What Is The Fastest Thing In The Universe
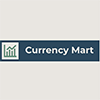
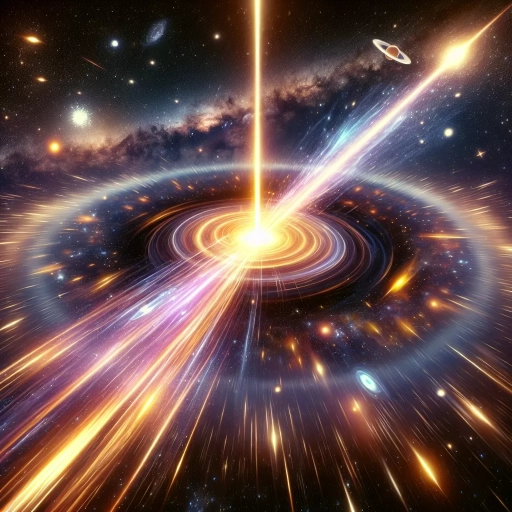
In the vast expanse of the universe, speed is a fundamental concept that has captivated human curiosity for centuries. The quest to identify the fastest thing in the universe leads us to explore several fascinating phenomena. At the heart of this inquiry lies the speed of light, which is widely recognized as the universal limit. However, other fast phenomena, such as high-energy particles and cosmic events, also warrant consideration. Additionally, theoretical limits and speculations about what could potentially exceed this speed offer intriguing insights into the nature of space and time. This article delves into these aspects, beginning with an in-depth examination of **The Speed of Light: The Universal Limit**, which sets the stage for understanding why it remains the benchmark against which all other speeds are measured.
The Speed of Light: The Universal Limit
The speed of light, a fundamental constant in the universe, stands as an unyielding limit that governs the behavior of all physical phenomena. This universal speed, approximately 299,792 kilometers per second, is not just a curiosity but a cornerstone of modern physics. Understanding its significance requires delving into three critical aspects: the definition and measurement of light speed, the physical implications it imposes, and its technological applications. The definition and measurement of light speed are crucial as they form the basis of our comprehension of this constant. Historically, scientists have employed various methods to measure it with increasing precision, from early experiments using rotating wheels to modern interferometry techniques. This precise measurement is essential for validating theories such as Einstein's theory of relativity. Moving forward, we will explore how the speed of light is defined and measured, laying the groundwork for understanding its profound physical implications and innovative technological applications. --- **Definition and Measurement of Light Speed**
Definition and Measurement of Light Speed
The speed of light, denoted by the letter \(c\), is a fundamental constant in physics that represents the maximum speed at which all massless particles and waves, including light, travel in a vacuum. This speed is approximately 299,792,458 meters per second (m/s) or about 186,282 miles per second. The definition of light speed is rooted in the theory of electromagnetism and is a cornerstone of Albert Einstein's theory of special relativity, where it serves as the universal limit for the speed of any object with mass. Measuring the speed of light has been a subject of scientific inquiry for centuries. Early attempts involved using astronomical observations, such as the timing of eclipses and the transit times of celestial bodies. However, these methods were not precise enough to yield accurate results. In the 17th century, Ole Rømer made significant strides by observing the eclipses of Jupiter's moons, noting that their timing varied depending on Earth's position relative to Jupiter. This led to an estimate of light speed that was remarkably close to the actual value. Modern measurements of light speed are far more precise and rely on sophisticated techniques. One method involves using interferometry, where light is split into two beams that travel different distances before being recombined to form an interference pattern. By carefully measuring the phase shift in this pattern, scientists can calculate the speed of light with high accuracy. Another approach uses time-of-flight measurements, where a pulse of light is sent over a known distance and the time taken for it to return is measured. This method has been refined to such an extent that it can determine the speed of light to within a fraction of a meter per second. The importance of accurately measuring the speed of light extends beyond mere curiosity; it underpins many areas of physics and engineering. For instance, in telecommunications, knowing the exact speed of light is crucial for designing fiber optic networks and satellite communications systems. In particle physics, precise measurements of light speed help in understanding the behavior of subatomic particles and the fundamental forces of nature. In conclusion, the speed of light is not just a fascinating constant but a critical component of our understanding of the universe. Its measurement has evolved significantly over time, from early astronomical observations to sophisticated laboratory techniques. As the universal limit for speed, it remains a cornerstone of modern physics, influencing fields ranging from telecommunications to particle physics.
Physical Implications of Light Speed
The physical implications of light speed are profound and far-reaching, underpinning many fundamental principles in physics. At approximately 299,792 kilometers per second, light speed (denoted as \(c\)) is the fastest speed at which any object or information can travel in a vacuum. This limit is not just a physical constraint but a cornerstone of Einstein's theory of special relativity. According to special relativity, as an object approaches the speed of light, its mass increases and time appears to slow down relative to an observer at a lower speed—a phenomenon known as time dilation. Additionally, the energy required to accelerate an object to higher speeds becomes exponentially greater as it approaches \(c\), making it impossible to reach or exceed light speed with any finite amount of energy. The speed of light also plays a crucial role in the structure of spacetime. In general relativity, Einstein described spacetime as a fabric that is warped by mass and energy. The speed of light is the maximum speed at which any disturbance in this fabric can propagate, influencing how we understand gravitational waves and black holes. For instance, the event horizon of a black hole marks the boundary beyond which nothing, including light, can escape once it falls inside. Furthermore, the speed of light has significant implications for communication and travel. Since no information can travel faster than light, there is a fundamental limit on how quickly we can communicate with distant objects or travel to other parts of the universe. This limit imposes severe constraints on interstellar travel and communication, making it virtually impossible to achieve real-time communication with distant stars or galaxies. In quantum mechanics, the speed of light is integral to understanding particle interactions and the behavior of photons. Photons are massless particles that always travel at the speed of light and are the quanta of electromagnetic radiation. Their behavior underlies many phenomena such as quantum entanglement and the photoelectric effect. In practical terms, the speed of light affects technological advancements in fields like telecommunications and computing. Fiber optic cables rely on light to transmit data at speeds close to \(c\), enabling high-speed internet connectivity over long distances. Similarly, in computing, the speed of light limits how quickly data can be transmitted within a computer system due to the physical constraints on signal propagation. In conclusion, the physical implications of light speed are multifaceted and pervasive across various domains of physics. It sets a universal limit that shapes our understanding of space, time, energy, and matter itself. Understanding these implications is crucial for advancing our knowledge of the universe and developing innovative technologies that push against these fundamental limits.
Technological Applications of Light Speed
The technological applications of light speed are vast and transformative, underpinning many modern technologies that shape our daily lives. At approximately 299,792 kilometers per second, light speed is the fastest speed at which any object or information can travel in a vacuum, making it a fundamental limit for communication and data transfer. In telecommunications, fiber optic cables rely on light to transmit data across long distances at speeds approaching this limit, enabling high-speed internet and global connectivity. This technology has revolutionized how we communicate, access information, and conduct business. In the realm of computing, light speed influences the design of high-performance computing systems. Optical interconnects within supercomputers use light to transfer data between processors, significantly reducing latency and increasing processing power. This advancement is crucial for complex simulations in fields like climate modeling, quantum physics, and artificial intelligence. Medical imaging technologies such as Optical Coherence Tomography (OCT) utilize light to create detailed images of internal structures without invasive procedures. By measuring the time delay of reflected light, OCT can produce high-resolution images of tissues, aiding in diagnostics and treatments for conditions like retinal diseases and cardiovascular disorders. Astronomy and space exploration also benefit from the properties of light. Telescopes use light from distant stars and galaxies to study the universe, while laser ranging techniques measure the distance to celestial bodies by timing the round-trip journey of laser pulses. These methods have significantly expanded our understanding of the cosmos and its evolution. Furthermore, quantum communication systems leverage the principles of quantum mechanics and light speed to secure data transmission. Quantum key distribution (QKD) protocols encode information onto photons, ensuring that any attempt to intercept the data would be detectable due to the no-cloning theorem. This technology promises unbreakable encryption for sensitive communications. In addition, the study of light speed has driven innovations in materials science. The development of metamaterials with negative refractive indices allows for the creation of optical devices that can manipulate light in unprecedented ways, such as perfect lenses and cloaking devices. These advancements hold potential for breakthroughs in fields ranging from energy harvesting to biomedical research. Lastly, the pursuit of achieving or approaching light speed has inspired significant investment in research and development across various disciplines. For instance, the concept of light-driven propulsion systems for spacecraft is being explored as a potential means to achieve faster-than-current travel times for interstellar missions. In summary, the technological applications of light speed are multifaceted and far-reaching, driving innovation in telecommunications, computing, medicine, astronomy, quantum communication, materials science, and space exploration. As our understanding of this universal limit continues to evolve, so too will the technologies that rely on it, promising even more transformative impacts on society in the future.
Other Fast Phenomena in the Universe
The universe is replete with phenomena that operate at incredible speeds, each offering unique insights into the fundamental laws of physics and the cosmos. High-speed particles in particle accelerators, for instance, allow scientists to probe the subatomic realm, revealing the intricate structure of matter and the forces that govern it. Fast-moving celestial objects like pulsars and quasars provide windows into extreme astrophysical environments, where gravity and energy are pushed to their limits. Additionally, the expanding universe and cosmic expansion underscore the dynamic nature of space itself, illustrating how galaxies and stars are constantly moving away from each other. These phenomena collectively highlight the universe's penchant for speed and energy, driving our understanding of both the microscopic and macroscopic scales. As we delve into these topics, we begin with the fascinating world of high-speed particles in particle accelerators, where human ingenuity meets the raw power of physics.
High-Speed Particles in Particle Accelerators
In the realm of particle accelerators, high-speed particles are accelerated to nearly the speed of light, showcasing some of the fastest phenomena in the universe. These accelerators, such as the Large Hadron Collider (LHC), are complex machines that use powerful magnetic fields and electric fields to propel subatomic particles like protons and electrons to incredible velocities. At the LHC, for instance, protons are accelerated to approximately 99.9999991% of the speed of light, reaching energies of up to 6.5 TeV (tera-electronvolts). This process involves a series of stages where particles are injected into a circular path and then accelerated by radiofrequency cavities that impart energy to the particles as they pass through. The high-speed collisions produced by these accelerators allow physicists to study the fundamental nature of matter and energy, recreating conditions similar to those in the early universe just after the Big Bang. These experiments have led to groundbreaking discoveries, including the detection of the Higgs boson, a particle crucial for understanding how other particles acquire mass. The technology behind particle accelerators is not only a marvel of engineering but also a key tool in advancing our understanding of the universe's most fundamental laws and phenomena. By pushing particles to such extreme speeds, scientists can explore the frontiers of physics, uncovering secrets that were previously inaccessible and shedding light on other fast phenomena observed throughout the cosmos.
Fast-Moving Celestial Objects like Pulsars and Quasars
Among the fastest-moving celestial objects in the universe are pulsars and quasars, which exhibit extraordinary velocities and energetic phenomena. **Pulsars**, incredibly dense neutron stars formed from the remnants of massive stars that have undergone supernova explosions, rotate at astonishing rates. Some pulsars can spin hundreds of times per second, emitting intense beams of electromagnetic radiation that sweep through space like cosmic lighthouses. These beams can travel at nearly the speed of light, making pulsars among the most precise natural clocks in the universe. Their rapid rotation and strong magnetic fields generate powerful electromagnetic pulses, which can be detected from vast distances, providing valuable insights into extreme astrophysical processes. **Quasars**, on the other hand, are incredibly luminous objects believed to be distant galaxies with supermassive black holes at their centers. These black holes actively accrete material, releasing enormous amounts of energy in the form of light and other forms of electromagnetic radiation. Quasars can outshine entire galaxies and are thought to be among the brightest objects in the universe. The jets of energetic particles ejected by quasars can move at significant fractions of the speed of light, sometimes exceeding 90% of this universal speed limit. These jets are powered by the gravitational energy released as matter falls toward the black hole, making quasars key objects for studying high-energy astrophysics and the early universe. Both pulsars and quasars offer unique windows into extreme astrophysical phenomena, allowing scientists to explore conditions that cannot be replicated in terrestrial laboratories. The study of these objects has significantly advanced our understanding of stellar evolution, black hole physics, and the behavior of matter under extreme conditions. Their fast-moving nature not only fascinates astronomers but also provides critical data for understanding some of the most fundamental questions about the universe's structure and evolution. As such, pulsars and quasars stand as compelling examples of other fast phenomena in the universe, highlighting the awe-inspiring scales and velocities that govern cosmic dynamics.
Expanding Universe and Cosmic Expansion
The expanding universe and cosmic expansion are fundamental concepts in modern astrophysics, offering insights into the dynamic nature of the cosmos. The universe's expansion was first observed by Edwin Hubble in the 1920s, who discovered that galaxies are moving away from each other at speeds proportional to their distances. This observation led to the Big Bang theory, which posits that the universe began as a single point and has been expanding ever since. The rate of this expansion is quantified by Hubble's constant, which, although subject to some uncertainty, provides a measure of how fast galaxies are receding from us. Cosmic expansion is not just about galaxies moving apart; it also involves the stretching of space itself. This means that the distance between any two points in the universe increases over time, a phenomenon that can be visualized by imagining dots on a balloon that inflate as the balloon expands. This expansion is thought to be driven by dark energy, a mysterious form of energy that permeates the universe and accelerates its expansion. The implications of cosmic expansion are profound. For instance, it suggests that there may be regions of the universe beyond our observable horizon, which are moving away from us faster than the speed of light due to the expansion of space. This raises intriguing questions about the ultimate fate of the universe, with possibilities ranging from a continued acceleration leading to a "big rip" to a deceleration that could result in a "big crunch." In the context of other fast phenomena in the universe, cosmic expansion stands out for its ubiquity and scale. While phenomena like supernovae explosions or gamma-ray bursts are incredibly fast and energetic on a local scale, cosmic expansion affects the entire fabric of space and time. It underscores the dynamic and evolving nature of the universe, highlighting that even the most distant galaxies are part of an ever-changing cosmic landscape. Understanding cosmic expansion also has practical implications for astronomy and cosmology. For example, it helps in calculating the ages of distant stars and galaxies by accounting for how much space has expanded since light left those objects. Additionally, studying cosmic expansion can provide insights into the fundamental laws of physics, particularly those related to gravity and the behavior of dark energy. In summary, the expanding universe and cosmic expansion represent a cornerstone of modern cosmology, revealing a universe in constant motion and evolution. This phenomenon not only explains the observed recession of galaxies but also offers a window into the universe's past, present, and future, making it one of the most fascinating and fast-paced aspects of the cosmos.
Theoretical Limits and Speculations
Theoretical limits and speculations in physics often push the boundaries of our understanding, delving into the realms of what is currently possible and what might be achievable in the future. This article explores three key areas that challenge our current knowledge: Theoretical Particles and Hypothetical Speeds, Quantum Mechanics and Potential Faster-than-Light Phenomena, and Speculative Theories on Faster-than-Light Travel. Each of these areas offers a unique perspective on how we might transcend the speed of light, a fundamental limit imposed by Einstein's theory of relativity. By examining theoretical particles that could potentially move at speeds greater than light, we delve into the hypothetical scenarios that these particles might enable. Additionally, quantum mechanics provides insights into phenomena that could potentially allow for faster-than-light communication or travel, albeit in highly speculative contexts. Finally, speculative theories on faster-than-light travel open up new avenues for thinking about interstellar travel and communication. This journey into the theoretical begins with an examination of Theoretical Particles and Hypothetical Speeds, where we explore the conceptual frameworks that underpin these extraordinary ideas.
Theoretical Particles and Hypothetical Speeds
Theoretical particles and hypothetical speeds are crucial components in the realm of physics, particularly when discussing the fastest things in the universe. At the forefront of these discussions are particles like tachyons, which are hypothetical particles proposed to travel faster than the speed of light. According to special relativity, nothing with mass can reach or exceed the speed of light, but tachyons are theorized to have imaginary mass and always move at speeds greater than light. However, their existence remains purely speculative as they have yet to be observed or proven experimentally. Another theoretical construct is the concept of quantum entanglement, where particles can become connected in such a way that their properties are correlated regardless of the distance between them. This phenomenon suggests that information can be transmitted between entangled particles instantaneously, potentially violating the speed limit imposed by special relativity. However, this does not imply physical movement but rather a non-local connection that challenges our understanding of space and time. In addition to these particles, hypothetical speeds are often discussed in the context of Alcubierre warp drives. Proposed by physicist Miguel Alcubierre, this theoretical model involves creating a region of space-time with negative mass-energy density. This "warp bubble" would cause space to contract in front of a spacecraft and expand behind it, effectively moving the spacecraft at faster-than-light speeds without violating relativity since the spacecraft itself is not moving faster than light within its local frame of reference. While intriguing, this concept remains highly speculative due to the enormous energy requirements and the unknown nature of negative mass-energy. Furthermore, certain theories in quantum mechanics and cosmology introduce concepts like quantum foam and inflationary epochs where space itself can expand at speeds greater than light. During the Big Bang's inflationary period, for instance, the universe expanded exponentially fast, with distances increasing at rates far exceeding light speed. However, this expansion is not about objects moving through space but rather about space itself expanding. In summary, while theoretical particles like tachyons and hypothetical speeds associated with concepts such as Alcubierre warp drives or quantum entanglement push the boundaries of our understanding of speed in the universe, they remain within the realm of speculation until empirical evidence supports these theories. The fastest thing we know with certainty is still light, but these theoretical constructs continue to inspire scientific inquiry and challenge our current understanding of physics.
Quantum Mechanics and Potential Faster-than-Light Phenomena
Quantum Mechanics, a fundamental theory in physics, delves into the behavior of matter and energy at the smallest scales. It introduces concepts such as wave-particle duality, superposition, and entanglement, which challenge classical notions of space and time. One of the most intriguing aspects of Quantum Mechanics is its potential implications for faster-than-light (FTL) phenomena. While the theory of relativity strictly prohibits objects with mass from reaching or exceeding the speed of light, certain quantum phenomena seem to defy this limit in subtle ways. For instance, quantum entanglement allows for instantaneous communication between particles regardless of their spatial separation. When something happens to one particle in an entangled pair, the effect is immediately observed in the other particle, even if they are separated by vast distances. This phenomenon appears to violate the speed of light limit but does not actually allow for information transfer faster than light; it merely correlates the states of particles in a way that transcends classical understanding. Another area of speculation involves quantum tunneling and the concept of quantum foam. Quantum tunneling allows particles to pass through barriers that would be classically insurmountable, potentially suggesting pathways for information or matter to move in ways that seem faster than light. However, these phenomena are still bound by the constraints of relativity and do not enable true FTL travel. Theoretical frameworks like Quantum Field Theory (QFT) and certain interpretations of String Theory also propose the existence of particles or entities that could potentially move at speeds greater than light. For example, tachyons in QFT are hypothetical particles that always move faster than light but are not directly observable and remain purely theoretical. Speculations about wormholes and Alcubierre drives further explore the possibility of FTL travel within the context of general relativity. Wormholes could theoretically connect two distant points in space-time, allowing for near-instant travel between them. The Alcubierre drive proposes creating a region of space-time with negative mass-energy density, which would cause space to contract in front of a spacecraft and expand behind it, effectively moving the spacecraft at faster-than-light speeds without violating relativity. However, these ideas are highly speculative and require further research to determine their feasibility. Theoretical limits imposed by our current understanding of physics suggest that achieving true FTL travel remains a distant dream, but the intriguing possibilities offered by Quantum Mechanics continue to inspire scientific investigation and philosophical debate about the nature of space, time, and the universe itself.
Speculative Theories on Faster-than-Light Travel
Speculative theories on faster-than-light (FTL) travel have long fascinated scientists and science fiction enthusiasts alike, as they challenge our current understanding of the universe's fundamental laws. One of the most popular concepts is **Alcubierre Warp Drive**, proposed by physicist Miguel Alcubierre in 1994. This theory involves creating a region of space-time with negative mass-energy density, which would cause space to contract in front of a spacecraft and expand behind it. This "warp bubble" would effectively move the spacecraft at faster-than-light speeds without violating Einstein's theory of relativity, as the spacecraft itself would not be moving faster than light within its local frame of reference. However, the energy requirements for such a feat are enormous, possibly exceeding the energy output of a star. Another speculative approach is **wormholes**, hypothetical shortcuts through space-time that could connect two distant points in the universe. If stable wormholes existed, they could potentially allow for near-instant travel between these points, effectively bypassing the speed of light. However, maintaining stability and navigating through such tunnels poses significant theoretical challenges, including the need for exotic matter with negative energy density. **Quantum entanglement** also offers a fascinating avenue for FTL communication and potentially travel. In quantum mechanics, entangled particles can instantaneously affect each other regardless of distance. While this phenomenon does not allow for physical transport of objects, it has sparked discussions about using entanglement for quantum communication that could transcend light speed limitations. However, the no-communication theorem in quantum mechanics strictly prohibits using entanglement for sending information faster than light, making it more of a theoretical curiosity than a practical means of FTL travel. **Folded space-time** or "folding" theories suggest that space-time could be manipulated in such a way that two distant points are brought closer together, allowing for faster travel between them. This concept is often depicted in science fiction but remains purely speculative within current scientific understanding. Lastly, **tachyons**, hypothetical particles that always travel faster than light, have been proposed in some theories. However, their existence is highly speculative and would require a significant revision of our current understanding of particle physics and relativity. In summary, while these speculative theories offer intriguing possibilities for FTL travel, they are still firmly in the realm of theoretical speculation and face significant scientific hurdles before they can be considered viable. The search for ways to circumvent the speed of light continues to inspire innovative thinking and research but remains one of the most challenging puzzles in modern physics.