What Are Formula Units
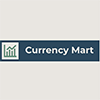
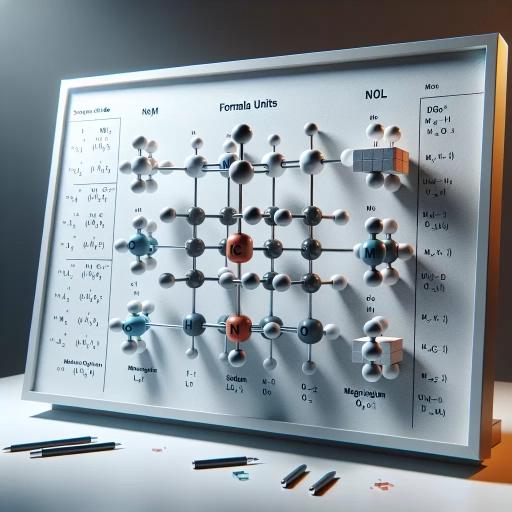
In the realm of chemistry, understanding the fundamental building blocks of compounds is crucial for grasping the intricacies of chemical reactions and structures. One such essential concept is the formula unit, which serves as a cornerstone in describing ionic compounds. This article delves into the comprehensive world of formula units, starting with their definition and concept. We will explore how formula units are defined and how they differ from molecular formulas, providing a clear understanding of their role in chemistry. Next, we will examine the various types of formula units that exist, highlighting their unique characteristics and how they are used to represent different kinds of compounds. Finally, we will discuss the practical applications and examples of formula units, illustrating their significance in real-world scenarios. By understanding these aspects, readers will gain a thorough insight into the importance and utility of formula units in chemistry. Let us begin by defining and conceptualizing what formula units are and how they are integral to our understanding of ionic compounds.
Definition and Concept of Formula Units
The concept of formula units is a fundamental aspect of chemistry, providing a concise and precise way to represent the chemical composition of ionic compounds. Unlike molecular formulas, which describe the composition of molecules, formula units are used to denote the simplest whole-number ratio of ions in an ionic compound. This distinction is crucial because it highlights the unique nature of ionic bonding compared to covalent bonding. Understanding formula units is essential for several reasons: they accurately represent the chemical composition of ionic compounds, they differentiate these compounds from those described by molecular formulas, and they play a pivotal role in various chemical processes and applications. In this article, we will delve into the definition and concept of formula units, starting with how they represent chemical composition, followed by a discussion on their difference from molecular formulas, and concluding with an exploration of their importance in chemistry. First, we will examine how formula units effectively capture the chemical composition of ionic compounds.
Chemical Composition Representation
Chemical composition representation is a fundamental concept in chemistry that allows scientists to convey the precise makeup of compounds using various notations. At its core, it involves expressing the types and numbers of atoms present in a molecule or ionic compound. The most common method of chemical composition representation is through chemical formulas, which can be molecular formulas, empirical formulas, or structural formulas. A **molecular formula** provides the exact number of each type of atom in a molecule, such as H₂O for water or C₆H₁₂O₆ for glucose. This notation is crucial for understanding the stoichiometry of chemical reactions and the properties of substances. An **empirical formula**, on the other hand, represents the simplest whole-number ratio of atoms in a compound. For example, the empirical formula for glucose is CH₂O, indicating that for every carbon atom, there are two hydrogen atoms and one oxygen atom. Empirical formulas are derived from the molecular formula but do not specify the actual number of atoms in a molecule. **Structural formulas** go a step further by showing how atoms are arranged within a molecule. These can include line-angle formulas, condensed structural formulas, and Lewis structures. Structural formulas are essential for understanding the shape and reactivity of molecules. In ionic compounds, chemical composition is often represented using **formula units**. A formula unit is the smallest whole-number ratio of ions in an ionic compound and represents the composition of the compound without indicating the actual number of atoms present. For instance, the formula unit for sodium chloride (NaCl) indicates one sodium ion and one chloride ion, while the molecular formula for water (H₂O) indicates two hydrogen atoms and one oxygen atom. Understanding these different forms of chemical composition representation is vital because they provide a concise and standardized way to communicate complex chemical information. This clarity is essential for predicting chemical properties, conducting experiments, and synthesizing new compounds. By mastering these notations, chemists can accurately describe and analyze the intricate world of chemical substances, facilitating advancements in fields ranging from pharmaceuticals to materials science. In summary, chemical composition representation through various types of formulas and structural notations is a cornerstone of chemistry, enabling precise communication about the atomic makeup of substances and facilitating deeper understanding and manipulation of chemical compounds.
Difference from Molecular Formulas
When discussing chemical formulas, it is crucial to distinguish between molecular formulas and formula units, as these terms are often confused but represent distinct concepts. A **molecular formula** specifies the exact number of atoms of each element present in a molecule. For example, the molecular formula for water is H₂O, indicating that one molecule of water consists of two hydrogen atoms and one oxygen atom. This type of formula provides detailed information about the composition of a single molecule. On the other hand, a **formula unit** is used to describe ionic compounds, which are formed by the electrostatic attraction between oppositely charged ions rather than covalent bonds. A formula unit represents the smallest whole-number ratio of ions in an ionic compound. For instance, the formula unit for sodium chloride (table salt) is NaCl, indicating that one sodium ion (Na⁺) is associated with one chloride ion (Cl⁻). Unlike molecular formulas, formula units do not necessarily represent discrete molecules but rather the simplest ratio of ions that maintains the compound's neutrality. To illustrate the difference further, consider carbon dioxide (CO₂) and calcium carbonate (CaCO₃). The molecular formula CO₂ tells us that one molecule of carbon dioxide contains one carbon atom and two oxygen atoms. In contrast, the formula unit CaCO₃ for calcium carbonate indicates that one calcium ion (Ca²⁺) is associated with one carbonate ion (CO₃²⁻), but it does not imply the existence of a discrete "molecule" of calcium carbonate in the same way that CO₂ does. Understanding this distinction is vital because it reflects the fundamental difference between covalent and ionic bonding. Covalent compounds, like water and carbon dioxide, are composed of discrete molecules held together by shared electrons. Ionic compounds, such as sodium chloride and calcium carbonate, consist of ions arranged in a lattice structure where each ion is attracted to multiple oppositely charged ions. In summary, while both molecular formulas and formula units provide essential information about chemical composition, they serve different purposes based on the type of chemical bonding involved. Molecular formulas describe covalent molecules with precise atomic counts, whereas formula units describe ionic compounds with the simplest ratio of ions necessary to maintain neutrality. This clarity is essential for accurately understanding and working with chemical compounds in various scientific contexts.
Importance in Chemistry
Chemistry plays a pivotal role in understanding the fundamental building blocks of matter and their interactions, which is crucial for defining and comprehending formula units. Formula units, a concept central to chemistry, represent the smallest whole-number ratio of ions in an ionic compound. The importance of chemistry in this context lies in its ability to elucidate the atomic structure, chemical bonding, and stoichiometry that underpin the formation of these units. Chemistry provides the tools to identify and quantify the elements present in a compound, allowing scientists to determine the exact ratio of ions necessary for a stable formula unit. This involves understanding atomic numbers, electron configurations, and valence electrons, which dictate how atoms interact and form bonds. The principles of chemical bonding—such as ionic, covalent, and metallic bonds—help explain why certain elements combine in specific ratios to form compounds. Moreover, chemistry's emphasis on stoichiometry ensures that the proportions of elements in a formula unit are accurately calculated. Stoichiometry involves the quantitative relationships between reactants and products in chemical reactions, enabling chemists to balance chemical equations and derive empirical and molecular formulas. This precision is essential for defining formula units accurately, as it ensures that the chemical composition of the compound is correctly represented. The study of chemistry also facilitates the understanding of chemical properties and behaviors that are inherent to formula units. For instance, knowing the ionic nature of a compound helps predict its solubility, melting point, and conductivity. These properties are critical in various applications across different fields, such as materials science, pharmaceuticals, and environmental science. Furthermore, chemistry's importance extends to practical applications where formula units are crucial. In materials science, understanding the formula units of compounds like ceramics and semiconductors is vital for designing materials with specific properties. In pharmaceuticals, the precise formulation of drugs relies on knowing the exact chemical composition of active ingredients and excipients. In environmental science, identifying and analyzing formula units in pollutants helps in developing strategies for pollution control and remediation. In summary, chemistry is indispensable for defining and understanding formula units due to its comprehensive framework for analyzing atomic structures, chemical bonding, stoichiometry, and chemical properties. This foundational knowledge enables precise identification and application of formula units across diverse scientific disciplines, underscoring the critical role chemistry plays in advancing our understanding of the chemical world.
Types of Formula Units in Chemistry
In the realm of chemistry, understanding the various types of formula units is crucial for grasping the fundamental principles of chemical bonding and compound formation. This article delves into three key categories: Ionic Compounds and Their Formula Units, Molecular Compounds and Their Formula Units, and Covalent Network Solids and Their Formula Units. Each category provides a unique perspective on how atoms combine to form stable chemical entities. Ionic compounds, for instance, involve the transfer of electrons between atoms, resulting in ions that are electrostatically attracted to each other. Molecular compounds, on the other hand, are formed through covalent bonds where atoms share electrons to achieve stability. Covalent network solids represent a more complex arrangement where atoms are interconnected in a continuous network of covalent bonds. By exploring these distinct types of formula units, chemists can better understand the properties and behaviors of various substances. Let's begin by examining Ionic Compounds and Their Formula Units, which serve as the foundation for understanding many inorganic substances.
Ionic Compounds and Their Formula Units
Ionic compounds are formed when electrons are transferred between atoms, resulting in the formation of ions with opposite charges. These ions then attract each other to form a stable compound. The formula unit of an ionic compound represents the simplest whole-number ratio of ions in the compound. For instance, sodium chloride (NaCl) consists of one sodium ion (Na⁺) and one chloride ion (Cl⁻). The formula unit for sodium chloride is NaCl, indicating that for every sodium ion, there is one chloride ion. In ionic compounds, the cation (positively charged ion) is typically written first, followed by the anion (negatively charged ion). The charges on the ions must balance out to form a neutral compound. For example, magnesium oxide (MgO) consists of one magnesium ion (Mg²⁺) and one oxygen ion (O²⁻). Here, the charges of the magnesium and oxygen ions balance each other out, resulting in a neutral compound. The naming of ionic compounds follows specific rules. Monatomic cations (single-atom ions) are named as the element itself, while monatomic anions are named by changing the ending of the element to "-ide." For example, sodium (Na⁺) and chloride (Cl⁻) form sodium chloride. Polyatomic ions, which are groups of atoms that act as a single ion, have unique names and are treated as single units. An example is ammonium (NH₄⁺), which forms compounds like ammonium chloride (NH₄Cl). Understanding the formula units of ionic compounds is crucial for predicting their chemical properties and behaviors. The ratio of ions in the formula unit can affect the physical properties of the compound, such as its melting point, solubility, and reactivity. For example, calcium carbonate (CaCO₃) has a different set of properties compared to sodium carbonate (Na₂CO₃) due to the different cations involved. In summary, ionic compounds are formed through the electrostatic attraction between oppositely charged ions, and their formula units represent the simplest ratio of these ions. The naming conventions and understanding of these formula units are essential for identifying and predicting the properties of ionic compounds in chemistry.
Molecular Compounds and Their Formula Units
Molecular compounds, a fundamental concept in chemistry, are composed of discrete molecules that consist of two or more nonmetal atoms chemically bonded together. Unlike ionic compounds, which form through the transfer of electrons between atoms, molecular compounds are held together by covalent bonds. These bonds involve the sharing of electron pairs between atoms to achieve a stable electronic configuration. The formula unit of a molecular compound represents the simplest whole-number ratio of atoms of each element present in the molecule. For example, water (H₂O) and carbon dioxide (CO₂) are molecular compounds where the formula units indicate one oxygen atom bonded to two hydrogen atoms and one carbon atom bonded to two oxygen atoms, respectively. The formula unit is crucial for understanding the composition and properties of the compound. In contrast to ionic compounds, which have a fixed ratio of ions due to electrostatic attraction, molecular compounds can exhibit a variety of ratios depending on the specific bonding arrangement. For instance, methane (CH₄) and ethane (C₂H₆) are both hydrocarbons but have different formula units reflecting their distinct molecular structures. The naming of molecular compounds follows specific rules. The first element in the formula is named using its full element name, while subsequent elements are named with prefixes indicating their number (e.g., mono-, di-, tri-) followed by the root of the element's name and the suffix "-ide." For example, carbon monoxide (CO) and sulfur hexafluoride (SF₆). Understanding the formula units of molecular compounds is essential for various chemical calculations and reactions. It helps in determining the molar mass of the compound, which is critical for stoichiometric calculations in chemical reactions. Additionally, knowing the formula unit aids in predicting physical properties such as boiling point and solubility, which are influenced by the intermolecular forces between molecules. In summary, molecular compounds are characterized by their covalent bonds and specific formula units that reflect their atomic composition. These formula units are vital for naming, calculating properties, and understanding the behavior of these compounds in various chemical contexts. They serve as a foundational piece of information that underpins many aspects of chemical study and application.
Covalent Network Solids and Their Formula Units
Covalent network solids are a unique class of materials where atoms are interconnected through covalent bonds, forming an extensive three-dimensional network. Unlike ionic compounds, which consist of discrete ions held together by electrostatic forces, covalent network solids have atoms that share electrons to form strong and stable bonds. This results in a rigid and often very hard structure. A prime example is diamond, where each carbon atom is tetrahedrally bonded to four neighboring carbon atoms, creating an exceptionally strong and durable material. Another well-known covalent network solid is silicon dioxide (SiO₂), commonly found in quartz and sand. Here, silicon atoms are bonded to four oxygen atoms in a tetrahedral arrangement, while each oxygen atom is bonded to two silicon atoms. This continuous network of silicon and oxygen atoms gives silicon dioxide its characteristic hardness and resistance to thermal shock. The formula units of covalent network solids do not represent discrete molecules but rather a small part of the extended structure. For instance, the formula unit for silicon dioxide is SiO₂, indicating that for every silicon atom, there are two oxygen atoms in the network. However, this does not mean that SiO₂ exists as a molecule; instead, it signifies the stoichiometric ratio of silicon to oxygen within the solid. In contrast to molecular compounds like water (H₂O) or ammonia (NH₃), which consist of discrete molecules with well-defined shapes and properties, covalent network solids do not have a specific molecular formula. Instead, their chemical composition is described by the smallest repeating unit within the network, which is often referred to as the empirical formula. The properties of covalent network solids are significantly influenced by their extended structure. They typically have high melting and boiling points due to the strong covalent bonds that must be broken for the solid to change state. Additionally, these materials often exhibit excellent thermal stability and resistance to chemical attack because of their robust bonding network. Understanding covalent network solids and their formula units is crucial in various fields such as materials science and engineering. These materials play critical roles in modern technology, from semiconductors used in electronics to advanced ceramics used in aerospace applications. Recognizing how these solids are structured at the atomic level allows scientists to design and develop new materials with tailored properties for specific applications. In summary, covalent network solids are characterized by their continuous three-dimensional networks of covalently bonded atoms. Their formula units represent the stoichiometric ratios within these networks rather than discrete molecules. This unique structure imparts exceptional physical properties, making these materials indispensable in a wide range of technological and industrial contexts.
Applications and Examples of Formula Units
Formula units are fundamental in chemistry, serving as the building blocks for understanding and analyzing chemical compounds. They are crucial for various applications, each highlighting their versatility and importance. This article delves into three key areas where formula units play a pivotal role: calculating molar mass, identifying chemical reactions, and real-world applications in materials science. By understanding how to calculate molar mass using formula units, chemists can accurately determine the mass of substances, which is essential for quantitative analysis. Identifying chemical reactions with formula units helps in predicting and balancing chemical equations, a cornerstone of chemical synthesis and analysis. Furthermore, real-world applications in materials science demonstrate how formula units are used to design and engineer new materials with specific properties. These concepts are interconnected, and a thorough grasp of each is necessary for a comprehensive understanding of chemistry. To begin, let's explore the first of these critical applications: calculating molar mass using formula units.
Calculating Molar Mass Using Formula Units
Calculating the molar mass of a compound using formula units is a fundamental concept in chemistry that allows chemists to determine the mass of one mole of a substance. This process involves several key steps. First, identify the chemical formula of the compound, which represents the ratio of atoms of each element present. For example, consider sodium chloride (NaCl), where one sodium atom is combined with one chlorine atom. Next, look up the atomic masses of the elements involved from the periodic table. The atomic mass of sodium (Na) is approximately 22.99 g/mol, and that of chlorine (Cl) is about 35.45 g/mol. To calculate the molar mass of NaCl, add these atomic masses together: 22.99 g/mol + 35.45 g/mol = 58.44 g/mol. For more complex compounds like calcium carbonate (CaCO3), the process is similar but involves multiple atoms of some elements. The atomic mass of calcium (Ca) is about 40.08 g/mol, carbon (C) is 12.01 g/mol, and oxygen (O) is 16.00 g/mol. Since there are three oxygen atoms in CaCO3, you multiply the atomic mass of oxygen by three: \(3 \times 16.00 = 48.00\) g/mol. Then add all these masses together: \(40.08 + 12.01 + 48.00 = 100.09\) g/mol. This method can be applied to any compound by summing the atomic masses of all atoms in its formula unit. It is crucial for various applications such as preparing solutions with precise concentrations and calculating reaction stoichiometry in chemical reactions. In practical terms, knowing how to calculate molar mass using formula units is essential for chemists and researchers who need to synthesize compounds or analyze their properties accurately. For instance, in pharmaceuticals, precise molar masses are critical for formulating drugs with consistent potency and efficacy. Moreover, this skill extends beyond laboratory settings into industrial processes where materials are produced on a large scale. Understanding how to calculate molar mass ensures that raw materials are used efficiently and that final products meet specific quality standards. In summary, calculating the molar mass using formula units is a straightforward yet powerful tool that underpins many aspects of chemistry and its applications across various fields. By following this method meticulously, chemists can ensure accuracy and precision in their work, whether it involves synthesizing new compounds or optimizing existing processes.
Identifying Chemical Reactions with Formula Units
Identifying chemical reactions with formula units is a fundamental skill in chemistry, enabling chemists to predict and understand the outcomes of various chemical processes. A formula unit represents the simplest whole-number ratio of atoms in a compound, which is crucial for balancing chemical equations and determining the stoichiometry of reactions. To identify a chemical reaction using formula units, one must first write the balanced chemical equation, where each reactant and product is represented by its respective formula unit. For example, in the reaction between sodium (Na) and chlorine (Cl) to form sodium chloride (NaCl), the balanced equation is \(2\text{Na} + \text{Cl}_2 \rightarrow 2\text{NaCl}\). Here, each molecule of chlorine gas reacts with two atoms of sodium to produce two formula units of sodium chloride. In more complex reactions, such as the combustion of methane (\(\text{CH}_4\)) with oxygen (\(\text{O}_2\)) to produce carbon dioxide (\(\text{CO}_2\)) and water (\(\text{H}_2\text{O}\)), the balanced equation is \(\text{CH}_4 + 2\text{O}_2 \rightarrow \text{CO}_2 + 2\text{H}_2\text{O}\). This equation shows that one formula unit of methane reacts with two formula units of oxygen to produce one formula unit of carbon dioxide and two formula units of water. Understanding these relationships allows chemists to calculate the amounts of reactants needed and products formed, which is essential in both laboratory settings and industrial processes. Moreover, identifying chemical reactions with formula units helps in recognizing different types of reactions. For instance, in a single displacement reaction like \(\text{Zn} + \text{CuSO}_4 \rightarrow \text{ZnSO}_4 + \text{Cu}\), zinc displaces copper from copper sulfate, resulting in zinc sulfate and copper. Here, each reactant and product is represented by its formula unit, facilitating the identification of the reaction type and the prediction of the products. In addition, formula units are pivotal in understanding ionic compounds and their reactions. For example, when calcium carbonate (\(\text{CaCO}_3\)) decomposes into calcium oxide (\(\text{CaO}\)) and carbon dioxide (\(\text{CO}_2\)), the balanced equation is \(\text{CaCO}_3 \rightarrow \text{CaO} + \text{CO}_2\). Each reactant and product is represented by its formula unit, highlighting the stoichiometric relationships between them. In summary, identifying chemical reactions with formula units is a critical aspect of chemistry that allows for the accurate prediction and analysis of chemical processes. By understanding these relationships, chemists can balance equations, determine reaction stoichiometry, and recognize different types of chemical reactions, all of which are essential for both theoretical and practical applications in chemistry.
Real-World Applications in Materials Science
Materials science, the study of the properties and applications of various materials, has numerous real-world applications that significantly impact our daily lives. One of the fundamental concepts in materials science is the formula unit, which represents the simplest whole-number ratio of atoms in a compound. Understanding formula units is crucial because it allows scientists and engineers to predict and manipulate the properties of materials. In **electronics**, for instance, the precise formulation of semiconductor materials is essential for creating efficient solar cells, LEDs, and transistors. Silicon, a key component in these devices, is often alloyed with other elements like boron or phosphorus to create p-type or n-type semiconductors. The formula units of these compounds (e.g., SiB or SiP) dictate their electrical conductivity and performance. In **biomedical engineering**, materials scientists develop biomaterials with specific formula units to ensure compatibility with biological tissues. For example, hydroxyapatite (Ca₅(PO₄)₃(OH)), a calcium phosphate compound, is used in bone grafts and dental implants due to its structural similarity to natural bone tissue. The precise formulation of hydroxyapatite ensures biocompatibility and mechanical strength. **Energy storage** also relies heavily on materials science. Lithium-ion batteries, which power many modern devices, contain lithium cobalt oxide (LiCoO₂) as the cathode material. The formula unit of this compound is critical for its electrochemical properties, enabling efficient charge and discharge cycles. In **construction**, advanced materials like high-strength concrete and fiber-reinforced polymers are designed with specific formula units to enhance durability and resistance to environmental factors. For example, the addition of silica fume (SiO₂) to concrete improves its compressive strength and reduces permeability, making it ideal for high-performance applications. **Aerospace engineering** demands materials with exceptional strength-to-weight ratios and resistance to extreme temperatures. Titanium alloys, such as Ti-6Al-4V (titanium-aluminum-vanadium), are widely used in aircraft and spacecraft due to their unique combination of mechanical properties, which are directly related to their formula units. Furthermore, **environmental science** benefits from materials science through the development of catalysts and sorbents. Zeolites, for instance, are microporous aluminosilicates (e.g., Na₂Al₂Si₂O₈·2H₂O) that can be tailored to capture specific pollutants or catalyze chemical reactions, making them invaluable in air and water purification systems. In summary, understanding and applying the concept of formula units in materials science is pivotal for creating and optimizing a wide range of materials that underpin various technological advancements across multiple industries. By controlling the chemical composition at the atomic level, scientists can design materials with tailored properties, leading to significant improvements in performance, efficiency, and sustainability.