What Is Thick Water
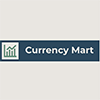
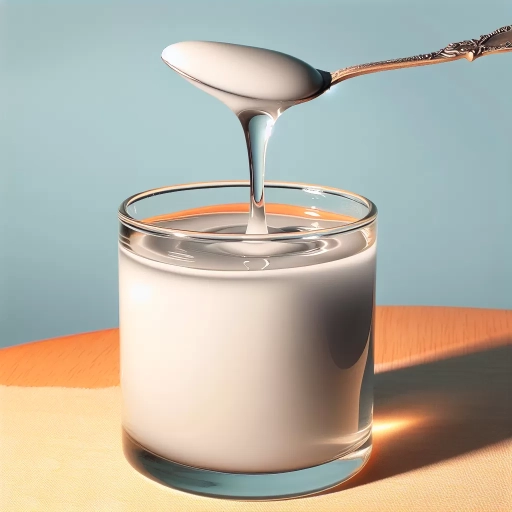
In the realm of scientific inquiry, the concept of "thick water" has garnered significant attention for its unique properties and potential applications. Thick water, also known as "non-Newtonian fluid" or "shear-thickening fluid," behaves in a manner that defies conventional expectations of liquid behavior. Unlike regular water, which maintains a consistent viscosity regardless of external forces, thick water becomes more viscous and even solid-like when subjected to stress or impact. This intriguing phenomenon has sparked extensive research into its underlying mechanisms, physical characteristics, and practical uses. To delve into the world of thick water, it is essential to first understand the concept itself, exploring how it differs from traditional fluids. Next, examining its properties and characteristics will reveal the intricate details that make it so fascinating. Finally, considering its applications and implications will highlight the potential impact on various fields, from materials science to safety engineering. By grasping these aspects, we can fully appreciate the significance of thick water and its role in advancing our understanding of fluid dynamics. Let us begin by understanding the concept of thick water.
Understanding the Concept of Thick Water
Understanding the concept of "thick water" requires a multifaceted approach, delving into its definition and origin, scientific basis, and historical context. At its core, "thick water" is a term that encapsulates the unique properties and behaviors of water under specific conditions, which set it apart from its more commonly understood liquid state. To grasp this concept fully, one must first explore its definition and origin, tracing back to how this term emerged and what it signifies in various scientific and cultural contexts. This foundational understanding will then pave the way for a deeper dive into the scientific basis of thick water, examining the physical and chemical principles that govern its behavior. Finally, considering the historical context in which this concept has evolved will provide a richer appreciation of its significance and relevance across different eras and disciplines. By examining these three dimensions, we can gain a comprehensive insight into what makes thick water such a fascinating subject of study. Let us begin by exploring the definition and origin of this intriguing term.
Definition and Origin
**Understanding the Concept of Thick Water** ### Definition and Origin The concept of "thick water" is a fascinating and relatively recent development in the field of materials science, particularly within the realm of non-Newtonian fluids. At its core, thick water refers to a type of fluid that exhibits properties unlike those of regular water. Unlike Newtonian fluids, which maintain a constant viscosity regardless of the force applied to them, thick water behaves as a non-Newtonian fluid, meaning its viscosity changes in response to the force or stress applied. The origin of thick water can be traced back to experiments involving the addition of certain polymers or nanoparticles to water. These additives alter the molecular structure of the fluid, creating a network of interconnected particles that resist flow when subjected to stress. This phenomenon is often observed in mixtures containing polyethylene oxide (PEO) or other high-molecular-weight polymers. When these polymers are dissolved in water, they form entangled networks that significantly increase the fluid's resistance to flow, thereby making it "thicker" under certain conditions. The term "thick water" was coined due to its unique ability to mimic the properties of both liquids and solids depending on the external forces applied. For instance, when subjected to low shear rates or gentle forces, thick water flows like regular water; however, when exposed to high shear rates or intense forces, it behaves more like a solid, exhibiting increased viscosity and even showing signs of elasticity. The discovery and study of thick water have been driven by various scientific and practical interests. Researchers are keen to understand how these fluids can be engineered for specific applications, such as in biomedical devices, where controlled viscosity could enhance drug delivery systems or improve the performance of medical implants. Additionally, understanding the mechanisms behind thick water's behavior can provide insights into natural phenomena involving complex fluids, such as blood flow in the human body or the movement of magma in geological processes. In summary, thick water is a non-Newtonian fluid created by adding specific polymers or nanoparticles to water, resulting in a fluid that changes viscosity based on applied stress. Its unique properties make it an intriguing subject for scientific study and potential practical applications across diverse fields. As research continues to unravel the complexities of thick water, it promises to open new avenues for innovation and problem-solving in various disciplines.
Scientific Basis
Understanding the concept of "thick water" requires a deep dive into its scientific basis, which is rooted in the principles of fluid dynamics and rheology. At its core, thick water refers to a non-Newtonian fluid that exhibits properties different from those of regular water due to the presence of additives or specific conditions. Unlike Newtonian fluids, which maintain a constant viscosity regardless of the force applied to them, non-Newtonian fluids change their viscosity in response to changes in shear rate or pressure. The scientific basis for thick water lies in the interaction between water molecules and the additives that alter its rheological behavior. For instance, when polymers or surfactants are added to water, they form complex networks that resist flow more effectively than pure water. This resistance is quantified by viscosity, a measure of a fluid's resistance to flow. In the case of thick water, the viscosity can increase significantly under certain conditions, making it behave more like a gel or a solid than a liquid. One key concept in understanding thick water is the Deborah number, a dimensionless quantity that compares the relaxation time of the fluid to the observation time. For thick water, this number is often high, indicating that the fluid's relaxation time is longer than the observation time, leading to its non-Newtonian behavior. This phenomenon is crucial in various industrial applications, such as in the production of personal care products like shampoos and lotions, where thick water provides the desired texture and stability. Another important aspect is the role of shear stress and shear rate. Shear stress is the force applied parallel or tangential to a face of a material, while shear rate is the rate of deformation due to this stress. In thick water, increasing the shear rate can lead to a decrease in viscosity—a property known as shear-thinning or pseudoplasticity. This characteristic makes thick water particularly useful in applications where it needs to flow easily under high shear conditions but maintain its thickness at rest. From a molecular perspective, the addition of polymers or surfactants introduces long-chain molecules that interact with each other and with water molecules. These interactions create a network structure that enhances the fluid's resistance to flow. The strength and complexity of this network determine the extent to which the fluid behaves as thick water. Advanced techniques such as rheometry and dynamic light scattering are used to study these interactions and quantify the resulting changes in viscosity and other rheological properties. In summary, the scientific basis of thick water is grounded in the principles of fluid dynamics and rheology, involving complex interactions between additives and water molecules that alter its viscosity and flow behavior. Understanding these underlying mechanisms is essential for harnessing the unique properties of thick water in various technological and industrial contexts. By delving into the molecular and macroscopic aspects of this phenomenon, researchers and engineers can develop innovative applications that leverage the distinctive characteristics of thick water.
Historical Context
Understanding the concept of "thick water" requires a deep dive into its historical context, which is intricately woven with the evolution of scientific thought and technological advancements. The term "thick water" is often associated with the early stages of understanding viscosity and fluid dynamics. Historically, the study of fluids dates back to ancient civilizations, where observations of natural phenomena like rivers and ocean currents sparked curiosity about the properties of water. In ancient Greece, philosophers such as Aristotle and Archimedes laid foundational groundwork by observing and theorizing about the behavior of fluids. Archimedes' principle, for instance, provided a mathematical framework for understanding buoyancy and density, which are crucial in comprehending why some waters appear "thicker" than others. However, it wasn't until the 17th and 18th centuries that significant strides were made in fluid dynamics. Scientists like Isaac Newton and Leonhard Euler developed laws that governed fluid motion, including viscosity—a key factor in what might be perceived as "thick water." The Industrial Revolution further accelerated research into fluid properties as engineers sought to optimize machinery performance and understand hydraulic systems. The work of Sir George Gabriel Stokes in the 19th century introduced the concept of kinematic viscosity, which quantifies a fluid's resistance to flow. This scientific progress enabled better design of pipelines, pumps, and turbines, all of which rely on precise understanding of fluid behavior. In modern times, advancements in materials science have led to the development of non-Newtonian fluids—substances whose viscosity changes under different shear rates or pressures. These materials can exhibit properties that might be described as "thick" under certain conditions but behave differently under others. For example, ketchup is a classic non-Newtonian fluid that appears thick when stationary but becomes more fluid when agitated. The historical context also includes cultural and practical applications. In traditional medicine and culinary practices across various cultures, substances with unique viscosities have been utilized for their perceived health benefits or culinary properties. For instance, honey has been valued for its thick consistency and medicinal properties since ancient times. Moreover, environmental studies have highlighted how changes in temperature and composition can affect the viscosity of natural bodies of water. Climate change research has shown that warming oceans can alter circulation patterns due to changes in water density and viscosity, impacting global weather patterns and marine ecosystems. In conclusion, understanding "thick water" is not just about recognizing its physical properties but also about appreciating the rich historical tapestry that has led to our current knowledge. From ancient philosophical musings to modern scientific breakthroughs, each era has contributed significantly to our comprehension of fluid dynamics and viscosity. This historical context not only enriches our understanding but also underscores the importance of continued research into the complex behaviors of fluids in various contexts.
Properties and Characteristics of Thick Water
Thick water, a term often used to describe non-Newtonian fluids or highly viscous liquids, exhibits a unique set of properties and characteristics that distinguish it from regular water. Understanding these properties is crucial for various applications in science, engineering, and everyday life. This article delves into the viscosity and density, chemical composition, and physical behavior of thick water, providing a comprehensive overview of its distinctive attributes. Viscosity and density are fundamental aspects that define the flow and resistance of thick water. Unlike regular water, which has a relatively low viscosity, thick water's high viscosity makes it more resistant to flow, affecting its handling and use in different contexts. The density of thick water can also vary significantly, impacting its buoyancy and mixing properties. The chemical composition of thick water is another critical factor. It often involves the presence of polymers, surfactants, or other additives that alter its rheological properties. These additives can enhance or modify the fluid's behavior under different conditions. Lastly, the physical behavior of thick water is characterized by its non-Newtonian flow, where the viscosity changes in response to shear rate or pressure. This behavior is essential for understanding how thick water interacts with surfaces and other materials. By examining these three key areas—viscosity and density, chemical composition, and physical behavior—we can gain a deeper understanding of the complex nature of thick water. Let's begin by exploring the critical role of viscosity and density in defining the properties of thick water.
Viscosity and Density
**Properties and Characteristics of Thick Water** ### Viscosity and Density Viscosity and density are two fundamental properties that define the behavior and characteristics of thick water, a term often used to describe water with enhanced viscosity due to the presence of additives or under specific conditions. **Viscosity**, a measure of a fluid's resistance to flow, plays a crucial role in determining how easily thick water can be poured, mixed, or pumped. Unlike regular water, which has a relatively low viscosity of about 0.89 centipoise (cP) at room temperature, thick water can exhibit viscosities several orders of magnitude higher. This increased viscosity can be achieved through the addition of polymers, gums, or other thickening agents that form complex networks within the water, thereby hindering the movement of molecules and increasing the fluid's resistance to flow. For instance, adding xanthan gum or guar gum can significantly enhance the viscosity of water, making it more suitable for applications where higher flow resistance is desired, such as in certain industrial processes or even in culinary preparations. **Density**, on the other hand, is the mass per unit volume of a substance and is typically measured in grams per cubic centimeter (g/cm³). While the density of pure water is approximately 1 g/cm³ at standard temperature and pressure, the density of thick water can vary depending on the type and amount of additives used. For example, if a thickening agent like cornstarch is added to water, it may slightly increase the overall density due to the higher molecular weight of the starch compared to water molecules. However, this increase is usually minimal unless large quantities of additives are used. The relationship between viscosity and density in thick water is complex; while increased viscosity does not directly affect density, the presence of thickening agents can alter both properties simultaneously. Understanding these interrelated properties is essential for predicting and controlling the behavior of thick water in various applications, from food science to engineering. In practical terms, the enhanced viscosity of thick water can significantly impact its handling and use. For instance, in cooking, thick water with high viscosity can be used as a sauce or dressing that maintains its consistency over time, unlike regular water-based sauces that might thin out quickly. In industrial settings, thick water can be used as a coolant or lubricant where higher flow resistance is beneficial. Additionally, the stability provided by increased viscosity makes thick water an excellent medium for suspending particles or emulsifying oils, which is crucial in formulations such as cosmetics or pharmaceuticals. In summary, the properties of viscosity and density are pivotal in defining the characteristics of thick water. By understanding and manipulating these properties through the addition of appropriate additives, it is possible to tailor thick water for a wide range of applications, enhancing its utility and performance in various fields. Whether it's improving the texture of food products or optimizing industrial processes, the unique properties of thick water make it a versatile and valuable resource.
Chemical Composition
Chemical composition is a fundamental aspect in understanding the properties and characteristics of thick water, a term often used to describe water with altered physical properties due to the presence of dissolved substances or additives. Thick water, also known as "thickened water" or "viscous water," typically contains polymers, gums, or other thickening agents that increase its viscosity. The chemical composition of thick water can vary widely depending on its intended use, such as in medical applications, food processing, or industrial processes. In medical contexts, thick water is often used to help individuals with dysphagia (swallowing disorders) by reducing the risk of aspiration. Here, the chemical composition might include xanthan gum, carboxymethylcellulose (CMC), or other hydrocolloids that are safe for human consumption. These polymers form a network of molecules that increase the fluid's viscosity, making it easier to swallow. The specific concentration of these additives can be adjusted to achieve different consistencies, ranging from nectar-thick to honey-thick or even pudding-thick. In food processing, thick water may be used as an ingredient in various products such as sauces, dressings, and beverages. The chemical composition in these cases might include natural thickeners like pectin from fruits or agar derived from seaweed. These substances not only enhance the texture but also contribute to the overall flavor profile and nutritional content of the product. For instance, pectin can add a fruity taste while providing dietary fiber. Industrial applications of thick water often involve more robust thickeners such as polyacrylamide or polyvinyl alcohol (PVA). These synthetic polymers are chosen for their high viscosity and stability under various conditions. In oil drilling operations, for example, thickened water can be used as a drilling fluid to improve lubrication and carry drill cuttings more efficiently. The chemical composition here must be carefully controlled to ensure compatibility with other drilling fluids and the geological environment. Understanding the chemical composition of thick water is crucial for predicting its behavior under different conditions. For instance, temperature changes can affect the solubility and performance of thickeners; some may degrade or lose their thickening properties at high temperatures. Similarly, pH levels can influence the stability of certain polymers, necessitating careful pH control in formulations. In summary, the chemical composition of thick water is a critical determinant of its properties and characteristics. By selecting appropriate thickeners and controlling their concentrations, thick water can be tailored for diverse applications ranging from medical care to industrial processes. This tailored approach ensures that thick water meets specific requirements for viscosity, stability, and safety across various contexts.
Physical Behavior
Physical behavior of thick water, a term often used to describe water under conditions where its viscosity and density are significantly altered, is a fascinating area of study. Unlike regular water, which has a viscosity of approximately 0.89 centipoise at room temperature, thick water exhibits enhanced resistance to flow. This alteration can be achieved through various means such as adding polymers or other substances that increase the molecular interactions within the water, thereby enhancing its viscosity. One of the most intriguing aspects of thick water is its non-Newtonian behavior. Unlike Newtonian fluids like regular water, whose viscosity remains constant regardless of the applied shear rate, thick water's viscosity changes in response to the force applied to it. For instance, when subjected to high shear rates, such as during vigorous stirring or pumping, thick water can exhibit a decrease in viscosity, a phenomenon known as shear-thinning. Conversely, under low shear rates or static conditions, its viscosity increases, making it behave more like a solid than a liquid. The physical behavior of thick water also impacts its surface tension and capillary action. With increased viscosity, the surface tension of thick water can be higher than that of regular water, affecting how it interacts with surfaces and other materials. This property is particularly relevant in applications such as industrial processes where fluid dynamics play a crucial role. Additionally, the enhanced viscosity can alter the rate of capillary action, which is the ability of water to flow through narrow spaces without the need for pressure. This can have significant implications for processes involving filtration and absorption. In terms of thermal properties, thick water generally retains the high specific heat capacity characteristic of regular water but may exhibit slightly different heat transfer rates due to its altered viscosity. This means that while it can still absorb and release heat efficiently, the rate at which this occurs might be slower compared to regular water. This aspect is important in applications where thermal management is critical, such as in cooling systems or thermal energy storage. The physical behavior of thick water also has practical implications in various fields. For example, in biomedical research, thick water can be used as a model fluid to simulate the behavior of biological fluids like blood or mucus, which exhibit non-Newtonian properties. In industrial settings, thick water can be used to improve the efficiency of mixing and pumping processes by reducing turbulence and energy consumption. Furthermore, its unique properties make it an interesting subject for studying fluid dynamics and rheology, providing insights into complex fluid behaviors that can be applied across multiple disciplines. In conclusion, the physical behavior of thick water is a complex and multifaceted topic that offers insights into the fundamental properties of fluids and their applications. Understanding these behaviors is crucial for optimizing various industrial and scientific processes, as well as for advancing our knowledge of fluid dynamics and rheology. The unique characteristics of thick water make it an invaluable tool for research and development, highlighting the importance of continued exploration into its properties and potential uses.
Applications and Implications of Thick Water
The concept of "thick water" refers to a unique state of water that exhibits enhanced viscosity and stability, making it an intriguing subject for various scientific and industrial applications. This article delves into the multifaceted implications of thick water, exploring its industrial uses, environmental impact, and future research directions. In the realm of industrial uses, thick water's distinct properties make it a valuable resource for improving processes in fields such as materials science and biotechnology. The environmental impact of thick water is also significant, as it can influence ecosystems and water cycles in profound ways. Furthermore, ongoing and future research into thick water holds the potential to uncover new applications and deepen our understanding of its behavior. By examining these aspects, we can better appreciate the versatility and importance of thick water. Let us begin by exploring how thick water is being harnessed in industrial settings, where its unique characteristics are revolutionizing manufacturing and production processes.
Industrial Uses
Industrial uses of thick water, also known as heavy water, are diverse and critical in various sectors due to its unique properties. One of the most significant applications is in nuclear reactors. Heavy water serves as an excellent neutron moderator and coolant because it has a higher neutron scattering cross-section compared to light water, allowing for more efficient nuclear reactions. This makes it particularly valuable in pressurized heavy water reactors (PHWRs) and other types of nuclear power plants, enhancing safety and efficiency by reducing the risk of overheating and improving fuel utilization. In addition to its role in nuclear energy, heavy water is crucial in the production of deuterium, a key component in the synthesis of heavy hydrogen isotopes. Deuterium is used in the manufacture of deuterated compounds, which are essential in chemical research and pharmaceuticals. For instance, deuterated solvents are used in NMR spectroscopy to analyze the structure of molecules, while deuterated drugs can offer enhanced stability and bioavailability. Heavy water also finds extensive use in the field of materials science and engineering. It is used as a coolant in high-energy particle accelerators and as a neutron shield to protect against radiation exposure. In the petrochemical industry, heavy water is employed in the production of ammonia through the Haber-Bosch process, where it helps in the synthesis of hydrogen gas. Moreover, heavy water has significant implications for environmental monitoring and research. It is used as a tracer in hydrological studies to understand water cycles and groundwater flow patterns. This helps scientists to better manage water resources, predict water quality changes, and assess the impact of human activities on aquatic ecosystems. In the realm of medical research, heavy water is utilized in various studies related to metabolism and cellular processes. It can be used to label biological molecules without altering their chemical properties, allowing researchers to track metabolic pathways and understand disease mechanisms more accurately. The industrial applications of heavy water underscore its importance as a versatile and critical resource. However, its production is energy-intensive and costly, making it a valuable commodity that requires careful management and optimization. As technology advances and new applications emerge, the demand for heavy water is likely to increase, highlighting the need for sustainable production methods and efficient use strategies to ensure its availability for future generations. Overall, the industrial uses of thick water are multifaceted and play a vital role in several key sectors, from energy production and chemical synthesis to environmental science and medical research. Its unique properties make it an indispensable tool in various applications, contributing significantly to technological advancements and scientific understanding.
Environmental Impact
The environmental impact of thick water, a term often used to describe seawater with high salinity or brine solutions, is multifaceted and significant. In natural ecosystems, thick water can have profound effects on marine life. For instance, brine pools on the ocean floor, which are essentially lakes of thick water, support unique microbial communities that thrive in these extreme environments. However, these ecosystems are also highly sensitive to changes in salinity and temperature, making them vulnerable to climate change and human activities such as deep-sea mining or drilling. In coastal areas, the discharge of thick water from industrial processes like desalination plants or oil and gas operations can disrupt local marine habitats. High salinity levels can be toxic to many marine species, leading to reduced biodiversity and altered food webs. Moreover, the increased density of thick water can lead to stratification in water bodies, reducing oxygen levels and exacerbating conditions like "dead zones" where life cannot thrive. On the other hand, thick water has several beneficial applications that can mitigate environmental impacts. For example, in geothermal energy production, thick brine solutions are used to extract heat from underground reservoirs, providing a renewable energy source with minimal greenhouse gas emissions. Additionally, thick water can be utilized in carbon capture and storage technologies by injecting CO2 into deep saline aquifers, helping to reduce atmospheric carbon dioxide levels. In agriculture, thick water can be used for irrigation in arid regions through advanced desalination techniques that make it economically viable. This not only supports food security but also helps in conserving freshwater resources for other uses. However, it is crucial to manage these systems sustainably to avoid over-extraction of groundwater and prevent soil salinization. From a broader perspective, understanding and managing the environmental impact of thick water is essential for sustainable development. This involves rigorous monitoring of industrial activities, implementing stringent regulations to prevent pollution, and investing in research to develop more efficient and environmentally friendly technologies. By balancing the applications and implications of thick water, we can harness its benefits while protecting our planet's delicate ecosystems. In conclusion, the environmental impact of thick water underscores the need for careful management and innovative solutions. As we continue to explore and utilize this resource, it is imperative that we do so with a deep understanding of its ecological implications and a commitment to sustainability. This approach will ensure that the benefits of thick water are realized without compromising the health of our planet.
Future Research Directions
Future research directions in the field of thick water, a novel and intriguing concept, are multifaceted and promising. As scientists continue to unravel the mysteries of this unique state of water, several key areas are emerging as focal points for further investigation. One primary direction involves the exploration of the structural and dynamical properties of thick water at the molecular level. Advanced spectroscopic techniques, such as nuclear magnetic resonance (NMR) and infrared spectroscopy, will be crucial in elucidating the hydrogen bonding networks and molecular arrangements that distinguish thick water from its more familiar liquid and solid forms. This fundamental understanding will not only shed light on the underlying mechanisms but also pave the way for tailored applications in fields like materials science and biotechnology. Another significant research avenue lies in the study of thick water's interactions with biological systems. Given its potential to influence protein folding, enzyme activity, and cellular hydration, thorough investigations into how thick water affects biological processes are essential. This could involve in vitro experiments using model proteins and cells, as well as in vivo studies to observe the effects on living organisms. Such research could have profound implications for our understanding of disease mechanisms and the development of novel therapeutic strategies. Furthermore, the technological applications of thick water are vast and varied. Researchers are keenly interested in exploiting its unique properties for innovative technologies such as advanced desalination methods, more efficient drug delivery systems, and enhanced agricultural practices. For instance, thick water's ability to retain solutes could be leveraged to create more effective filtration membranes or to design novel drug carriers that release therapeutic agents in a controlled manner. Additionally, its potential impact on plant hydration and nutrient uptake suggests that thick water could play a role in improving crop yields and resilience to environmental stressors. The environmental implications of thick water also warrant detailed study. Understanding how this state of water interacts with pollutants and contaminants could lead to breakthroughs in water purification technologies. Moreover, investigating the role of thick water in natural ecosystems—such as its presence in soil, sediments, or atmospheric aerosols—could provide insights into global water cycles and climate dynamics. This knowledge could inform policies aimed at managing water resources sustainably and mitigating the effects of climate change. Finally, interdisciplinary collaborations between physicists, chemists, biologists, and engineers will be indispensable for advancing our knowledge of thick water. The integration of theoretical models with experimental data will help in predicting and validating the behavior of thick water under various conditions. Computational simulations, such as molecular dynamics and quantum mechanics/molecular mechanics (QM/MM) methods, will be particularly valuable in this regard, allowing researchers to model complex systems that are challenging to study experimentally. In conclusion, the future research directions in the field of thick water are rich with potential for groundbreaking discoveries and practical applications. By delving deeper into its molecular structure, biological interactions, technological uses, environmental impact, and through interdisciplinary collaboration, scientists can unlock the full promise of this fascinating state of matter. As our understanding of thick water evolves, so too will its contributions to diverse fields, ultimately leading to innovative solutions that benefit society and the environment.