What Is A Somatic Cell
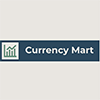
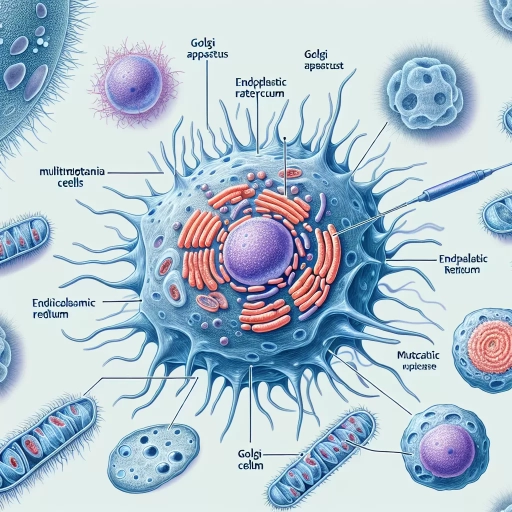
Somatic cells are the fundamental building blocks of our bodies, comprising the vast majority of cells in multicellular organisms. These cells, distinct from reproductive cells (gametes), play a crucial role in maintaining the integrity and function of various tissues and organs. Understanding somatic cells is essential for grasping the intricacies of human biology and health. This article delves into the world of somatic cells, starting with **Understanding the Basics of Somatic Cells**, where we explore their definition, types, and life cycle. We then examine **The Structure and Function of Somatic Cells**, detailing their cellular components and how these contribute to their diverse roles in the body. Finally, we discuss **The Significance of Somatic Cells in Health and Disease**, highlighting their impact on overall well-being and their involvement in various pathological conditions. By understanding these aspects, we gain a deeper appreciation for the vital role somatic cells play in our lives. Let us begin by **Understanding the Basics of Somatic Cells**.
Understanding the Basics of Somatic Cells
Understanding the basics of somatic cells is crucial for grasping the fundamental biology of human health and development. Somatic cells, which make up the majority of our body, play a vital role in maintaining our overall well-being. To delve into this topic, it is essential to explore three key aspects: the definition and origin of somatic cells, the various types that exist, and their roles within the human body. Firstly, understanding the definition and origin of somatic cells sets the foundation for comprehending their significance. Somatic cells are non-reproductive cells that arise from the zygote during embryonic development and differentiate into various cell types to form tissues and organs. This foundational knowledge is critical for appreciating how these cells contribute to our bodily functions. Secondly, recognizing the diverse types of somatic cells helps in understanding their specialized roles. From epithelial cells that form protective barriers to muscle cells that enable movement, each type of somatic cell has a unique function that contributes to the intricate machinery of the human body. Lastly, examining the role of somatic cells in the human body reveals their importance in maintaining health and responding to disease. These cells are involved in everything from metabolic processes to immune responses, highlighting their indispensable role in our daily lives. By exploring these three facets—definition and origin, types of somatic cells, and their roles—we can gain a deeper appreciation for the complex and vital functions these cells perform. Let us begin by examining the definition and origin of somatic cells to lay the groundwork for this comprehensive understanding.
Definition and Origin
**Definition and Origin** Somatic cells, a fundamental component of multicellular organisms, are non-reproductive cells that make up the bulk of an organism's body. Unlike germ cells, which are involved in reproduction, somatic cells perform a wide array of functions essential for the organism's survival and development. The term "somatic" originates from the Greek word "somatikos," meaning "of the body," reflecting their role in forming and maintaining the body's tissues and organs. The origin of somatic cells can be traced back to the earliest stages of embryonic development. During embryogenesis, the fertilized egg undergoes several cell divisions, leading to the formation of a blastocyst. This stage is crucial because it marks the differentiation between the inner cell mass (ICM) and the trophoblast. The ICM gives rise to the embryoblast, which eventually develops into the three primary germ layers: ectoderm, mesoderm, and endoderm. These germ layers are the precursors to all somatic cells in the body, each layer giving rise to specific types of tissues and organs. For instance, the ectoderm develops into skin and nervous tissue, the mesoderm forms muscles, bones, and blood vessels, and the endoderm gives rise to the lining of the digestive tract and other internal organs. Understanding the definition and origin of somatic cells is pivotal for grasping their diverse roles within an organism. Somatic cells can be further classified based on their functions and locations within the body. For example, epithelial cells form the lining of glands and other body surfaces; connective tissue cells provide support and structure; muscle cells facilitate movement; and nervous cells transmit signals. Each type of somatic cell has unique characteristics and responsibilities that contribute to the overall health and function of the organism. Moreover, somatic cells undergo various processes such as mitosis, apoptosis (programmed cell death), and differentiation, which are essential for growth, repair, and maintenance of tissues. The ability of somatic cells to regenerate and replace damaged or aged cells is a critical aspect of tissue homeostasis. However, this regenerative capacity can sometimes be disrupted, leading to diseases such as cancer, where uncontrolled cell division and loss of cellular differentiation occur. In summary, somatic cells are the building blocks of multicellular life, originating from the early stages of embryonic development and differentiating into various types to perform specialized functions. Their roles are multifaceted and vital for maintaining the integrity and function of an organism's body. Understanding these cells' definition and origin provides a foundational knowledge necessary for exploring their complex biology and significance in health and disease.
Types of Somatic Cells
Somatic cells, which comprise the majority of cells in the human body, are crucial for maintaining the structure and function of tissues and organs. These cells are non-reproductive, meaning they do not participate in the formation of gametes (sperm or eggs) and are not involved in the transmission of genetic material to offspring. There are several types of somatic cells, each with distinct roles and characteristics. **Epithelial Cells**: These cells form the lining of various body surfaces and cavities, such as the skin, respiratory tract, gastrointestinal tract, and urinary system. Epithelial cells can be further classified into squamous, cuboidal, and columnar types based on their shape and function. For example, squamous epithelial cells are flat and provide a smooth surface for gas exchange in the lungs or protection against external factors in the skin. **Connective Tissue Cells**: These cells support other tissues and bind them together. They include fibroblasts, which produce collagen and other fibers that give strength to connective tissue; adipocytes (fat cells), which store energy; and osteoblasts and osteoclasts, which are involved in bone formation and resorption. Connective tissue also includes blood cells like erythrocytes (red blood cells), leukocytes (white blood cells), and thrombocytes (platelets), which circulate through the vascular system. **Muscle Cells**: Muscle cells or muscle fibers are specialized for contraction and movement. There are three main types: skeletal muscle cells (also known as striated muscle), smooth muscle cells, and cardiac muscle cells. Skeletal muscle cells are attached to bones and facilitate voluntary movements. Smooth muscle cells are found in the walls of hollow organs like the digestive tract and blood vessels, enabling involuntary contractions. Cardiac muscle cells form the heart and are responsible for pumping blood throughout the body. **Nervous System Cells**: These include neurons and glial cells. Neurons transmit signals through electrical impulses, allowing for communication between different parts of the body. Glial cells support neurons by providing nutrients, removing waste products, and insulating neurons with myelin sheaths to enhance signal transmission speed. Each type of somatic cell has unique structural adaptations that enable it to perform its specific functions efficiently. For instance, epithelial cells often have tight junctions to prevent leakage across their surfaces, while muscle cells contain actin and myosin filaments that slide past each other during contraction. Understanding these different types of somatic cells is essential for appreciating how they contribute to overall health and how their dysfunction can lead to various diseases. In summary, somatic cells are diverse and play vital roles in maintaining the integrity and function of the human body. Their specialized structures and functions allow them to perform a wide range of tasks necessary for survival, from protecting against external pathogens to facilitating movement and communication within the body. This diversity underscores the complexity and beauty of cellular biology and highlights why studying somatic cells is fundamental to understanding human health and disease.
Role in Human Body
Somatic cells play a pivotal role in the human body, constituting the vast majority of cells that make up our tissues and organs. These cells are non-reproductive, meaning they do not participate in the formation of gametes (sperm or eggs), and their primary function is to maintain the integrity and functionality of various bodily systems. From the skin that protects us from external damage to the muscles that enable movement, somatic cells are essential for every aspect of our daily lives. In the skin, somatic cells such as keratinocytes and melanocytes work together to form a barrier against pathogens and UV radiation. Keratinocytes produce keratin, a protein that provides strength and rigidity to the skin, while melanocytes produce melanin, which protects against harmful UV rays. In the musculoskeletal system, muscle cells (fibers) are somatic cells that contract to facilitate movement and maintain posture. These muscle fibers are organized into muscles that are attached to bones via tendons, allowing for a wide range of motions. The nervous system, comprising neurons and glial cells, is another critical area where somatic cells are indispensable. Neurons transmit signals that enable communication between different parts of the body, while glial cells support neurons by providing them with nutrients and removing waste products. In the circulatory system, endothelial cells line blood vessels, regulating blood pressure and ensuring proper blood flow to all tissues. Somatic cells also form the bulk of our internal organs. For instance, in the digestive system, epithelial cells line the gastrointestinal tract and facilitate nutrient absorption. In the respiratory system, alveolar cells in the lungs are responsible for gas exchange—oxygenating the blood and removing carbon dioxide. The liver's hepatocytes detoxify harmful substances and metabolize nutrients, while pancreatic islet cells produce hormones like insulin to regulate blood sugar levels. Moreover, somatic cells have a remarkable ability to regenerate and repair damaged tissues. For example, skin cells can rapidly proliferate to heal wounds, and liver cells can regenerate lost tissue following injury. This regenerative capacity is crucial for maintaining overall health and preventing disease progression. In addition to their functional roles, somatic cells are also involved in immune responses. Dendritic cells and macrophages, for instance, recognize pathogens and present antigens to T-cells, initiating an immune response that helps protect against infections. Understanding the role of somatic cells is fundamental to appreciating how our bodies function at a cellular level. Their diverse functions underscore their importance in maintaining homeostasis and ensuring that our bodily systems operate efficiently. By recognizing the critical contributions of these cells, we can better comprehend the intricate mechanisms that govern human health and disease. This knowledge is essential for developing targeted therapies and treatments aimed at restoring or enhancing cellular function when it is compromised by injury or disease.
The Structure and Function of Somatic Cells
Somatic cells, which comprise the majority of cells in the human body, play a crucial role in maintaining overall health and function. Understanding their structure and function is essential for grasping how our bodies operate at a cellular level. This article delves into the intricate details of somatic cells, exploring three key aspects: cellular components and organelles, metabolic processes and energy production, and cell division and reproduction. By examining the diverse array of organelles within somatic cells, we can appreciate how these specialized structures contribute to cellular activities such as protein synthesis, waste management, and energy generation. Additionally, understanding the metabolic pathways and energy production mechanisms within these cells provides insight into how they sustain life. Finally, the processes of cell division and reproduction are vital for tissue repair, growth, and the maintenance of cellular homeostasis. To begin, let's focus on the foundational aspect of somatic cells: their cellular components and organelles, which form the backbone of cellular function and efficiency.
Cellular Components and Organelles
**Cellular Components and Organelles** At the heart of every somatic cell lies a complex array of cellular components and organelles, each playing a crucial role in maintaining cellular integrity and function. The **plasma membrane**, a semi-permeable lipid bilayer, encases the cell and regulates the exchange of materials between the cell and its environment. Beneath this membrane, the **cytoplasm** serves as a dynamic medium where various metabolic processes occur, housing organelles that are specialized for different functions. The **nucleus**, often referred to as the "control center" of the cell, contains most of the cell's genetic material in the form of DNA. It is enveloped by a double membrane called the nuclear envelope, which is perforated by nuclear pores that allow for the passage of molecules. The **mitochondria**, known as the "powerhouses" of the cell, generate energy through cellular respiration, converting glucose into ATP (adenosine triphosphate). These organelles are particularly abundant in cells with high energy demands, such as muscle cells. Other key organelles include **ribosomes**, which are responsible for protein synthesis; **endoplasmic reticulum (ER)**, which comes in two forms—rough ER with ribosomes for protein synthesis and smooth ER involved in lipid synthesis and detoxification; **Golgi apparatus**, which modifies, sorts, and packages proteins and lipids for transport out of the cell; and **lysosomes**, membrane-bound sacs containing digestive enzymes that break down and recycle cellular waste. Additionally, **peroxisomes** are involved in the breakdown of fatty acids and amino acids, while **centrioles** play a role in the formation of cilia, flagella, and the spindle fibers that separate chromosomes during cell division. **Cytoskeleton**, comprising microtubules, microfilaments, and intermediate filaments, provides structural support, shape maintenance, and facilitates cell movement and division. Each organelle works in concert with others to ensure proper cellular function. For instance, proteins synthesized by ribosomes attached to the rough ER are further processed by the Golgi apparatus before being secreted or integrated into cellular membranes. This intricate network of organelles underscores the complexity and efficiency of cellular processes within somatic cells, highlighting their critical role in maintaining tissue health and overall organismal function. Understanding these components is essential for grasping how somatic cells contribute to the diverse range of biological activities that sustain life.
Metabolic Processes and Energy Production
Metabolic processes are the cornerstone of cellular function, particularly in somatic cells, which are the building blocks of tissues and organs in multicellular organisms. These processes are intricately linked to energy production, ensuring that cells maintain their structural integrity, perform specialized functions, and respond to environmental cues. At the heart of cellular metabolism lies the conversion of nutrients into energy, primarily through the breakdown of carbohydrates, fats, and proteins. The journey begins with glycolysis, a universal metabolic pathway that converts glucose into pyruvate in the cytoplasm of somatic cells. This initial step generates a small amount of ATP (adenosine triphosphate), the primary energy currency of the cell. Pyruvate then enters the mitochondria, where it is further processed in the citric acid cycle (Krebs cycle), producing additional ATP, NADH, and FADH2 as byproducts. These electron carriers play a crucial role in the electron transport chain, a series of protein complexes embedded in the mitochondrial inner membrane that harness the energy from NADH and FADH2 to generate a proton gradient. This gradient drives the production of ATP through the process of chemiosmosis, significantly amplifying the energy yield from glucose. In addition to glycolysis and the citric acid cycle, somatic cells also engage in fatty acid oxidation and amino acid metabolism to diversify their energy sources. Fatty acids are broken down into acetyl-CoA units, which enter the citric acid cycle, while amino acids can be converted into intermediates that feed into various metabolic pathways. These processes are tightly regulated by feedback mechanisms and allosteric control, ensuring that energy production is balanced with cellular needs. Moreover, somatic cells have evolved adaptive strategies to cope with varying energy demands. For instance, during periods of high energy expenditure or when oxygen levels are low, cells can resort to anaerobic glycolysis, producing lactate instead of pyruvate. This allows for rapid ATP production without the need for oxygen, albeit at a lower efficiency. Conversely, when energy is abundant, cells can store it in the form of glycogen or triglycerides for future use. The efficiency and flexibility of these metabolic pathways are critical for maintaining cellular homeostasis and supporting the diverse functions of somatic cells. For example, muscle cells rely heavily on glycolysis and fatty acid oxidation to fuel contraction and relaxation, while nerve cells utilize a high rate of oxidative phosphorylation to sustain the electrical activity necessary for signal transmission. The intricate balance between different metabolic routes ensures that somatic cells can adapt to changing conditions, from periods of rest to intense activity, thereby underpinning the overall health and function of tissues and organs. In summary, the metabolic processes in somatic cells are finely tuned to optimize energy production from various substrates. This complex interplay between glycolysis, the citric acid cycle, fatty acid oxidation, and amino acid metabolism not only sustains cellular activities but also enables somatic cells to respond dynamically to environmental challenges. Understanding these processes is essential for appreciating the structure and function of somatic cells and their pivotal role in maintaining the integrity and function of multicellular organisms.
Cell Division and Reproduction
Cell division and reproduction are fundamental processes that underpin the life cycle of somatic cells, which are the non-reproductive cells that make up the majority of an organism's body. These processes ensure the growth, repair, and maintenance of tissues, allowing organisms to develop, adapt, and survive. At the heart of cell division lies the intricate mechanism of mitosis, a highly regulated series of steps that result in the precise duplication of genetic material and the division of a single cell into two genetically identical daughter cells. This process begins with interphase, where the cell grows, replicates its DNA, and prepares for division. The replicated DNA then condenses into visible chromosomes during prophase, followed by metaphase where these chromosomes align at the cell's equator. Anaphase ensues with the separation of sister chromatids to opposite poles of the cell, culminating in telophase where nuclear envelopes reform around each set of chromosomes. Finally, cytokinesis splits the cytoplasm, resulting in two daughter cells. This meticulous process is crucial for somatic cell reproduction because it ensures genetic continuity and stability within an organism. For instance, skin cells undergo rapid mitosis to replace damaged or dead cells, while muscle cells divide to facilitate growth and repair tissues after injury. The precision of mitosis is maintained by a complex system of checkpoints that monitor each phase for errors or damage, halting the process if necessary to prevent the propagation of defective cells. This safeguard mechanism is vital for preventing uncontrolled cell growth and mutations that could lead to diseases such as cancer. In addition to mitosis, another form of cell division known as meiosis occurs in reproductive cells (gametes), but its principles highlight the broader significance of accurate cell division. Meiosis involves two successive divisions that reduce the chromosome number by half, ensuring genetic diversity through recombination and independent assortment. While meiosis is specific to gametes, understanding its mechanisms provides insights into the broader importance of precise genetic segregation in all cell divisions. The ability of somatic cells to undergo mitosis also underscores their role in tissue regeneration and homeostasis. Stem cells, a subset of somatic cells with the capacity for self-renewal and differentiation into various cell types, play a pivotal role in this context. They can proliferate through mitosis to maintain their population while also differentiating into specialized cells to replace those lost due to injury or normal turnover. This dual capability ensures that tissues remain functional and healthy throughout an organism's life. In summary, cell division and reproduction are essential for the maintenance and function of somatic cells. Mitosis ensures that these cells can proliferate accurately, maintaining genetic integrity and supporting tissue growth and repair. The precision and regulation of this process are critical for preventing genetic errors and ensuring the overall health of an organism. Understanding these mechanisms provides a deeper appreciation for the intricate biology underlying the structure and function of somatic cells.
The Significance of Somatic Cells in Health and Disease
Somatic cells, which comprise the majority of cells in the human body, play a pivotal role in maintaining health and combating disease. Their significance extends across various dimensions, each highlighting a different facet of their importance. Firstly, somatic cells are crucial in tissue repair and regeneration, enabling the body to heal from injuries and maintain tissue integrity. Secondly, these cells have profound implications in genetic disorders, as mutations within them can lead to a range of diseases. Lastly, somatic cells influence aging and longevity, with their function and resilience determining the overall healthspan of an individual. Understanding the role of somatic cells in these areas is essential for developing effective therapeutic strategies. By delving into their role in tissue repair and regeneration, we can uncover the mechanisms that underpin the body's ability to heal and adapt, setting the stage for innovative treatments that leverage these cellular processes to restore health and function.
Role in Tissue Repair and Regeneration
Somatic cells play a pivotal role in tissue repair and regeneration, a process that is crucial for maintaining tissue integrity and function. When tissues are damaged due to injury, disease, or wear and tear, somatic cells are key players in the healing process. This involves several intricate steps, starting with the activation of immune cells to clear debris and pathogens, followed by the proliferation and differentiation of somatic cells to replace damaged tissue. One of the primary mechanisms through which somatic cells contribute to tissue repair is through their ability to proliferate. For instance, in the skin, keratinocytes and fibroblasts rapidly divide to cover wounds and restore the epithelial barrier. Similarly, in muscle tissue, satellite cells—a type of stem cell—are activated to proliferate and differentiate into new muscle fibers, thereby restoring muscle function after injury. Another critical aspect of somatic cell involvement in tissue repair is their role in producing growth factors and cytokines. These signaling molecules orchestrate the repair process by recruiting other cells, promoting angiogenesis (the formation of new blood vessels), and enhancing the deposition of extracellular matrix components necessary for structural support. For example, platelet-derived growth factor (PDGF) secreted by platelets and fibroblasts stimulates the migration and proliferation of fibroblasts and smooth muscle cells, which are essential for wound closure. In addition to their direct participation in repair processes, somatic cells also contribute indirectly by supporting the microenvironment that fosters regeneration. For instance, in the liver, hepatocytes and stellate cells work together to maintain a balance between inflammation and tissue regeneration following liver injury. Hepatocytes can regenerate themselves through a process known as compensatory hyperplasia, while stellate cells modulate the inflammatory response and produce matrix components that support new tissue growth. Moreover, advances in stem cell biology have highlighted the potential of somatic cells to be reprogrammed into induced pluripotent stem cells (iPSCs), which can differentiate into various cell types. This technology holds promise for regenerative medicine by enabling the generation of patient-specific cells for tissue repair and replacement therapies. For example, iPSCs derived from skin fibroblasts can be differentiated into cardiomyocytes or neurons, offering new avenues for treating heart disease or neurological disorders. In summary, somatic cells are indispensable for tissue repair and regeneration due to their capacity for proliferation, differentiation, and secretion of growth factors. Their role in maintaining tissue homeostasis and responding to injury underscores their significance in health and disease. Understanding the mechanisms by which somatic cells contribute to tissue repair not only sheds light on natural healing processes but also opens up new possibilities for therapeutic interventions aimed at enhancing regeneration and restoring function in damaged tissues.
Implications in Genetic Disorders
The implications of genetic disorders are far-reaching and multifaceted, significantly impacting both individuals and society as a whole. At the core of these disorders are mutations or alterations in the DNA sequence of somatic cells, which can lead to a wide array of health issues. For instance, genetic mutations in somatic cells can result in cancer, as seen in the case of tumor suppressor genes or oncogenes. When these genes are mutated, they can lose their ability to regulate cell growth and division, leading to uncontrolled proliferation and tumor formation. This not only affects the individual's quality of life but also places a substantial burden on healthcare systems due to the need for extensive treatments such as chemotherapy, radiation therapy, and surgery. Moreover, genetic disorders in somatic cells can manifest in various other forms, including autoimmune diseases like rheumatoid arthritis or lupus. In these conditions, genetic predispositions can lead to an overactive immune response where the body mistakenly attacks its own tissues. The implications here are twofold: patients suffer from chronic inflammation and tissue damage, while healthcare providers must manage complex treatment regimens that balance immune suppression with the risk of infections. Additionally, genetic disorders affecting somatic cells can have profound psychological and social implications. Conditions such as Huntington's disease or early-onset Alzheimer's disease, which are caused by specific genetic mutations, can lead to cognitive decline and behavioral changes that affect not only the individual but also their family and community. The emotional toll on caregivers and the economic burden of long-term care facilities highlight the broader societal impact of these disorders. From a public health perspective, understanding the genetic basis of somatic cell disorders is crucial for developing targeted therapies and preventive measures. For example, genetic screening programs can identify individuals at risk for certain conditions, allowing for early intervention and potentially altering disease trajectories. Furthermore, advances in gene editing technologies like CRISPR hold promise for treating or even curing some genetic disorders by correcting the underlying mutations in somatic cells. In conclusion, the implications of genetic disorders in somatic cells are vast and varied, influencing individual health outcomes, healthcare systems, and societal well-being. As research continues to unravel the complexities of these disorders, it is clear that a deeper understanding of somatic cell genetics will be pivotal in developing effective treatments and improving quality of life for those affected. This underscores the significance of somatic cells in health and disease, emphasizing their role as a critical frontier in medical science and public health policy.
Impact on Aging and Longevity
The impact of somatic cells on aging and longevity is a complex and multifaceted topic that has garnered significant attention in recent scientific research. Somatic cells, which comprise the majority of cells in the human body and are responsible for various physiological functions, play a crucial role in determining an individual's lifespan. As we age, somatic cells undergo a series of changes that can either promote or hinder longevity. One key aspect is the accumulation of cellular damage over time. Somatic cells are susceptible to DNA mutations, epigenetic alterations, and mitochondrial dysfunction, all of which can lead to cellular senescence or apoptosis (programmed cell death). Cellular senescence, in particular, has been linked to aging as senescent cells can secrete pro-inflammatory factors that contribute to tissue dysfunction and chronic inflammation, accelerating the aging process. Conversely, mechanisms such as DNA repair pathways and telomere maintenance help mitigate these effects, thereby supporting cellular health and longevity. Another critical factor is the role of stem cells, a subset of somatic cells with the ability to self-renew and differentiate into various cell types. Stem cells are essential for tissue regeneration and homeostasis, but their function declines with age. This decline can lead to reduced tissue repair capabilities, contributing to the manifestations of aging such as decreased muscle mass, bone density loss, and impaired immune function. Furthermore, lifestyle factors and environmental exposures can significantly influence the health and longevity of somatic cells. For instance, a diet rich in antioxidants and a regular exercise regimen can enhance cellular resilience by reducing oxidative stress and promoting mitochondrial health. Conversely, exposure to harmful substances like tobacco smoke or excessive UV radiation can accelerate cellular aging through increased DNA damage. Recent advances in senolytic therapy—a therapeutic approach aimed at eliminating senescent cells—have shown promise in improving healthspan (the period of life spent in good health) by reducing the burden of senescent cells. This emerging field highlights the potential for targeted interventions to enhance somatic cell health and extend healthy lifespan. In summary, the health and function of somatic cells are pivotal in determining an individual's aging trajectory and overall longevity. Understanding the intricate mechanisms by which these cells age or remain resilient can provide valuable insights into developing strategies to promote healthy aging and extend lifespan. As research continues to unravel the complexities of somatic cell biology, it is clear that maintaining the integrity and function of these cells is crucial for achieving optimal health outcomes across the lifespan.