What Does Atp Mean In Text
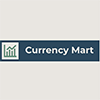
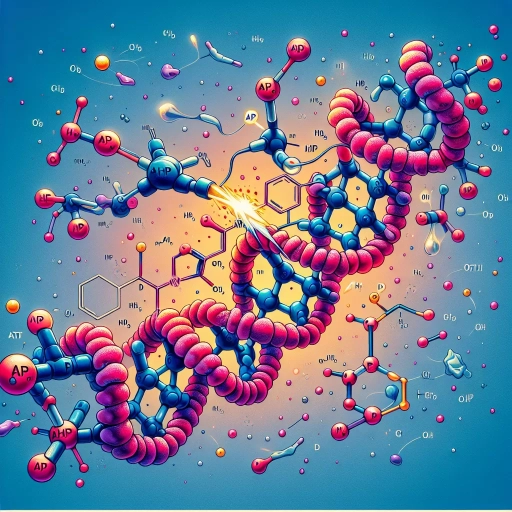
In the vast and dynamic landscape of modern communication, acronyms and abbreviations have become integral to our daily interactions. Among these, "ATP" stands out as a term that can have multiple meanings depending on the context. For many, ATP is synonymous with Adenosine Triphosphate, the molecular currency of energy in living organisms. However, in text-based communication, particularly in online forums and social media, ATP can signify something entirely different. This article delves into the multifaceted nature of ATP, exploring its various interpretations and implications. We will begin by **Understanding the Basics of ATP in Text**, where we dissect its common usage and origins. From there, we will delve into **Technical and Scientific Aspects of ATP**, examining its role in biology and technology. Finally, we will discuss **Practical Applications and Cultural Significance of ATP**, highlighting how this term intersects with everyday life and cultural narratives. By the end of this journey, readers will have a comprehensive understanding of what ATP means in different contexts, starting with the foundational knowledge presented in **Understanding the Basics of ATP in Text**.
Understanding the Basics of ATP in Text
Adenosine triphosphate, commonly abbreviated as ATP, is a molecule that serves as the primary energy carrier in cells, playing a crucial role in various biological processes. To fully grasp the significance of ATP, it is essential to delve into its fundamental aspects. This article will explore the definition and origin of ATP, highlighting its chemical structure and how it is synthesized within cells. Additionally, we will examine the common usage contexts of ATP, including its role in energy transfer during metabolic processes and its involvement in cellular activities such as muscle contraction and protein synthesis. Finally, we will trace the historical development of ATP, from its discovery to the current understanding of its mechanisms and functions. By understanding these core elements, readers will gain a comprehensive insight into the basics of ATP, enabling them to appreciate its vital role in sustaining life at the cellular level. Transitioning into the heart of this discussion, let us begin by understanding the basics of ATP in text.
Definition and Origin
**Definition and Origin** Adenosine triphosphate (ATP) is the molecular currency of energy transfer within cells, playing a pivotal role in various biological processes. At its core, ATP is a nucleotide composed of adenine, a sugar molecule called ribose, and three phosphate groups. The energy stored in the phosphate bonds of ATP is released when these bonds are hydrolyzed, a process that fuels numerous cellular activities such as muscle contraction, protein synthesis, and membrane transport. The concept of ATP was first introduced by German chemist Karl Lohmann in 1929, who isolated it from muscle tissue. However, it was not until the 1940s that the full significance of ATP as an energy carrier became widely recognized through the work of Fritz Lipmann and other biochemists. The origin of ATP can be traced back to ancient metabolic pathways that evolved in early life forms. In these primordial organisms, ATP likely served as a versatile molecule capable of storing and releasing energy efficiently. Over billions of years, this molecule has remained central to cellular metabolism, with its structure and function being highly conserved across different species. This universality underscores the fundamental importance of ATP in sustaining life as we know it. Understanding the basics of ATP is crucial for grasping how cells operate at a molecular level. For instance, during cellular respiration, glucose is broken down to produce ATP through a series of complex biochemical reactions. This process involves the electron transport chain, where electrons are passed through a series of protein complexes in the mitochondrial inner membrane, generating a proton gradient that drives ATP synthesis. Similarly, in photosynthesis, light energy is captured by chlorophyll and converted into chemical energy stored in ATP and NADPH. In the context of human physiology, ATP is essential for maintaining muscle function and nerve activity. During physical exercise, muscles rely heavily on ATP to fuel contractions; when ATP stores are depleted, muscles fatigue. Additionally, ATP plays a critical role in neurotransmission by powering the release and uptake of neurotransmitters at synapses. In summary, ATP's definition as an energy-rich molecule and its origin from ancient metabolic pathways highlight its indispensable role in cellular biology. From powering basic cellular functions to supporting complex physiological processes, ATP remains at the heart of life's energetic machinery. Understanding these basics provides a solid foundation for exploring more advanced topics related to ATP and its multifaceted roles in living organisms.
Common Usage Contexts
In the context of understanding the basics of ATP in text, it is crucial to explore its common usage contexts. ATP, or Adenosine Triphosphate, is a molecule that serves as the primary energy carrier in cells, but its acronym has been adopted in various other fields due to its versatility and recognizability. In sports and fitness, ATP is frequently discussed as the energy currency that fuels muscle contractions and other cellular processes, making it a central topic in discussions about athletic performance and endurance training. For instance, athletes and trainers often focus on strategies to optimize ATP production and utilization to enhance performance during high-intensity activities. In scientific and educational settings, ATP is a fundamental concept taught in biology and biochemistry classes. Here, it is explained as a molecule that stores energy in the form of phosphate bonds, which are broken down to release energy for cellular processes. This context highlights the biochemical significance of ATP in cellular metabolism, including its role in glycolysis, the citric acid cycle, and oxidative phosphorylation. Beyond its biological significance, the term "ATP" has also been borrowed in other domains. In technology and computing, ATP can stand for Application Transaction Protocol or Automated Testing Platform, depending on the context. For example, in software development, an Automated Testing Platform (ATP) is used to automate the testing of software applications to ensure they meet certain standards and functionalities. In business and finance, ATP might refer to Accelerated Tax Payment or Alternative Trading Platform. For instance, an Alternative Trading Platform (ATP) provides a venue for trading securities outside traditional stock exchanges, offering flexibility and sometimes lower costs for traders. In everyday communication, especially in text messages or social media posts, "ATP" could be used colloquially to mean "Answer The Phone" or "At The Present," depending on the context of the conversation. This informal usage underscores how acronyms can be repurposed for convenience and brevity in digital communication. Understanding these diverse contexts is essential for accurately interpreting references to ATP across different fields. Whether discussing cellular energy, athletic performance, software testing, financial transactions, or casual communication, recognizing the specific context in which ATP is used ensures clear and effective communication. This multifaceted nature of the term underscores its adaptability and importance in various aspects of modern life.
Historical Development
The historical development of ATP (Adenosine Triphosphate) as a concept in biology is a fascinating narrative that underscores the evolution of scientific understanding. The journey began in the late 19th century when scientists first started to unravel the mysteries of cellular metabolism. In 1929, the German biochemist Karl Lohmann isolated ATP from muscle tissue, marking a significant milestone. However, it was not until the 1940s that the role of ATP as an energy carrier became more clearly defined. The work of Fritz Lipmann, who introduced the concept of "energy-rich phosphate bonds," laid the groundwork for understanding how ATP functions within cells. The 1950s and 1960s saw a surge in research on ATP, particularly with the discovery of the electron transport chain by Peter Mitchell. His chemiosmotic theory explained how ATP is generated during oxidative phosphorylation, revolutionizing the field of bioenergetics. This period also witnessed the elucidation of glycolysis and the citric acid cycle, further solidifying ATP's central role in cellular energy production. In the latter half of the 20th century, advances in molecular biology and structural biology provided detailed insights into ATP's molecular structure and its interactions with enzymes. The discovery of ATP synthase by Paul Boyer and John Walker in the 1980s revealed the mechanism by which ATP is synthesized during cellular respiration. This work earned them the Nobel Prize in Chemistry in 1997. Today, our understanding of ATP extends beyond its role as an energy carrier to include its involvement in various cellular processes such as DNA synthesis, muscle contraction, and neurotransmission. Modern techniques like single-molecule microscopy and computational modeling continue to refine our knowledge of ATP's dynamics and interactions at the molecular level. Understanding the historical development of ATP highlights not only the scientific rigor but also the collaborative effort that has shaped our current comprehension of cellular energy metabolism. From its initial isolation to the detailed mechanistic insights of today, the story of ATP serves as a testament to human curiosity and the relentless pursuit of scientific knowledge. This historical context is crucial for appreciating the fundamental role that ATP plays in sustaining life at the cellular level, making it an essential component of any discussion on cellular biology and metabolism.
Technical and Scientific Aspects of ATP
Adenosine triphosphate (ATP) is a molecule that plays a pivotal role in the biological processes of living organisms, serving as the primary energy currency of the cell. To fully appreciate its significance, it is essential to delve into the technical and scientific aspects that underpin its function. This article will explore three critical dimensions of ATP: its chemical structure and function, its role in cellular energy transfer, and its involvement in various biological processes. Understanding the intricate details of ATP's chemical structure is crucial for grasping how it stores and releases energy. Additionally, examining its role in cellular energy transfer will reveal how ATP facilitates the metabolic activities necessary for life. Finally, exploring the diverse biological processes involving ATP will highlight its central position in maintaining cellular homeostasis and supporting life's complex functions. By dissecting these key aspects, we can gain a comprehensive understanding of ATP's fundamental importance, setting the stage for a deeper exploration of its role in cellular biology. Understanding the basics of ATP is essential for appreciating its multifaceted contributions to life, and this article aims to provide a detailed and engaging overview of these critical aspects.
Chemical Structure and Function
Chemical structure and function are intricately linked in the context of ATP (Adenosine Triphosphate), a molecule that serves as the primary energy currency of cells. ATP's structure consists of three main components: a nitrogenous base called adenine, a sugar molecule known as ribose, and three phosphate groups. The phosphate groups are arranged in a linear fashion, with the last phosphate group being the most critical for energy storage and transfer. This specific arrangement allows ATP to store energy in the form of phosphate bonds, which can be easily broken and reformed to release or capture energy. The function of ATP is deeply rooted in its chemical structure. When cells require energy, ATP undergoes hydrolysis, a process where the last phosphate group is cleaved off, releasing energy that can be used to perform various cellular tasks such as muscle contraction, protein synthesis, and membrane transport. This reaction converts ATP into ADP (Adenosine Diphosphate) and inorganic phosphate. Conversely, during energy-rich conditions, cells can replenish ATP by adding a phosphate group back to ADP through a process known as phosphorylation, often driven by cellular respiration or photosynthesis. The unique chemical properties of ATP's phosphate bonds make it an ideal molecule for energy transfer. The high-energy phosphate bonds have a significant negative free energy change, meaning that when these bonds are broken, a substantial amount of energy is released. This energy is then harnessed by enzymes and other cellular machinery to drive essential biochemical reactions. Moreover, the versatility of ATP's structure allows it to interact with a wide range of enzymes and proteins, facilitating its role in diverse cellular processes. For instance, ATP-binding proteins can recognize and bind to the specific shape and charge distribution of ATP, enabling precise control over energy-dependent reactions. In summary, the chemical structure of ATP is perfectly suited to its function as a universal energy carrier in cells. The arrangement of its phosphate groups allows for efficient energy storage and release, while its interactions with various cellular components ensure that energy is utilized precisely where it is needed. This synergy between structure and function underscores the critical role of ATP in maintaining cellular homeostasis and supporting life's complex processes.
Role in Cellular Energy Transfer
In the intricate dance of cellular metabolism, ATP (Adenosine Triphosphate) plays a pivotal role in energy transfer, serving as the primary currency of energy within cells. This molecule is crucial for various cellular processes, including muscle contraction, protein synthesis, and membrane transport. The structure of ATP, comprising a nucleotide base (adenine), a sugar molecule (ribose), and three phosphate groups, is key to its function. The energy stored in the phosphate bonds of ATP is released when these bonds are hydrolyzed to form ADP (Adenosine Diphosphate) and inorganic phosphate, a process known as dephosphorylation. This energy release is harnessed by cells to perform work. The role of ATP in cellular energy transfer is multifaceted. During cellular respiration, particularly in the mitochondria, ATP is synthesized through the processes of glycolysis, the citric acid cycle, and oxidative phosphorylation. In glycolysis, glucose is broken down into pyruvate, generating a small amount of ATP. The citric acid cycle further breaks down pyruvate, producing more ATP, NADH, and FADH2. These electron carriers then fuel the electron transport chain in oxidative phosphorylation, where the majority of cellular ATP is produced through the process of chemiosmosis. ATP's versatility extends beyond energy production; it also acts as a signaling molecule and a cofactor in various enzymatic reactions. For instance, ATP is involved in the regulation of metabolic pathways by acting as an allosteric inhibitor or activator for key enzymes. Additionally, ATP-binding cassette (ABC) transporters utilize ATP hydrolysis to pump molecules across cell membranes against concentration gradients, essential for nutrient uptake and waste removal. The efficiency and speed at which ATP can be synthesized and utilized make it an ideal energy carrier. Cells maintain a dynamic balance between ATP production and consumption, ensuring that energy is available on demand. This balance is tightly regulated by feedback mechanisms that adjust metabolic rates according to cellular needs. For example, when ATP levels are high, glycolysis and the citric acid cycle are inhibited to prevent excessive energy production. In summary, ATP's central role in cellular energy transfer underpins the metabolic activities of cells. Its unique structure allows it to store and release energy efficiently, making it indispensable for maintaining cellular homeostasis and supporting the diverse functions necessary for life. Understanding the technical and scientific aspects of ATP highlights its critical importance in cellular biology and underscores why it is often referred to as the "molecular unit of currency" for intracellular energy transfer.
Biological Processes Involving ATP
Adenosine triphosphate (ATP) is the molecular currency of energy within cells, playing a pivotal role in various biological processes that sustain life. At the heart of cellular metabolism, ATP is synthesized and utilized in a continuous cycle that fuels the vast array of biochemical reactions essential for cellular function. During cellular respiration, ATP is produced through the breakdown of glucose and other organic molecules in processes such as glycolysis, the citric acid cycle, and oxidative phosphorylation. In glycolysis, glucose is converted into pyruvate, generating a small amount of ATP and NADH. The citric acid cycle further breaks down pyruvate, producing more ATP, NADH, and FADH2. These electron carriers then feed into the electron transport chain during oxidative phosphorylation, where the majority of ATP is generated through the process of chemiosmosis. Beyond energy production, ATP is crucial for muscle contraction and relaxation. In muscle cells, ATP binds to myosin heads, allowing them to detach from actin filaments and move along the filament, causing muscle contraction. The hydrolysis of ATP to ADP and inorganic phosphate releases energy that powers this movement. Similarly, in nerve cells, ATP is involved in neurotransmitter release and uptake. The transport of neurotransmitters across synaptic vesicles and their subsequent release into the synaptic cleft are energy-dependent processes facilitated by ATP. In addition to these roles, ATP is integral to active transport mechanisms that maintain cellular homeostasis. For instance, the sodium-potassium pump uses ATP to transport sodium ions out of the cell and potassium ions into the cell against their concentration gradients, helping to regulate membrane potential and ion balance. ATP also drives the synthesis of macromolecules such as proteins and nucleic acids by providing the necessary energy for peptide bond formation and nucleotide polymerization. Furthermore, ATP participates in signal transduction pathways that regulate cellular activities in response to external stimuli. For example, in the phosphoinositide signaling pathway, ATP is required for the phosphorylation of proteins that activate downstream signaling cascades. This intricate web of ATP-dependent processes underscores its central role in maintaining cellular integrity and function. In summary, ATP's multifaceted involvement in energy production, muscle contraction, neurotransmission, active transport, and signal transduction highlights its indispensable status as a molecular energy carrier. Its dynamic interplay with various cellular components ensures that cells can perform their diverse functions efficiently, making ATP a cornerstone of biological processes.
Practical Applications and Cultural Significance of ATP
Adenosine triphosphate (ATP) is the molecular currency of energy within cells, playing a pivotal role in various biological processes. Beyond its biochemical significance, ATP has profound practical applications and cultural resonance that extend far beyond the realm of cellular metabolism. This article delves into the multifaceted importance of ATP, exploring its medical and health implications, where it serves as a critical component in diagnosing and treating diseases. We also examine the technological innovations driven by ATP, including advancements in bioenergy and biotechnology. Additionally, we uncover the cultural references and symbolism associated with ATP, highlighting its presence in literature, art, and popular culture. By understanding these diverse aspects, we can appreciate the full spectrum of ATP's influence on human society. To fully grasp these applications, it is essential to first understand the basics of ATP, which will be detailed in the following section: **Understanding the Basics of ATP**.
Medical and Health Implications
Adenosine triphosphate (ATP) is a molecule that plays a pivotal role in the biological processes of living organisms, and its implications extend far beyond its function as an energy carrier. In the context of medical and health, ATP's significance is multifaceted. Firstly, ATP is crucial for cellular metabolism, powering various biochemical reactions that sustain life. Any disruption in ATP production or utilization can lead to severe health issues. For instance, mitochondrial diseases, which affect the mitochondria's ability to produce ATP, result in conditions such as muscular dystrophy and neurodegenerative disorders. Understanding ATP's role in these diseases has led to the development of therapeutic strategies aimed at enhancing mitochondrial function. Moreover, ATP's involvement in cellular signaling pathways makes it a key player in immune responses and inflammation. ATP can act as an extracellular signaling molecule, influencing the behavior of immune cells and modulating inflammatory reactions. This has important implications for conditions like arthritis and autoimmune diseases, where dysregulated immune responses are central to the pathology. Research into ATP's signaling mechanisms has opened avenues for novel therapeutic approaches targeting these pathways to mitigate inflammation and restore immune homeostasis. In addition to its direct biological roles, ATP has practical applications in medical diagnostics and treatments. For example, ATP bioluminescence assays are used to detect microbial contamination in clinical settings, ensuring the sterility of medical instruments and environments. In cancer therapy, targeting ATP-dependent pathways has emerged as a promising strategy to inhibit tumor growth and metastasis. Drugs that interfere with ATP production or utilization can selectively kill cancer cells by depriving them of the energy needed for proliferation. Culturally, the understanding of ATP's role in human health has influenced public awareness about the importance of maintaining cellular energy balance. This knowledge has driven interest in lifestyle choices that support mitochondrial health, such as regular exercise and a balanced diet rich in nutrients that enhance ATP production. The cultural significance of ATP extends to its symbolic representation in popular media, often depicted as a molecule of life or energy, reinforcing its critical role in sustaining life processes. In conclusion, the medical and health implications of ATP are profound and far-reaching. From its central role in cellular metabolism to its involvement in immune responses and potential therapeutic targets, ATP remains a cornerstone of biomedical research. Its practical applications in diagnostics and treatments underscore its importance in clinical settings, while its cultural impact highlights the broader societal appreciation for the intricate mechanisms that underpin human health. As research continues to unravel the complexities of ATP's functions, it is clear that this molecule will remain at the forefront of medical advancements for years to come.
Technological Innovations
Technological innovations have revolutionized the way we live, work, and interact, significantly impacting both practical applications and cultural significance. At the heart of these advancements is the concept of ATP (Adenosine Triphosphate), which, while primarily known as the energy currency of cells in biology, also serves as a metaphor for technological energy and efficiency. In practical terms, technological innovations have transformed industries such as healthcare, finance, and education. For instance, advancements in medical technology have led to the development of personalized medicine, where genetic data and AI algorithms are used to tailor treatments to individual patients. Similarly, fintech innovations have enabled secure and instantaneous transactions across borders, fostering global economic connectivity. In education, virtual learning platforms have democratized access to knowledge, allowing students from diverse backgrounds to engage with high-quality educational content. Culturally, these technological innovations have reshaped societal norms and behaviors. Social media platforms, for example, have redefined how we communicate and form communities. They have also raised important questions about privacy, misinformation, and digital literacy. The rise of remote work has blurred the lines between personal and professional life, necessitating new forms of work-life balance. Moreover, technological advancements in entertainment have given birth to immersive experiences like virtual reality and augmented reality, which are changing the way we consume media and interact with each other. The integration of technology into daily life has also led to significant cultural shifts. For instance, the proliferation of smartphones has made information accessible at our fingertips, altering how we navigate the world and make decisions. E-commerce has transformed consumer behavior, with online shopping becoming a norm that challenges traditional retail models. Furthermore, the Internet of Things (IoT) has connected our homes, cars, and even wearables, creating a network of interconnected devices that enhance convenience but also raise concerns about data security and privacy. In conclusion, technological innovations are not just tools; they are drivers of societal change. They have practical applications that improve efficiency and productivity across various sectors while also influencing cultural norms and behaviors. As we continue to harness the power of technology, it is crucial to consider both its benefits and its challenges to ensure that these innovations serve humanity's best interests. The metaphorical connection between ATP and technological energy underscores the importance of understanding how these advancements can energize our lives while also requiring careful management to avoid unintended consequences.
Cultural References and Symbolism
In the realm of cultural references and symbolism, ATP (Adenosine Triphosphate) transcends its biochemical role to embody various metaphors and analogies that resonate deeply within human society. As the primary energy carrier in cells, ATP is often symbolically linked to concepts of vitality, power, and efficiency. In literature and art, it can represent the driving force behind life's processes, much like how it fuels cellular activities. For instance, in science fiction narratives, characters might harness ATP as a metaphor for unlocking human potential or overcoming existential challenges. This symbolism extends into everyday language where phrases like "energizing" or "recharging" can be seen as analogous to the ATP cycle, highlighting the universal quest for renewal and sustenance. In educational contexts, ATP serves as a powerful teaching tool. By illustrating how ATP molecules are synthesized and broken down to release energy, educators can explain complex biochemical processes in a way that resonates with students' everyday experiences. This pedagogical approach not only enhances understanding but also fosters an appreciation for the intricate mechanisms underlying life. Furthermore, the concept of ATP has inspired technological innovations such as bio-batteries and energy storage systems, reflecting humanity's relentless pursuit of efficient energy solutions. Culturally, ATP's significance is also reflected in its role within traditional practices and beliefs. For example, in some indigenous cultures, rituals involving physical exertion are seen as ways to harness and balance life's energies—a concept that parallels the biochemical function of ATP. Similarly, in modern wellness trends, the idea of "energy" is often tied to dietary supplements and lifestyle choices aimed at optimizing ATP production in the body. The practical applications of ATP extend far beyond its biological function; it has become a cultural touchstone for discussions around energy, vitality, and sustainability. In environmental discourse, the efficiency of ATP production in cells is frequently cited as an ideal model for developing sustainable energy technologies. This cross-disciplinary appeal underscores how scientific concepts can permeate various aspects of human culture, enriching our understanding of both the natural world and our place within it. Ultimately, the cultural significance of ATP lies in its ability to bridge scientific knowledge with broader societal themes. By recognizing these connections, we can better appreciate the profound impact that biochemical processes have on our collective imagination and daily lives. Whether through literature, education, technology, or traditional practices, ATP stands as a compelling example of how scientific concepts can be woven into the fabric of human culture.