What Do The Following Points Describe They Have Strong Turbulent Currents
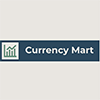
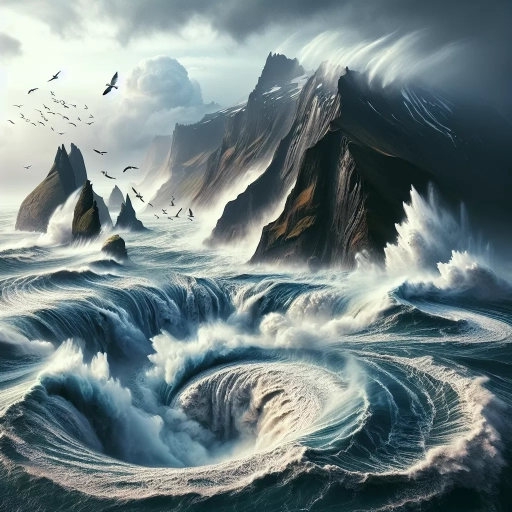
Strong turbulent currents are powerful and chaotic movements of fluid that play a crucial role in various natural and engineered systems. These currents are characterized by intense mixing and unpredictable flow patterns, which have significant implications for both the environment and technological advancements. In this article, we will delve into the **Characteristics of Strong Turbulent Currents**, exploring their defining features and how they differ from other types of fluid flows. We will also examine the **Environmental Impact of Turbulent Currents**, discussing how these currents influence ecosystems, climate patterns, and human activities. Additionally, we will look at **Technological and Scientific Approaches to Studying Turbulent Currents**, highlighting the innovative methods and tools used to understand and predict these complex phenomena. By understanding these aspects, we can better appreciate the complexity and importance of strong turbulent currents. Let us begin by examining the **Characteristics of Strong Turbulent Currents**.
Characteristics of Strong Turbulent Currents
Strong turbulent currents are characterized by several key attributes that distinguish them from other types of fluid flows. These characteristics include high velocity and energy, chaotic and unpredictable flow patterns, and significant mixing and erosion capabilities. High velocity and energy are fundamental to the nature of turbulent currents, as they drive the intense and dynamic movements within these flows. The chaotic and unpredictable nature of turbulent currents makes them challenging to model and predict, adding complexity to their study. Additionally, the significant mixing and erosion caused by these currents play crucial roles in shaping their environments, whether in oceanic or atmospheric contexts. Understanding these aspects is essential for comprehending the full impact of strong turbulent currents. By examining these characteristics, we can better grasp the mechanisms behind these powerful flows, starting with their high velocity and energy.
High Velocity and Energy
High velocity and energy are key characteristics that define strong turbulent currents. These currents are marked by rapid flow rates, often exceeding several meters per second, which significantly surpass the velocities of laminar or calm flows. The high energy associated with these currents is a result of the intense kinetic energy and momentum transfer within the fluid. This energy manifests as chaotic and irregular motions, leading to eddies and swirls that mix the fluid vigorously. The turbulence is further amplified by the Reynolds number, a dimensionless quantity that indicates the ratio of inertial to viscous forces; high Reynolds numbers typically signify turbulent flow. In natural environments, such as rivers, oceans, or atmospheric flows, these strong turbulent currents can erode landscapes, transport large amounts of sediment, and influence weather patterns. In engineering contexts, understanding these characteristics is crucial for designing structures like bridges, pipelines, and wind turbines that must withstand or harness the power of turbulent flows. Overall, the combination of high velocity and energy in turbulent currents underscores their dynamic and transformative nature.
Chaotic and Unpredictable Flow
**Characteristics of Strong Turbulent Currents** Strong turbulent currents are characterized by several key features that distinguish them from laminar flows. One of the most significant aspects is their **chaotic and unpredictable flow**. Unlike laminar flows, which are smooth and orderly, turbulent flows are marked by random and chaotic motion. This unpredictability arises from the complex interactions between various scales of fluid motion, ranging from small eddies to large-scale vortices. The chaotic nature of turbulent flows makes it challenging to predict the exact path a fluid particle will take, even with advanced computational models. This unpredictability is a direct result of the high Reynolds numbers associated with turbulent flows, which indicate a dominance of inertial forces over viscous forces. Consequently, turbulent flows exhibit a wide range of velocities and directions at any given point in space and time, leading to enhanced mixing and increased energy dissipation compared to laminar flows. Additionally, strong turbulent currents often feature **high levels of mixing and diffusion**. The chaotic motion within these flows ensures that fluid elements are rapidly mixed across different regions, leading to a more uniform distribution of properties such as temperature, concentration, and momentum. This enhanced mixing is crucial in various natural and engineering contexts, such as ocean currents, atmospheric circulation, and chemical reactors. Another characteristic of strong turbulent currents is their **high energy dissipation rates**. The random and chaotic motion within these flows results in significant frictional losses, converting kinetic energy into heat. This process is particularly evident in boundary layers where the flow interacts with solid surfaces, leading to increased drag and wear on structures like ship hulls or wind turbine blades. Furthermore, turbulent flows are often **anisotropic and inhomogeneous**. Unlike laminar flows that can be isotropic (having the same properties in all directions) and homogeneous (having the same properties at all points), turbulent flows exhibit directional dependencies and spatial variations. This anisotropy arises from the influence of external factors such as boundary conditions, shear rates, and external forces like gravity or magnetic fields. In summary, strong turbulent currents are distinguished by their chaotic and unpredictable flow patterns, high levels of mixing and diffusion, high energy dissipation rates, and anisotropic and inhomogeneous characteristics. These features make turbulent flows both fascinating and challenging to study and predict accurately. Understanding these characteristics is essential for modeling and engineering applications across various fields including fluid mechanics, meteorology, oceanography, and chemical engineering.
Significant Mixing and Erosion
Significant mixing and erosion are hallmark characteristics of strong turbulent currents. These currents, often found in oceanic and atmospheric environments, exhibit intense chaotic motion that leads to substantial mixing of fluids. Here are the key points that describe why strong turbulent currents are associated with significant mixing and erosion: 1. **High Velocity Gradients**: Strong turbulent currents have high velocity gradients, which create shear forces that break down larger fluid structures into smaller ones, enhancing mixing. 2. **Vortices and Eddies**: The presence of vortices and eddies in turbulent flows increases the surface area for mixing, allowing different fluid layers to interact more effectively. 3. **Energy Dissipation**: Turbulent currents dissipate a significant amount of energy through friction and viscosity, leading to the breakdown of larger-scale motions into smaller-scale ones, thereby facilitating mixing. 4. **Boundary Layer Interaction**: At the interface between different fluid layers or between fluids and solid boundaries, turbulent currents cause significant erosion due to the increased shear stress and kinetic energy transfer. 5. **Homogenization**: The chaotic nature of turbulent flows ensures that substances within the flow are homogenized, leading to uniform distribution of heat, nutrients, or pollutants. 6. **Enhanced Diffusion**: Turbulence enhances diffusion rates by creating pathways for substances to move more rapidly across different regions of the flow compared to laminar flows. 7. **Sediment Transport**: In aquatic environments, strong turbulent currents are capable of transporting large amounts of sediment, leading to erosion of riverbanks, coastlines, and seabeds. These characteristics collectively underscore why significant mixing and erosion are intrinsic to strong turbulent currents, making them crucial in various natural and engineered systems.
Environmental Impact of Turbulent Currents
Turbulent currents play a pivotal role in shaping our planet's environmental dynamics, with far-reaching impacts that are both profound and multifaceted. These powerful water movements not only sculpt coastal landscapes through erosion and deposition, but they also significantly affect marine ecosystems by distributing nutrients and influencing the habitats of various species. Additionally, turbulent currents contribute to global climate patterns by facilitating the transport of heat and moisture across different regions. Understanding these processes is crucial for predicting and mitigating the effects of climate change and preserving marine biodiversity. By examining the ways in which turbulent currents shape coastal landscapes, affect marine ecosystems, and influence climate patterns, we can gain a deeper appreciation for the intricate balance of our planet's systems. Let us begin by exploring how these currents reshape our coastlines, a process that is both relentless and transformative.
Shaping Coastal Landscapes
Strong turbulent currents are characterized by several key features and impacts on coastal landscapes. Here are the points that describe these currents: 1. **High Energy Levels**: Turbulent currents possess high kinetic energy, which is capable of eroding and transporting large amounts of sediment. 2. **Rapid Water Movement**: These currents involve rapid and chaotic water movements, often resulting in significant changes to shoreline morphology. 3. **Wave Action**: Strong turbulent currents are often associated with intense wave activity, which can lead to coastal erosion, dune destruction, and beach reshaping. 4. **Sediment Transport**: They are efficient at transporting sediment, leading to the formation of new landforms such as sandbars, spits, and deltas. 5. **Erosion and Deposition**: Turbulent currents cause both erosion and deposition, reshaping the coastline by removing material from one area and depositing it in another. 6. **Impact on Marine Life**: These currents can disrupt marine habitats and affect the distribution of marine species due to the constant movement and turbulence. 7. **Formation of Coastal Features**: They play a crucial role in the formation of various coastal features like ripples, dunes, and tidal flats. 8. **Human Impact**: Turbulent currents can impact human activities such as shipping, fishing, and coastal development by posing navigational hazards and altering shoreline stability. These points collectively highlight the dynamic nature of strong turbulent currents and their profound influence on shaping coastal landscapes.
Affecting Marine Ecosystems
Strong turbulent currents in marine ecosystems can have profound and far-reaching impacts. Here are the key points: 1. **Habitat Disruption**: Turbulent currents can disrupt the habitats of marine species, particularly those that rely on stable environments such as coral reefs and seagrass beds. 2. **Nutrient Redistribution**: These currents can redistribute nutrients, affecting the availability of food resources for various marine organisms, which in turn impacts the entire food chain. 3. **Species Migration and Extinction**: The intense energy of turbulent currents can force species to migrate or even lead to local extinctions if they are unable to adapt. 4. **Sediment Transport**: Turbulent currents can transport large amounts of sediment, leading to changes in seafloor topography and affecting benthic communities. 5. **Ocean Mixing**: They play a crucial role in mixing ocean waters, influencing temperature and salinity gradients, which are vital for many marine species. 6. **Coral Bleaching**: Increased turbulence can cause coral bleaching by disrupting the delicate balance between corals and their symbiotic algae. 7. **Fisheries Impact**: Changes in current patterns can affect fish migration routes and spawning grounds, impacting commercial fisheries. 8. **Coastal Erosion**: Turbulent currents can exacerbate coastal erosion, threatening coastal ecosystems and human settlements. 9. **Marine Pollution**: These currents can spread pollutants over wider areas, increasing the exposure of marine life to harmful substances. 10. **Climate Regulation**: They influence global climate patterns by facilitating the transport of heat and nutrients across different regions of the ocean. These factors collectively highlight the significant environmental impact of turbulent currents on marine ecosystems.
Influencing Climate Patterns
**Influencing Climate Patterns** Strong turbulent currents play a crucial role in shaping global climate patterns. These currents, often found in oceanic and atmospheric systems, facilitate the transfer of heat, moisture, and nutrients across vast distances. For instance, the Gulf Stream in the North Atlantic Ocean is a prime example of a strong turbulent current that significantly influences regional climates. By transporting warm water from the equatorial regions towards the poles, it moderates temperatures in Western Europe, making them milder compared to other regions at similar latitudes. Similarly, the Kuroshio Current in the Pacific Ocean has a warming effect on Japan and the eastern coast of Asia. Turbulent currents also drive global atmospheric circulation patterns. The jet stream, a fast-moving band of air in the upper atmosphere, exhibits strong turbulence and plays a key role in weather forecasting. Its meandering path can lead to significant variations in weather patterns, influencing the formation of high and low-pressure systems and the trajectory of storms. Additionally, turbulent currents within the atmosphere contribute to the distribution of greenhouse gases and aerosols, which in turn affect global climate conditions. In marine ecosystems, turbulent currents are essential for nutrient cycling and marine productivity. Upwelling regions, such as those off the coasts of Peru and California, are characterized by strong turbulent currents that bring nutrient-rich deep water to the surface. This process supports rich biodiversity and fisheries, highlighting the ecological importance of these currents. Moreover, turbulent currents can impact climate through their interaction with ice sheets and glaciers. In polar regions, ocean currents influence the melting rates of ice masses by transporting warmer waters towards them. This interaction is critical for understanding sea-level rise and global climate change. In summary, strong turbulent currents are pivotal in influencing climate patterns by redistributing heat and nutrients globally, driving atmospheric circulation, supporting marine ecosystems, and interacting with ice masses. Their dynamics are essential for understanding and predicting climate variability and change.
Technological and Scientific Approaches to Studying Turbulent Currents
The study of turbulent currents is a complex and multifaceted field that has seen significant advancements through the integration of technological and scientific approaches. To understand and predict the behavior of these dynamic fluid flows, researchers rely on a trio of key methodologies: Advanced Measurement Techniques, Computational Modeling and Simulation, and Real-Time Monitoring Systems. Advanced Measurement Techniques provide the foundational data necessary for understanding the intricate dynamics of turbulent currents. These techniques involve the use of sophisticated instruments such as particle image velocimetry (PIV) and laser Doppler velocimetry (LDV), which capture detailed velocity fields and flow patterns. Computational Modeling and Simulation complement these measurements by allowing scientists to simulate and predict the behavior of turbulent flows under various conditions, enhancing our theoretical understanding. Real-Time Monitoring Systems ensure that data is collected continuously, enabling immediate feedback and adjustments in both research and practical applications. By combining these approaches, scientists can achieve a comprehensive understanding of turbulent currents, which is crucial for fields such as oceanography, engineering, and environmental science. This article will delve into these methodologies, starting with the critical role of Advanced Measurement Techniques.
Advanced Measurement Techniques
Advanced measurement techniques play a crucial role in the study of turbulent currents, enabling scientists to capture and analyze the complex dynamics involved. Here are key points that describe strong turbulent currents: 1. **High Velocity Fluctuations**: Strong turbulent currents are characterized by significant fluctuations in velocity, which can be measured using techniques like Laser Doppler Velocimetry (LDV) or Particle Image Velocimetry (PIV). 2. **Eddy Formation**: The presence of eddies, which are rotating masses of fluid, is a hallmark of turbulent flow. Advanced measurement techniques such as Acoustic Doppler Current Profilers (ADCPs) and High-Frequency Radar can detect these eddies. 3. **Energy Dissipation**: Turbulent flows dissipate energy rapidly, which can be quantified using techniques like Direct Numerical Simulation (DNS) or Large Eddy Simulation (LES). 4. **Chaotic Behavior**: Strong turbulent currents exhibit chaotic behavior, making them unpredictable over long periods. This unpredictability is often studied using statistical methods and data from sensors like anemometers and current meters. 5. **Boundary Layer Interactions**: Turbulent flows often interact intensely with boundaries, such as riverbanks or ocean floors, which can be observed using techniques like sonar and bathymetric mapping. 6. **Vorticity**: High levels of vorticity, or the tendency to rotate, are indicative of strong turbulence. This can be measured using vortex probes or inferred from velocity data collected by instruments like ADCPs. 7. **Mixing and Dispersion**: Turbulent currents facilitate mixing and dispersion of substances within the flow, which can be tracked using dye or tracer studies combined with advanced imaging techniques. 8. **Frequency Spectra**: The frequency spectra of turbulent flows show a wide range of frequencies, indicating the presence of various scales of motion. This is typically analyzed using Fast Fourier Transform (FFT) on data from sensors like accelerometers and pressure transducers. These points collectively highlight the characteristics of strong turbulent currents and underscore the importance of advanced measurement techniques in their study.
Computational Modeling and Simulation
**Computational Modeling and Simulation** In the realm of studying turbulent currents, computational modeling and simulation play a pivotal role by offering a detailed and dynamic understanding of complex fluid dynamics. These tools enable researchers to simulate various scenarios that would be impractical or impossible to replicate in real-world experiments. Here are key points that highlight the significance of computational modeling and simulation in this context: 1. **High-Fidelity Simulations**: Computational models can capture the intricate details of turbulent flows, including eddies, vortices, and other small-scale structures that are crucial for understanding turbulence. 2. **Scalability**: Simulations can be scaled up or down to study turbulent currents at different levels, from microscopic to macroscopic scales, allowing for a comprehensive analysis. 3. **Predictive Capabilities**: Advanced algorithms and high-performance computing enable predictive simulations that forecast the behavior of turbulent currents under various conditions, aiding in the design of engineering systems and environmental assessments. 4. **Cost-Effective**: Conducting simulations is often more cost-effective than physical experiments, especially when dealing with large-scale or hazardous environments. 5. **Repeatability**: Simulations can be run multiple times with slight variations, ensuring reproducibility and allowing for systematic studies that would be challenging with physical experiments. 6. **Data Analysis**: Computational models generate vast amounts of data that can be analyzed to extract insights into the underlying mechanisms of turbulence, facilitating deeper scientific understanding. 7. **Integration with Experimental Data**: Simulations can be validated against experimental data, enhancing their accuracy and reliability, and vice versa, experimental findings can inform and improve simulation models. These capabilities make computational modeling and simulation indispensable tools for studying turbulent currents, complementing experimental approaches and providing a robust framework for technological and scientific advancements in this field.
Real-Time Monitoring Systems
Real-Time Monitoring Systems are crucial in the study of turbulent currents, providing immediate and accurate data that help scientists and engineers understand and predict these complex phenomena. Here are the key points that describe strong turbulent currents and how real-time monitoring systems support their study: 1. **High Velocity Variations**: Strong turbulent currents are characterized by rapid changes in velocity, which real-time monitoring systems can capture through advanced sensors such as acoustic Doppler velocimeters (ADVs) and particle image velocimetry (PIV). 2. **Eddy Formation**: Turbulent flows often involve the formation of eddies, which are rotating fluid masses. Real-time monitoring systems use high-resolution cameras and sensors to track these eddies in real-time, providing insights into their dynamics. 3. **Energy Dissipation**: Turbulent currents dissipate a significant amount of energy, which can be measured using real-time monitoring systems equipped with sensors that detect temperature, pressure, and flow rate changes. 4. **Flow Instabilities**: Strong turbulent currents exhibit flow instabilities that can lead to chaotic behavior. Real-time monitoring systems help identify these instabilities by continuously tracking flow parameters such as velocity gradients and shear stresses. 5. **Boundary Layer Interactions**: The interaction between turbulent flows and boundary layers is critical. Real-time monitoring systems with wall-mounted sensors can measure the impact of turbulent currents on these boundary layers in real-time. 6. **Environmental Factors**: Environmental factors like wind, waves, and tides influence turbulent currents. Real-time monitoring systems integrate data from various environmental sensors to provide a comprehensive view of these influences. 7. **Predictive Modeling**: By feeding real-time data into computational models, scientists can predict the behavior of turbulent currents more accurately. This predictive capability is essential for applications such as oceanography, meteorology, and engineering. 8. **Safety and Efficiency**: In practical applications like offshore engineering or river management, real-time monitoring of turbulent currents ensures safety by alerting to potential hazards and optimizes operations by providing timely data for decision-making. 9. **Research and Development**: Continuous real-time data from monitoring systems aid in the development of new theories and models of turbulence, advancing our scientific understanding of these complex flows. 10. **Scalability**: Modern real-time monitoring systems are scalable, allowing for the deployment of networks of sensors that can cover large areas, providing detailed spatial and temporal resolution of turbulent current dynamics. By leveraging these capabilities, real-time monitoring systems significantly enhance our ability to study and manage strong turbulent currents, contributing to both scientific knowledge and practical applications.