What Is A Spectrometer
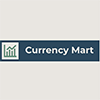
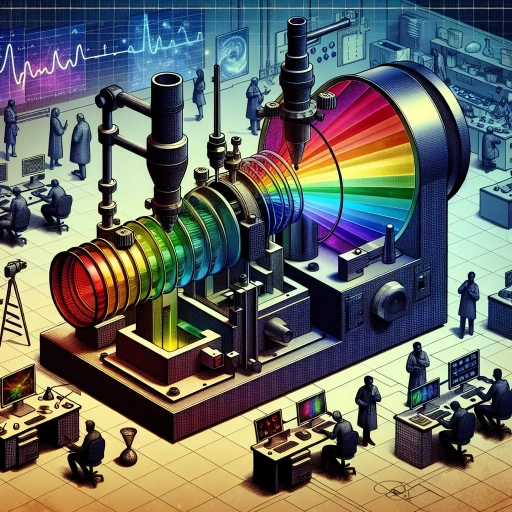
A spectrometer is a versatile scientific instrument that plays a crucial role in various fields, including chemistry, physics, and biology. By analyzing the interaction between matter and electromagnetic radiation, spectrometers provide valuable insights into the composition and properties of substances. This article delves into the world of spectrometers, exploring their fundamental principles, diverse types, and extensive applications. We begin by introducing the basics of spectrometers, explaining how they work and what they measure. Next, we will examine the different types of spectrometers, each designed for specific analytical tasks. Finally, we will discuss the wide range of applications and uses of spectrometers, highlighting their importance in research, industry, and everyday life. To understand the full scope of these powerful tools, let's start with an **Introduction to Spectrometers**, where we will uncover the foundational concepts that underpin their functionality.
Introduction to Spectrometers
Spectrometers are sophisticated scientific instruments that have revolutionized various fields by enabling the precise analysis of the interaction between matter and electromagnetic radiation. At the heart of their functionality lies a deep understanding of their definition and basic principles, which form the foundation for their widespread applications. This article delves into the world of spectrometers, starting with a detailed exploration of their definition and basic principles. We will examine how these instruments work, including the key components and processes involved in spectral analysis. Historically, the development of spectrometers has been a journey marked by significant milestones and innovations. From the early beginnings with prisms and gratings to the advanced technologies of today, each step has contributed to the enhancement of spectral resolution and accuracy. Understanding this historical context provides valuable insights into how spectrometers have evolved to meet the demands of modern science. The importance of spectrometers extends across multiple disciplines, including chemistry, physics, biology, and environmental science. They play a crucial role in identifying and quantifying substances, analyzing chemical structures, and monitoring environmental changes. By grasping the fundamental principles of spectrometers, we can better appreciate their impact on these fields and the critical role they play in advancing scientific knowledge. Transitioning to the core of our discussion, it is essential to begin with the definition and basic principles of spectrometers. This foundational knowledge will serve as a springboard for understanding their historical development and their significance across various scientific domains.
Definition and Basic Principles
A spectrometer is an essential scientific instrument that plays a crucial role in various fields such as chemistry, physics, and biology. At its core, a spectrometer is defined as a device that measures the interaction between matter and electromagnetic radiation. This interaction can be observed in terms of absorption, emission, or scattering of light, which provides valuable information about the composition and properties of the material being analyzed. The basic principles of spectrometry revolve around the concept that different substances absorb or emit light at specific wavelengths. When light passes through a sample, certain wavelengths are absorbed by the atoms or molecules, while others are transmitted or reflected. By analyzing these interactions, spectrometers can identify the presence of specific elements or compounds within a sample. This is achieved through the use of a light source, a sample holder, a dispersing element (such as a prism or grating), and a detector. One of the fundamental principles underlying spectrometry is the Beer-Lambert Law, which describes the relationship between the concentration of a substance and the amount of light it absorbs. According to this law, the absorbance of light by a sample is directly proportional to the concentration of the absorbing species and the path length of the light through the sample. This principle allows for quantitative analysis, enabling researchers to determine not only the identity but also the concentration of substances present in a sample. Spectrometers operate across various regions of the electromagnetic spectrum, including ultraviolet (UV), visible (Vis), infrared (IR), and even X-ray and gamma-ray regions. Each region provides unique insights into different aspects of molecular structure and interactions. For instance, UV-Vis spectroscopy is commonly used to study electronic transitions in molecules, while IR spectroscopy is used to analyze vibrational modes, providing detailed information about molecular bonds and functional groups. The versatility of spectrometers extends beyond simple identification; they are also instrumental in understanding complex phenomena such as chemical reactions, molecular interactions, and environmental monitoring. In environmental science, spectrometers help in detecting pollutants and monitoring their levels in air, water, and soil. In medical research, they aid in diagnosing diseases by analyzing biological samples for specific biomarkers. In addition to their analytical capabilities, modern spectrometers have evolved to incorporate advanced technologies such as Fourier transform spectroscopy (FTS) and mass spectrometry. FTS enhances resolution and sensitivity by using interferometry to generate high-resolution spectra. Mass spectrometry, on the other hand, combines separation techniques with spectroscopic analysis to identify and quantify complex mixtures at the molecular level. In conclusion, spectrometers are powerful tools that leverage the principles of light-matter interaction to provide detailed insights into the chemical and physical properties of substances. Their ability to operate across different spectral regions and their integration with advanced technologies make them indispensable in various scientific disciplines. Understanding these basic principles is essential for harnessing the full potential of spectrometry in research, diagnostics, and industrial applications.
Historical Development
The historical development of spectrometers is a rich and fascinating narrative that spans centuries, reflecting the evolving understanding of light, matter, and their interactions. The journey began in the early 17th century with the work of Isaac Newton, who first demonstrated the composition of white light by passing it through a prism in his groundbreaking experiment in 1666. This foundational discovery laid the groundwork for the study of spectra and the development of early spectrometers. In the 19th century, scientists such as Joseph von Fraunhofer and Gustav Kirchhoff made significant contributions. Fraunhofer's detailed observations of the solar spectrum led to the identification of numerous dark lines, now known as Fraunhofer lines, which are crucial for understanding atomic absorption. Kirchhoff and Robert Bunsen's work on emission spectra in the 1850s further solidified the connection between spectral lines and chemical elements, paving the way for spectroscopy as a tool in chemistry. The late 19th and early 20th centuries saw the invention of more sophisticated spectrometers. The development of photographic plates allowed for the recording of spectra with greater precision, while advancements in optics and mechanics enabled the construction of more accurate and versatile instruments. The introduction of quantum mechanics by Max Planck, Albert Einstein, and Niels Bohr provided a theoretical framework for understanding the spectral behavior of atoms and molecules. This period also witnessed the emergence of different types of spectrometers, including mass spectrometers, which were pioneered by J.J. Thomson and F.W. Aston, and infrared spectrometers, which were developed in response to the need for analyzing molecular vibrations. The mid-20th century marked a significant leap forward with the advent of electronic and digital technologies. The invention of the photomultiplier tube and subsequent improvements in detector technology enhanced sensitivity and speed. The integration of computers into spectrometer design allowed for real-time data processing and analysis, revolutionizing the field. Modern spectrometers now incorporate advanced materials, sophisticated software, and innovative techniques such as Fourier transform spectroscopy, which significantly improve resolution and efficiency. Today, spectrometers are indispensable tools across various disciplines, including chemistry, physics, biology, and environmental science. They are used in a wide range of applications from forensic analysis and medical diagnostics to space exploration and climate monitoring. The continuous evolution of spectrometer technology reflects our growing understanding of the intricate interactions between light and matter, underscoring the importance of historical developments in shaping our current capabilities. As we move forward, ongoing advancements promise even more precise and powerful instruments, further expanding our ability to analyze and understand the world around us. This historical context sets the stage for a deeper exploration into what a spectrometer is and how it functions, highlighting its critical role in modern scientific inquiry.
Importance in Various Fields
Spectrometers play a pivotal role across various fields, underscoring their versatility and importance in scientific inquiry and practical applications. In **chemistry**, spectrometers are indispensable for identifying and quantifying the chemical composition of substances. Techniques such as infrared (IR) spectroscopy and nuclear magnetic resonance (NMR) spectroscopy allow chemists to analyze molecular structures, which is crucial for drug development, forensic analysis, and environmental monitoring. For instance, IR spectroscopy can detect specific functional groups within molecules, while NMR provides detailed information about the molecular environment of atoms. In **physics**, spectrometers are essential tools for understanding the properties of light and matter. Optical spectrometers help physicists study the absorption and emission spectra of atoms and molecules, which reveal fundamental information about energy levels and transitions. This knowledge is vital for advancing our understanding of quantum mechanics and the behavior of materials under different conditions. Additionally, mass spectrometers are used in particle physics to analyze the mass-to-charge ratio of ions, aiding in the discovery of new particles and understanding subatomic interactions. **Biology** also heavily relies on spectrometric techniques. Mass spectrometry (MS) is a cornerstone in proteomics, enabling researchers to identify and quantify proteins within complex biological samples. This has significant implications for understanding disease mechanisms, developing biomarkers, and personalizing medicine. Furthermore, spectroscopic methods like fluorescence spectroscopy are used in molecular biology to study protein-ligand interactions and cellular processes, providing insights into biological pathways and potential therapeutic targets. In **environmental science**, spectrometers are crucial for monitoring and analyzing pollutants in air, water, and soil. Techniques such as atomic absorption spectroscopy (AAS) and inductively coupled plasma mass spectrometry (ICP-MS) allow scientists to detect trace amounts of heavy metals and other contaminants, which is essential for environmental regulation and public health protection. These methods also facilitate the study of climate change by analyzing isotopic ratios in ice cores and atmospheric samples. **Astronomy** benefits significantly from spectrometric analysis as well. Astronomical spectrometers help scientists study the light emitted or absorbed by celestial objects, providing valuable information about their composition, temperature, and motion. This data is critical for understanding the formation and evolution of stars, galaxies, and planetary systems. For example, spectroscopic observations can reveal the presence of exoplanets through the detection of subtle changes in a star's spectrum caused by planetary transits. **Medical diagnostics** also leverage spectrometric techniques to improve patient care. Raman spectroscopy, for instance, is being explored for its potential in non-invasive cancer diagnosis by analyzing the molecular signatures of tissues. Similarly, near-infrared spectroscopy (NIRS) is used to monitor oxygen levels in tissues, aiding in the diagnosis of conditions such as ischemia. In **industrial quality control**, spectrometers ensure the consistency and purity of products. For example, Fourier transform infrared (FTIR) spectroscopy is commonly used to analyze the chemical composition of materials in real-time, which is vital for maintaining quality standards in manufacturing processes. In summary, spectrometers are multifaceted instruments that contribute significantly to various scientific disciplines and practical applications. Their ability to provide detailed information about the composition and properties of substances makes them indispensable tools for advancing knowledge and solving real-world problems. Whether in chemistry, physics, biology, environmental science, astronomy, medical diagnostics, or industrial quality control, spectrometers play a critical role in driving innovation and understanding.
Types of Spectrometers
Spectrometers are sophisticated instruments that play a crucial role in various scientific fields by analyzing the interaction between matter and electromagnetic radiation or other forms of energy. These devices are essential for identifying and quantifying the chemical composition of substances, understanding molecular structures, and determining the presence of specific elements or compounds. The article delves into three primary types of spectrometers: Optical Spectrometers, Mass Spectrometers, and Nuclear Magnetic Resonance (NMR) Spectrometers. Each type offers unique capabilities and applications. Optical Spectrometers analyze the interaction between light and matter, providing insights into the chemical and physical properties of substances. Mass Spectrometers, on the other hand, measure the mass-to-charge ratio of ions, enabling detailed analysis of molecular structures and identification of unknown compounds. NMR Spectrometers utilize magnetic fields to study the nuclei of atoms, offering comprehensive information about molecular structures and dynamics. By exploring these different types of spectrometers, researchers and scientists can gain a deeper understanding of the world around them. This article begins by examining Optical Spectrometers, which are fundamental tools in many laboratories due to their versatility and wide range of applications.
Optical Spectrometers
Optical spectrometers are sophisticated instruments that play a crucial role in analyzing the interaction between light and matter, providing invaluable insights into the chemical and physical properties of substances. These devices are fundamental in various fields such as chemistry, physics, biology, and environmental science. At its core, an optical spectrometer measures the distribution of light intensity versus wavelength, which can reveal detailed information about the composition and structure of samples. There are several types of optical spectrometers, each tailored to specific applications and offering unique advantages. **Absorption Spectrometers**, for instance, measure the amount of light absorbed by a sample at different wavelengths. This is particularly useful in determining the concentration of substances in a solution, as seen in UV-Vis spectroscopy. **Emission Spectrometers**, on the other hand, analyze the light emitted by a sample when it is excited by an external energy source. This technique is often used in atomic emission spectroscopy to identify elements based on their characteristic emission lines. **Fluorescence Spectrometers** are another variant that detects the light emitted by a sample after it has absorbed light at a specific wavelength. This method is highly sensitive and is commonly used in biological research to study molecular interactions and dynamics. **Raman Spectrometers** utilize the Raman effect, where the inelastic scattering of light by molecules provides information about their vibrational modes. This technique is invaluable for identifying molecular structures and is widely used in materials science and pharmaceutical research. The design of an optical spectrometer typically includes several key components: a light source, a sample holder, a dispersing element (such as a prism or grating), and a detector. The light source can range from simple lamps to sophisticated lasers, depending on the application. The dispersing element separates the light into its component wavelengths, which are then detected by photodetectors such as photomultiplier tubes or charge-coupled devices (CCDs). Advanced spectrometers may also incorporate additional features like monochromators to enhance resolution and sensitivity. In addition to their analytical capabilities, optical spectrometers are also used in various industrial applications. For example, they are employed in quality control processes to ensure the purity and consistency of products. In environmental monitoring, spectrometers help track pollutants and monitor water and air quality. In medical diagnostics, they assist in identifying biomarkers for diseases and analyzing tissue samples. The advent of modern technologies has significantly enhanced the performance and accessibility of optical spectrometers. Advances in detector technology have improved sensitivity and speed, while computational methods have streamlined data analysis. Portable spectrometers now allow for on-site measurements, expanding their utility in fieldwork and remote sensing applications. In conclusion, optical spectrometers are powerful tools that leverage the principles of light-matter interaction to provide detailed insights into the properties of substances. Their versatility, coupled with ongoing technological advancements, ensures their continued importance across a broad spectrum of scientific and industrial disciplines. Whether used in research laboratories or industrial settings, these instruments remain indispensable for advancing our understanding of the world around us.
Mass Spectrometers
Mass spectrometers are sophisticated analytical instruments that play a crucial role in various scientific disciplines, including chemistry, biology, and physics. These devices are designed to measure the mass-to-charge ratio of ions, providing detailed information about the molecular composition of a sample. At the heart of a mass spectrometer lies its ability to ionize molecules, separate these ions based on their mass-to-charge ratio, and detect them with high precision. The process begins with ionization, where the sample is converted into ions. This can be achieved through various methods such as electron ionization (EI), chemical ionization (CI), or electrospray ionization (ESI), each suited for different types of samples. Once ionized, the ions are then accelerated and focused into a beam using electric fields. This beam is then directed into the mass analyzer, which is the core component of the mass spectrometer. There are several types of mass analyzers, each offering unique advantages. The quadrupole mass analyzer uses four parallel rods to filter ions based on their mass-to-charge ratio by applying specific electric fields. Time-of-flight (TOF) analyzers measure the time it takes for ions to travel through a field-free region, providing high resolution and sensitivity. Quadrupole time-of-flight (Q-TOF) analyzers combine the filtering capabilities of quadrupoles with the high resolution of TOF analyzers. Another type is the Orbitrap analyzer, which uses an electrostatic field to trap ions in a stable orbit around a central electrode, offering exceptional resolution and accuracy. After passing through the mass analyzer, the ions are detected by a detector that converts the ion signal into an electrical signal. This signal is then processed by sophisticated software to generate a mass spectrum, which is a plot of the intensity of the ions versus their mass-to-charge ratio. The resulting spectrum provides valuable information about the molecular weight, structure, and composition of the sample. Mass spectrometers have numerous applications across various fields. In pharmaceutical research, they are used to identify and quantify drug metabolites and impurities. In forensic science, they help in analyzing evidence such as hair samples, bloodstains, and explosives. In environmental science, mass spectrometers are employed to detect pollutants and contaminants in water and air samples. Additionally, they are indispensable tools in proteomics and genomics for identifying proteins and understanding biological pathways. The versatility and precision of mass spectrometers make them an essential tool in modern analytical chemistry. Continuous advancements in technology have improved their sensitivity, resolution, and speed, enabling researchers to tackle complex analytical challenges with greater ease. Whether it is identifying unknown compounds, quantifying trace amounts of substances, or elucidating complex biological processes, mass spectrometers stand at the forefront of analytical science, providing unparalleled insights into the molecular world.
Nuclear Magnetic Resonance (NMR) Spectrometers
Nuclear Magnetic Resonance (NMR) spectrometers are a cornerstone in the field of analytical chemistry, offering unparalleled insights into the molecular structure and dynamics of substances. These sophisticated instruments exploit the phenomenon of nuclear magnetic resonance, where nuclei of atoms with odd mass numbers (such as hydrogen-1, carbon-13, and nitrogen-15) interact with an external magnetic field and radiofrequency pulses. This interaction causes the nuclei to absorb and emit electromagnetic radiation, which is then detected and analyzed to provide detailed information about the molecular environment. At the heart of an NMR spectrometer lies a strong magnetic field, typically generated by a superconducting magnet. This field aligns the nuclear spins of the sample, creating a population imbalance that is essential for signal detection. The sample is placed in a probe, which is inserted into the magnet, and exposed to radiofrequency pulses that perturb the aligned spins. As these spins return to their equilibrium state, they emit signals that are captured by the probe and processed by sophisticated electronics. The data collected from NMR experiments can be interpreted in various ways, depending on the type of NMR technique employed. For instance, one-dimensional (1D) NMR spectra provide information about the chemical shifts and coupling constants of nuclei, which are crucial for identifying molecular structures. Two-dimensional (2D) NMR techniques, such as COSY and HSQC, offer more detailed insights by correlating different nuclei within a molecule, facilitating the assignment of complex structures. Advanced techniques like solid-state NMR and dynamic nuclear polarization (DNP) extend the capabilities of NMR to study solids and enhance sensitivity, respectively. NMR spectrometers are versatile tools that find applications across diverse fields. In organic chemistry, they are indispensable for structure elucidation and confirmation of synthetic compounds. In biochemistry, NMR is used to determine the three-dimensional structures of proteins and nucleic acids, providing insights into their functions and interactions. Pharmaceutical research relies heavily on NMR for drug discovery and development, while materials science utilizes NMR to study the properties of polymers and other materials. The evolution of NMR technology has led to significant improvements in sensitivity, resolution, and accessibility. Modern NMR spectrometers often feature cryogenically cooled probes that enhance signal-to-noise ratios, allowing for the analysis of smaller sample quantities. Automated systems and user-friendly software have made NMR more accessible to researchers without extensive training in the technique. Additionally, advancements in magnet design have enabled the development of benchtop NMR instruments, which offer a more compact and cost-effective alternative to traditional high-field systems. In summary, NMR spectrometers are powerful analytical tools that leverage the principles of nuclear magnetic resonance to provide detailed molecular information. Their applications span various scientific disciplines, and ongoing technological advancements continue to expand their capabilities and accessibility. As a result, NMR remains a cornerstone in modern scientific research, enabling scientists to delve deeper into the intricacies of molecular structures and dynamics.
Applications and Uses of Spectrometers
Spectrometers are versatile analytical instruments that have revolutionized various fields by providing precise and detailed information about the composition and properties of substances. These devices are instrumental in chemical analysis and identification, enabling scientists to determine the molecular structure and concentration of compounds. Beyond chemical analysis, spectrometers play a crucial role in biological and medical research, where they help in understanding biochemical processes, diagnosing diseases, and developing new treatments. Additionally, spectrometers are essential for environmental monitoring and quality control, allowing for the detection of pollutants and ensuring compliance with regulatory standards. By leveraging these capabilities, spectrometers contribute significantly to advancements in science, healthcare, and environmental protection. This article delves into these applications, starting with the foundational role of spectrometers in chemical analysis and identification.
Chemical Analysis and Identification
Chemical analysis and identification are pivotal in various scientific and industrial fields, and spectrometers play a crucial role in these processes. Spectrometers are sophisticated instruments that measure the interaction between matter and electromagnetic radiation, allowing for the detailed analysis of chemical compositions. This capability is essential in fields such as chemistry, biology, environmental science, and pharmaceuticals. For instance, in chemistry labs, spectrometers like mass spectrometers (MS) and nuclear magnetic resonance (NMR) spectrometers are used to identify unknown compounds by analyzing their molecular structure and mass-to-charge ratio. In environmental science, spectrometers help in monitoring pollutants by detecting specific wavelengths of light absorbed or emitted by contaminants in water or air samples. In pharmaceutical research, spectrometers aid in drug development by verifying the purity and structure of new compounds. Additionally, in forensic science, spectrometers are used to analyze evidence such as blood samples or fibers to help solve crimes. The versatility of spectrometers extends to food safety, where they can detect harmful additives or contaminants in food products. Furthermore, spectrometers are integral in medical diagnostics, enabling the identification of biomarkers for diseases through techniques like infrared spectroscopy. The precision and accuracy provided by spectrometers make them indispensable tools for chemical analysis and identification, facilitating advancements across multiple disciplines and ensuring the quality and safety of various products and environments. By leveraging the unique properties of different types of spectrometers—such as UV-Vis, IR, and Raman spectrometry—scientists can gain comprehensive insights into the chemical makeup of substances, thereby enhancing our understanding of complex systems and driving innovation in numerous fields.
Biological and Medical Research
In the realm of biological and medical research, spectrometers play a pivotal role in advancing our understanding of life processes and diagnosing diseases. These sophisticated instruments enable scientists to analyze the interaction between matter and electromagnetic radiation, providing invaluable insights into the molecular structure and composition of biological samples. For instance, mass spectrometers are crucial in proteomics, where they help identify and quantify proteins in complex biological mixtures. This capability is essential for understanding protein function, identifying biomarkers for diseases, and developing targeted therapies. In metabolomics, spectrometers such as NMR (Nuclear Magnetic Resonance) and GC-MS (Gas Chromatography-Mass Spectrometry) are used to study the metabolic profiles of cells, tissues, and organisms, which aids in the discovery of metabolic pathways and the diagnosis of metabolic disorders. Spectrometers also find extensive application in drug development. High-performance liquid chromatography (HPLC) coupled with mass spectrometry (LC-MS) is a powerful tool for analyzing the purity and efficacy of pharmaceutical compounds. This technique allows researchers to detect trace amounts of impurities and metabolites, ensuring the safety and potency of drugs before they reach clinical trials. Furthermore, infrared spectrometers are used in forensic medicine to analyze biological fluids and tissues, helping in the identification of substances such as narcotics and toxins. In clinical diagnostics, spectrometers contribute significantly to non-invasive or minimally invasive diagnostic techniques. For example, Raman spectroscopy can be used to analyze tissue samples for cancer diagnosis without the need for extensive tissue preparation or biopsy. This method provides real-time information about the biochemical composition of tissues, enabling early detection and monitoring of diseases like cancer and diabetes. Additionally, near-infrared spectroscopy (NIRS) is employed in neuroimaging to monitor brain activity and oxygenation levels, which is particularly useful in neurosurgical procedures and the study of neurological disorders. The versatility of spectrometers extends to environmental health studies as well. By analyzing water and air samples using techniques like atomic absorption spectroscopy (AAS) and inductively coupled plasma mass spectrometry (ICP-MS), researchers can detect trace levels of heavy metals and other pollutants, helping to monitor environmental health and enforce regulatory standards. This capability is critical for public health policy-making and ensuring the safety of ecosystems. In summary, spectrometers are indispensable tools in biological and medical research, offering a wide range of applications from basic research to clinical diagnostics. Their ability to provide detailed molecular information has revolutionized various fields, from drug development and disease diagnosis to environmental monitoring. As technology continues to evolve, the role of spectrometers will only become more pivotal in advancing our understanding of life sciences and improving human health outcomes.
Environmental Monitoring and Quality Control
Environmental monitoring and quality control are critical components in the preservation of our planet's health, and spectrometers play a pivotal role in these efforts. Spectrometers, by analyzing the interaction between matter and electromagnetic radiation, provide precise and detailed information about the chemical composition of various environmental samples. This capability is essential for monitoring air, water, and soil quality. For instance, spectrometers can detect pollutants such as heavy metals, volatile organic compounds (VOCs), and other harmful substances in water bodies, helping to ensure that drinking water meets safety standards. In air quality monitoring, spectrometers can identify and quantify pollutants like particulate matter, nitrogen oxides, and sulfur dioxide, which are key indicators of air pollution. This data is crucial for policymakers to implement effective regulations and for industries to adopt cleaner technologies. In soil monitoring, spectrometers help in assessing nutrient levels and detecting contaminants such as pesticides and industrial chemicals. This information is vital for sustainable agriculture practices and preventing soil degradation. Additionally, spectrometers are used in climate change research to measure greenhouse gases like carbon dioxide and methane, providing valuable insights into global warming trends. The data collected through spectrometric analysis also aids in the development of strategies for mitigating the effects of climate change. Moreover, spectrometers are integral in waste management and industrial processes. They help in monitoring emissions from industrial plants, ensuring compliance with environmental regulations. In waste management, spectrometers can analyze the composition of waste materials, facilitating the identification of recyclable materials and hazardous substances that need special handling. This not only reduces the environmental impact of waste disposal but also promotes resource recovery. The precision and reliability of spectrometric data make it a cornerstone in environmental research and policy-making. By providing accurate and real-time information, spectrometers enable scientists and policymakers to make informed decisions that protect public health and the environment. For example, during environmental disasters like oil spills or chemical leaks, spectrometers can quickly identify the types and quantities of pollutants involved, guiding cleanup efforts and minimizing ecological damage. In summary, the applications of spectrometers in environmental monitoring and quality control are diverse and indispensable. From ensuring the safety of our air, water, and soil to supporting sustainable practices and mitigating climate change, spectrometers are essential tools in the ongoing effort to protect our environment. Their ability to provide detailed chemical analyses makes them invaluable for both research and regulatory purposes, ultimately contributing to a healthier planet for future generations.