientists to probe these outer layers in detail. For example, spectrographic analysis of light emitted by the chromosphere and corona can reveal chemical compositions and temperature profiles. Advanced telescopes equipped with coronagraphs can directly observe the corona by blocking the intense light from the photosphere, enabling detailed studies of its structure and behavior. Understanding the outer layers of stars is essential for a comprehensive view of stellar structure and composition. These regions are not just passive envelopes but active participants in the star's overall energy budget and interaction with its surroundings. By delving into the intricacies of these outer layers, researchers can gain deeper insights into the life cycles of stars and their roles within the broader cosmic landscape. This knowledge not only enhances our understanding of celestial bodies but also has practical implications for predicting space weather events that could impact Earth's technological infrastructure.
Advanced Technologies for Studying Stars Up Close
The study of stars has evolved significantly with the advent of advanced technologies, enabling scientists to observe celestial bodies with unprecedented detail and precision. At the forefront of these advancements are space missions and satellites, which have revolutionized our ability to gather data from distant stars. These missions, equipped with sophisticated instruments, can capture high-resolution images and spectra that reveal the composition, temperature, and dynamics of stellar environments. Additionally, interferometry and high-resolution imaging techniques have become crucial tools in stellar research, allowing for the combination of data from multiple telescopes to form a single, highly detailed image. Looking ahead, future prospects in stellar observation promise even more groundbreaking discoveries, leveraging emerging technologies such as next-generation telescopes and advanced computational methods. By integrating these cutting-edge approaches, scientists are poised to unravel the mysteries of star formation, evolution, and death. As we delve into the specifics of these technologies, we begin with the pivotal role of space missions and satellites in expanding our understanding of the cosmos.
Space Missions and Satellites
Space missions and satellites play a pivotal role in advancing our understanding of the cosmos, particularly when it comes to studying stars up close. These technological marvels have revolutionized astronomy by providing unprecedented access to celestial bodies that were previously beyond our reach. For instance, the Hubble Space Telescope, launched in 1990, has been a cornerstone in stellar research. With its ability to capture high-resolution images and spectra of stars, Hubble has revealed intricate details about star formation, evolution, and death. Its successor, the James Webb Space Telescope (JWST), launched in 2021, further enhances our capabilities with its advanced infrared optics, allowing scientists to peer through dust and gas clouds to observe the earliest stars in the universe. Satellites like the Kepler Space Telescope have transformed our knowledge of exoplanetary systems by detecting thousands of planets orbiting other stars. This data has not only expanded our understanding of planetary formation but also shed light on the potential for life beyond Earth. The Transiting Exoplanet Survey Satellite (TESS) continues this legacy by monitoring nearby stars for planetary transits, providing valuable insights into the diversity of planetary systems. The European Space Agency's Gaia mission is another exemplary project that has significantly contributed to stellar studies. By creating a precise three-dimensional map of the Milky Way galaxy, Gaia has enabled astronomers to determine the distances, motions, and properties of millions of stars with unprecedented accuracy. This data is crucial for understanding the structure and evolution of our galaxy. Moreover, space missions such as the Solar Dynamics Observatory (SDO) and the Parker Solar Probe focus on our nearest star, the Sun. These missions provide real-time observations of solar activity, helping scientists predict space weather events that can impact Earth's magnetic field and satellite communications. The integration of advanced technologies such as spectroscopy, interferometry, and gravitational lensing further enhances the capabilities of these space missions. Spectroscopy allows for the analysis of light emitted or absorbed by stars, revealing their chemical composition and temperature. Interferometry combines light from multiple telescopes to achieve higher resolution than any single telescope could alone, enabling detailed observations of stellar surfaces and atmospheres. Gravitational lensing exploits the bending of light around massive objects to study distant stars that would otherwise be too faint to detect. In summary, space missions and satellites are indispensable tools for studying stars up close. They leverage cutting-edge technologies to gather data that was previously unattainable, significantly advancing our understanding of stellar physics and the broader universe. As these technologies continue to evolve, we can expect even more profound insights into the nature of stars and their role in the cosmos.
Interferometry and High-Resolution Imaging
Interferometry and high-resolution imaging are pivotal advanced technologies that enable astronomers to study stars in unprecedented detail, offering a glimpse into the intricate structures and behaviors of celestial bodies. Interferometry, a technique that combines the light from multiple telescopes to form a single, high-resolution image, allows scientists to achieve resolutions far beyond what individual telescopes can provide. By synchronizing the light waves collected by separate telescopes, interferometry effectively creates a virtual telescope with a diameter equal to the distance between the farthest telescopes in the array. This method is particularly crucial for observing stars up close because it can resolve features that would otherwise be too small or too distant to be seen clearly. High-resolution imaging, often facilitated by interferometry, involves capturing detailed images of stars and their environments. Advanced optical and infrared telescopes equipped with adaptive optics systems can correct for atmospheric distortions, producing sharp images that reveal the surface details of stars. For instance, the Very Large Telescope Interferometer (VLTI) in Chile uses a combination of four 8.2-meter telescopes to achieve resolutions that are ten times better than those of the Hubble Space Telescope. This capability allows astronomers to observe phenomena such as stellar rotation, magnetic field activity, and even the presence of exoplanets. The synergy between interferometry and high-resolution imaging also extends to understanding stellar evolution. By observing the fine details of stellar surfaces, scientists can infer properties like temperature gradients, chemical composition, and surface dynamics. For example, interferometric observations have revealed complex patterns on the surfaces of red giant stars, providing insights into their internal structure and evolutionary stages. Moreover, these technologies have enabled the direct imaging of circumstellar disks around young stars, which are crucial for understanding planet formation processes. In addition to these scientific advancements, interferometry and high-resolution imaging have practical applications in astrophysics research. They facilitate the study of binary and multiple star systems, allowing astronomers to measure orbital parameters and masses with high precision. This information is essential for understanding stellar interactions and the dynamics of star clusters. Furthermore, these technologies are being refined for future missions such as the Giant Magellan Telescope and the James Webb Space Telescope, which promise even greater capabilities for studying stars up close. In summary, interferometry and high-resolution imaging represent a significant leap forward in our ability to study stars in detail. These advanced technologies not only enhance our understanding of stellar physics but also open new avenues for exploring the universe with unprecedented clarity. As these techniques continue to evolve, they will undoubtedly reveal more about the intricate lives of stars, bringing us closer to understanding the cosmos in all its complexity.
Future Prospects in Stellar Observation
As we continue to push the boundaries of stellar observation, the future prospects are nothing short of exhilarating. Advanced technologies such as next-generation telescopes, like the James Webb Space Telescope and the Giant Magellan Telescope, are poised to revolutionize our understanding of stars. These instruments will offer unprecedented resolution and sensitivity, allowing us to study stars in greater detail than ever before. For instance, the ability to observe the atmospheres of distant stars will provide insights into their chemical composition and evolutionary stages, shedding light on the formation of planetary systems and the potential for life beyond Earth. The integration of artificial intelligence and machine learning algorithms will also play a crucial role in stellar observation. These technologies will enable the analysis of vast datasets generated by modern telescopes, identifying patterns and anomalies that human observers might miss. This could lead to the discovery of new types of stars or unexpected behaviors in known stellar populations. Additionally, advancements in space missions such as the Transiting Exoplanet Survey Satellite (TESS) and the PLATO mission will continue to uncover thousands of exoplanets, many of which orbit stars that are similar to our Sun. Another significant area of development is in the field of interferometry, where multiple telescopes are combined to form a virtual telescope with the resolving power of a single telescope as large as the distance between the individual telescopes. This technique will allow scientists to study the surfaces of stars directly, observing phenomena like star spots and convective patterns that are currently beyond our reach. Furthermore, the advent of space-based interferometry missions could potentially image the immediate surroundings of black holes and neutron stars, offering a glimpse into some of the most extreme environments in the universe. The future also holds promise for direct imaging of exoplanets using advanced coronagraphs and other light-blocking technologies. These tools will help scientists study the atmospheres of exoplanets in detail, searching for biosignatures that could indicate the presence of life. Moreover, the development of new spectrographic techniques will allow for more precise measurements of stellar properties such as mass, age, and metallicity, which are crucial for understanding stellar evolution and the history of our galaxy. In conclusion, the future prospects in stellar observation are bright and multifaceted. With cutting-edge technologies on the horizon, we are on the cusp of a new era in astronomy where we can study stars up close with unprecedented clarity. These advancements will not only deepen our understanding of stellar physics but also open new avenues for exploring the cosmos and searching for life beyond Earth. As we continue to innovate and push the limits of what is possible, we may uncover secrets about the universe that have been hidden from us until now.