What Is Disruptive Selection
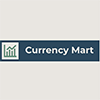
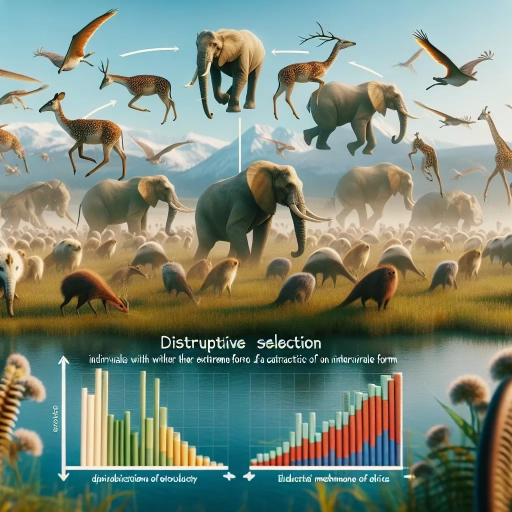
Disruptive selection, a fascinating and complex evolutionary process, plays a crucial role in shaping the diversity of life on Earth. Unlike stabilizing selection, which favors intermediate traits, disruptive selection acts to increase the frequency of extreme phenotypes within a population. This unique mechanism can lead to the emergence of new species and significant changes in population dynamics. In this article, we will delve into the intricacies of disruptive selection, exploring its underlying principles and how it operates. We will examine real-world examples and case studies that illustrate its impact, highlighting instances where disruptive selection has driven evolutionary change. Additionally, we will discuss the broader implications and consequences of this process, considering how it influences ecological balance and biodiversity. By understanding the mechanisms of disruptive selection, we can gain a deeper insight into the evolutionary forces that shape our natural world. Let us begin by **Understanding Disruptive Selection**, the foundational concept that underpins this compelling phenomenon.
Understanding Disruptive Selection
Understanding Disruptive Selection is a crucial concept in evolutionary biology, offering insights into how populations adapt and diversify under specific environmental pressures. This phenomenon is distinct from other forms of natural selection and has a rich historical context that underscores its significance. To fully grasp disruptive selection, it is essential to delve into its definition and mechanism, which involves the favoring of extreme traits over intermediate ones, leading to the formation of distinct subpopulations. Additionally, comparing disruptive selection with other forms of selection, such as stabilizing and directional selection, highlights its unique role in driving genetic diversity. The historical context and discovery of disruptive selection also provide valuable perspectives on how scientists have come to understand this complex process. By exploring these aspects, we can gain a comprehensive understanding of how disruptive selection shapes the evolution of species. Let us begin by examining the definition and mechanism of disruptive selection, which forms the foundational understanding of this intriguing evolutionary process.
Definition and Mechanism
**Understanding Disruptive Selection: Definition and Mechanism** Disruptive selection, a pivotal concept in evolutionary biology, refers to the process where natural selection favors individuals with extreme traits over those with intermediate traits. This mechanism stands in contrast to stabilizing selection, which prefers individuals with average characteristics. To grasp the essence of disruptive selection, it is crucial to delve into its definition and underlying mechanisms. **Definition:** Disruptive selection occurs when the environment or ecological niches favor individuals at both ends of a trait spectrum, leading to the survival and reproduction of those with either very high or very low values of a particular trait. This form of selection can result in the divergence of populations into distinct groups, each specializing in different ecological niches. **Mechanism:** The mechanism of disruptive selection involves several key steps. First, a population must exhibit genetic variation in the trait of interest. This variation can arise from mutations, genetic drift, or gene flow. Next, the environment must impose selective pressures that differentially affect individuals based on their trait values. For instance, in a scenario where food resources are scarce and competition is high, individuals with either very large or very small beak sizes might have an advantage over those with average-sized beaks because they can exploit different food sources more efficiently. As disruptive selection acts on these traits, it leads to an increase in the frequency of extreme phenotypes within the population. Over time, this can result in the formation of distinct subpopulations or even speciation if the selected traits are heritable and if there is sufficient genetic divergence between the groups. For example, in the case of the Galapagos finches, disruptive selection has been observed where finches with larger beaks can crack tough seeds while those with smaller beaks can eat smaller seeds and insects, thus occupying different ecological niches. Moreover, disruptive selection can be influenced by various factors such as predation pressure, resource availability, and social interactions. For instance, in some species, disruptive selection may be driven by predator-prey dynamics where predators target intermediate-sized prey more frequently than extreme-sized ones. This selective pressure would then favor the survival of individuals at both ends of the size spectrum. In summary, disruptive selection is a powerful evolutionary force that drives the diversification of populations by favoring extreme traits over intermediate ones. Its mechanism hinges on genetic variation, differential selection pressures, and ecological specialization. Understanding this process provides insights into how species adapt to their environments and how new species may emerge through evolutionary divergence. By recognizing the role of disruptive selection in shaping biodiversity, we gain a deeper appreciation for the complex dynamics that underpin the natural world.
Comparison with Other Forms of Selection
Disruptive selection stands out distinctly when compared to other forms of natural selection, such as stabilizing and directional selection. While stabilizing selection favors individuals with average traits, thereby reducing variation within a population, disruptive selection does the opposite. It promotes the survival and reproduction of individuals with extreme traits, leading to an increase in genetic diversity. This contrasts sharply with directional selection, which drives the evolution of a population towards one extreme of a trait spectrum. For instance, in a scenario where a population of birds is subjected to disruptive selection based on beak size, birds with either very large or very small beaks are more likely to survive and reproduce compared to those with average-sized beaks. This would result in two distinct subpopulations emerging over time, each adapted to different ecological niches. In contrast, stabilizing selection would favor birds with beak sizes close to the mean, reducing the variability in beak size within the population. Directional selection, on the other hand, might favor birds with larger beaks if larger beaks confer an advantage in foraging or other survival tasks. The unique aspect of disruptive selection is its ability to create and maintain polymorphism within a population, allowing for the coexistence of multiple phenotypes that are better suited to different environmental conditions. This form of selection is particularly relevant in environments where there are multiple resources or niches available, as it enables populations to exploit these resources more effectively by diversifying their traits. Moreover, disruptive selection can play a crucial role in speciation processes. By driving the formation of distinct subpopulations with different traits, it can lead to reproductive isolation and eventually the emergence of new species. This is because the extreme traits favored by disruptive selection can lead to differences in mating behaviors or other reproductive barriers between the subpopulations. For example, if the birds with large beaks and those with small beaks begin to mate preferentially within their respective groups due to differences in foraging habits or other behaviors associated with beak size, this could eventually lead to genetic divergence and speciation. Understanding disruptive selection is essential for grasping how populations adapt and evolve in complex environments where multiple selective pressures are at play. It highlights the dynamic nature of evolutionary processes and how different forms of selection can shape the diversity and adaptability of species in unique ways. By recognizing the role of disruptive selection alongside other forms of natural selection, scientists can gain a more comprehensive understanding of the mechanisms driving evolutionary change and the maintenance of biodiversity in natural ecosystems.
Historical Context and Discovery
**Historical Context and Discovery** The concept of disruptive selection, a key mechanism driving evolutionary change, has its roots in the early 20th century when geneticists and evolutionary biologists began to unravel the mysteries of natural selection. The term "disruptive selection" was first coined by the British geneticist and evolutionary biologist Ronald Fisher in his seminal work "The Genetical Theory of Natural Selection" (1930). Fisher's groundbreaking book laid the foundation for modern evolutionary theory, introducing the idea that natural selection could act in various modes, including stabilizing, directional, and disruptive selection. In the context of disruptive selection, Fisher proposed that this mode of selection favors extreme phenotypes over intermediate ones, leading to the formation of distinct subpopulations within a species. This contrasts with stabilizing selection, which favors average phenotypes, and directional selection, which drives the population towards one extreme. The historical significance of Fisher's work lies in its integration of genetics and evolutionary theory, providing a mechanistic understanding of how populations evolve over time. The discovery and subsequent development of disruptive selection as a concept were further enriched by the contributions of other scientists. For instance, the American geneticist Sewall Wright's work on genetic drift and the shifting balance theory (1931) complemented Fisher's ideas by highlighting the role of random genetic drift in population dynamics. Wright's theory suggested that genetic drift could lead to the fixation of alleles that might initially be disadvantageous but later become beneficial under different environmental conditions, thereby facilitating disruptive selection. The empirical evidence supporting disruptive selection has been bolstered by numerous studies across various species. One of the most compelling examples comes from the work of Peter Grant and Rosemary Grant on the Galapagos finches. Their long-term research demonstrated how environmental changes, such as droughts, could lead to disruptive selection favoring finches with either larger or smaller beaks, depending on the availability of food resources. This real-world example illustrates how disruptive selection can act rapidly in response to changing environmental pressures. In addition to these empirical studies, advances in molecular biology and genomics have provided new tools for understanding the genetic basis of disruptive selection. By analyzing genetic variation within populations, scientists can identify specific loci under selection and trace the evolutionary history of these genes. This integration of molecular data with ecological and behavioral observations has significantly enhanced our understanding of how disruptive selection shapes the evolution of species. In summary, the historical context and discovery of disruptive selection are deeply intertwined with the development of modern evolutionary theory. From Fisher's initial conceptualization to the empirical validations by scientists like the Grants, and the ongoing contributions from molecular biology, our understanding of disruptive selection has evolved significantly. This mode of selection remains a crucial aspect of evolutionary biology, highlighting the complex and dynamic nature of species adaptation and diversification. As we continue to explore the intricacies of evolutionary processes, the concept of disruptive selection stands as a testament to the power of interdisciplinary research in uncovering the mechanisms that shape life on Earth.
Examples and Case Studies of Disruptive Selection
Disruptive selection, a powerful evolutionary force, shapes the diversity of life by favoring extreme traits over intermediate ones. This phenomenon is not merely a theoretical concept but is observed and studied in various contexts, each offering unique insights into its mechanisms and impacts. In the realm of natural examples, wildlife populations provide compelling evidence of disruptive selection in action. For instance, the peppered moth's shift from light to dark coloration in response to industrial pollution is a classic example. Additionally, laboratory studies have been instrumental in experimentally demonstrating the effects of disruptive selection, allowing scientists to control and manipulate environmental pressures to observe evolutionary outcomes. Furthermore, human activities have inadvertently driven disruptive selection in many species, illustrating the profound impact of anthropogenic factors on evolutionary trajectories. By examining these natural examples, experimental evidence, and human-induced changes, we can gain a comprehensive understanding of how disruptive selection operates across different scales and environments. Let us begin by delving into the natural examples in wildlife populations, where the intricate dance between environment and genetics is vividly illustrated.
Natural Examples in Wildlife Populations
Disruptive selection, a process where natural selection favors extreme traits over intermediate ones, is vividly illustrated through various examples in wildlife populations. One of the most compelling examples is the peppered moth in England. Prior to the Industrial Revolution, these moths were predominantly light-colored, allowing them to blend seamlessly with the lichen-covered tree bark they rested on. However, with the increase in air pollution, tree trunks became darker due to soot deposits. This environmental shift led to a dramatic change in the moth population; a genetic variation causing dark-colored moths became more prevalent because these individuals were better camouflaged on the dark tree trunks and thus less likely to be preyed upon by birds. Conversely, light-colored moths were more visible and vulnerable to predation. This scenario exemplifies disruptive selection where both light and dark traits were favored over intermediate shades, leading to an increase in genetic diversity within the population. Another illustrative case is the finches on the Galapagos Islands. Peter and Rosemary Grant's extensive research on these finches revealed how different beak sizes and shapes are favored under varying environmental conditions. During periods of drought, seeds become scarce and harder to crack open. In such times, finches with larger beaks have an advantage as they can crack open tougher seeds that other finches cannot access. Conversely, during wet seasons when seeds are abundant and softer, smaller-beaked finches thrive because they can exploit these resources more efficiently. This oscillation between favoring larger and smaller beaks disrupts the intermediate beak sizes, highlighting how disruptive selection can drive evolutionary change based on environmental pressures. The African elephant provides another striking example of disruptive selection in action. In some populations, there is a noticeable split between elephants with large tusks and those with smaller or no tusks at all. Large-tusked elephants have an advantage in terms of fighting for mates and defending against predators but are also more vulnerable to poaching due to their valuable ivory. On the other hand, elephants without tusks avoid this risk but may face other challenges such as reduced social status or difficulty in defending themselves. This dichotomy illustrates how disruptive selection can act on a population where both extremes (large tusks and no tusks) are favored over intermediate tusk sizes. In addition to these examples, the guppy fish in Trinidadian streams offer a fascinating study on coloration and predation pressure. Male guppies exhibit vibrant colors which serve as a signal for attracting mates but also make them more visible to predators like the pike cichlid. In streams with high predation pressure, duller-colored males are favored because they are less conspicuous to predators. However, in streams with low predation pressure, brightly colored males have an advantage as they can attract more mates without significant risk. This dynamic demonstrates how disruptive selection can lead to the coexistence of different traits within a population based on varying environmental conditions. These natural examples underscore the complex and dynamic nature of disruptive selection in wildlife populations. By favoring extreme traits over intermediate ones under specific environmental conditions, disruptive selection plays a crucial role in maintaining genetic diversity and driving evolutionary change. These case studies not only illustrate the mechanisms behind this form of natural selection but also highlight its significance in shaping the adaptability and resilience of species in diverse ecosystems.
Experimental Evidence from Laboratory Studies
Experimental evidence from laboratory studies has significantly contributed to our understanding of disruptive selection, a process where natural selection favors extreme phenotypes over intermediate ones. These controlled environments allow scientists to manipulate variables and observe the effects of disruptive selection in a more precise manner than field studies. For instance, in the laboratory, researchers can create populations with specific genetic traits and then apply selective pressures to see how these traits evolve over generations. One seminal study involved the fruit fly *Drosophila melanogaster*. By manipulating the environment to favor either larger or smaller body sizes, scientists observed that disruptive selection led to the evolution of bimodal distributions in body size, where intermediate sizes were less common. This was achieved by providing food sources that were either very large or very small, making it difficult for flies of average size to survive and reproduce effectively. Over several generations, this selective pressure resulted in the emergence of distinct morphs that were better adapted to their respective food sources. Another example comes from experiments with the bacterium *Escherichia coli*. In these studies, researchers subjected bacterial populations to varying levels of antibiotic resistance. By creating an environment where only bacteria with high or low resistance could survive, they observed that disruptive selection drove the evolution of distinct strains with extreme resistance levels. This was evident from the bimodal distribution of resistance phenotypes in the population after several rounds of selection. Laboratory studies also highlight the role of genetic mechanisms underlying disruptive selection. For example, experiments involving the yeast *Saccharomyces cerevisiae* have shown how genetic variation can be maintained through mechanisms such as heterozygote advantage or frequency-dependent selection. These studies demonstrate that disruptive selection can act on multiple genetic loci simultaneously, leading to complex evolutionary outcomes. Moreover, laboratory experiments have allowed researchers to explore the interaction between disruptive selection and other evolutionary forces like genetic drift and gene flow. For instance, simulations using digital organisms have shown that even in the presence of genetic drift, disruptive selection can still lead to the evolution of distinct morphs if the selective pressures are strong enough. In summary, laboratory studies provide robust experimental evidence for disruptive selection by allowing precise control over environmental conditions and genetic makeup. These controlled experiments have elucidated how disruptive selection operates at various levels—from individual traits like body size in fruit flies to complex genetic adaptations in bacteria—and have shed light on the underlying genetic mechanisms that drive this process. By replicating these findings across different organisms and environments, scientists can confidently assert that disruptive selection is a significant force shaping evolutionary diversity in natural populations.
Human Impact and Anthropogenic Disruptive Selection
Human impact on the natural world has significantly altered the evolutionary trajectories of numerous species, often leading to a phenomenon known as anthropogenic disruptive selection. This form of selection occurs when human activities create or exacerbate environmental pressures that favor extreme traits over intermediate ones, driving populations towards divergent phenotypes. A compelling example is the peppered moth in England. Prior to the Industrial Revolution, the moth population was predominantly light-colored, allowing them to blend in with lichen-covered tree bark. However, as industrial pollution darkened tree trunks with soot, a genetic variation in the moth population that resulted in dark-colored individuals became more prevalent. This shift was not due to natural selection alone but was accelerated by human-induced changes in the environment, illustrating how anthropogenic factors can disrupt natural selection processes. Another case study involves the evolution of finches on the Galapagos Islands. Human activities such as habitat destruction and the introduction of invasive species have altered the availability of food resources, leading to changes in beak morphology among finch populations. For instance, the large ground finch has evolved larger beaks in response to increased competition for seeds, a resource that has become more limited due to human activities. This example highlights how human impacts can create new selective pressures that drive disruptive selection, favoring extreme traits that are better suited to the altered environment. In marine ecosystems, overfishing has led to disruptive selection in fish populations. For example, the Atlantic cod has experienced a shift towards earlier maturation and smaller size at maturity due to the selective removal of larger, older individuals by commercial fisheries. This change is not only a response to natural predation but also a direct result of human fishing practices that target larger fish, thereby altering the demographic structure of cod populations and favoring individuals with traits that allow them to reproduce before being caught. Additionally, urbanization has been shown to induce disruptive selection in various species. The common frog, for instance, has adapted to urban environments by developing traits such as earlier breeding times and altered vocalizations to cope with increased noise pollution. These adaptations are not merely responses to natural environmental changes but are driven by the specific challenges posed by human urban development. In agricultural settings, the use of pesticides and herbicides has led to the evolution of pesticide-resistant pests and weeds. For example, the diamondback moth has developed resistance to Bacillus thuringiensis (Bt) toxin, which is widely used in genetically modified crops. This resistance is a clear example of disruptive selection where human agricultural practices have created strong selective pressures favoring individuals with resistant traits over those without. These examples underscore the profound impact of human activities on evolutionary processes. By altering environments and creating new selective pressures, humans are driving disruptive selection in various species, leading to rapid evolutionary changes that might not have occurred otherwise. Understanding these dynamics is crucial for managing ecosystems and conserving biodiversity in the face of increasing anthropogenic influences.
Implications and Consequences of Disruptive Selection
Disruptive selection, a powerful evolutionary force, has profound implications and consequences that resonate across various biological and ecological domains. This phenomenon, where extreme phenotypes are favored over intermediate ones, significantly impacts population genetics and diversity by driving the formation of distinct genetic clusters. It also plays a crucial role in evolutionary adaptation and speciation, as it can lead to the emergence of new species by isolating genetic variants. Furthermore, understanding disruptive selection is essential for conservation and management efforts, as it helps in predicting and mitigating the effects of environmental changes on vulnerable populations. By examining these aspects, we can gain a deeper insight into how disruptive selection shapes the evolutionary landscape. Let us first delve into the **Impact on Population Genetics and Diversity**, where we will explore how this selective pressure influences genetic variation and population structure.
Impact on Population Genetics and Diversity
Disruptive selection, a process where natural selection favors extreme phenotypes over intermediate ones, has profound implications for population genetics and diversity. This type of selection can lead to the formation of distinct subpopulations or even new species, significantly altering the genetic landscape. When disruptive selection acts on a population, it often results in the creation of two or more adaptive peaks, each corresponding to different environmental niches or survival strategies. This can drive genetic divergence as individuals with intermediate traits are less fit and thus less likely to reproduce, while those at the extremes of the trait distribution are more likely to thrive. As a consequence, disruptive selection can increase genetic diversity within a population by maintaining multiple alleles at higher frequencies than would be expected under stabilizing selection. This is because the selection pressure against intermediate phenotypes prevents the population from converging on a single optimal trait value, allowing for the coexistence of diverse genotypes. Furthermore, this process can lead to the evolution of reproductive isolation mechanisms, as individuals with different extreme traits may develop barriers to interbreeding, thereby enhancing speciation. However, disruptive selection also has the potential to reduce overall population fitness in the short term. The increased mortality and reduced reproductive success of individuals with intermediate traits can lead to a temporary decline in population size and overall health. Additionally, if the environmental conditions change rapidly or unpredictably, the adaptive peaks favored by disruptive selection may no longer be advantageous, leading to population instability. In terms of genetic diversity, disruptive selection can result in the maintenance of genetic polymorphisms that might otherwise be lost due to genetic drift or other forms of selection. This is particularly important for populations facing changing environments, as it allows them to adapt more quickly to new conditions. For example, in species where diet varies widely among individuals, disruptive selection could favor both herbivorous and carnivorous traits, leading to a more resilient population capable of exploiting different food sources. Moreover, the impact of disruptive selection on population structure can be observed in various natural systems. For instance, in the Galapagos finches, different beak sizes and shapes have evolved under disruptive selection pressures related to food availability, leading to distinct species that occupy different ecological niches. Similarly, in human populations, genetic variations related to lactase persistence and high-altitude adaptation have been shaped by disruptive selection pressures tied to dietary and environmental factors. In conclusion, disruptive selection plays a crucial role in shaping the genetic diversity and structure of populations. By favoring extreme phenotypes over intermediate ones, it drives genetic divergence and can lead to speciation. While it may temporarily reduce population fitness and stability, it ultimately enhances the adaptability and resilience of populations to changing environments. Understanding these dynamics is essential for appreciating the complex interplay between genetic variation, environmental pressures, and evolutionary outcomes.
Role in Evolutionary Adaptation and Speciation
Disruptive selection plays a pivotal role in evolutionary adaptation and speciation, driving the diversification of species in complex and dynamic environments. This form of selection favors extreme phenotypes over intermediate ones, leading to the emergence of distinct subpopulations within a single species. As disruptive selection acts on existing genetic variation, it can accelerate the process of speciation by creating reproductive barriers between these diverging groups. For instance, in environments where resources are limited and competition is high, disruptive selection can favor individuals with traits that allow them to exploit different ecological niches. This niche partitioning reduces competition within the species, enabling each subpopulation to thrive in its respective environment. The process begins with genetic variation within a population, which is then acted upon by disruptive selection pressures. These pressures can arise from various sources such as predation, climate change, or resource availability. As individuals with extreme traits have higher fitness in their respective niches, they are more likely to survive and reproduce, passing their advantageous traits to their offspring. Over time, this leads to the accumulation of genetic differences between the subpopulations, which can eventually result in reproductive isolation. Reproductive isolation is a critical step towards speciation because it prevents gene flow between the diverging groups, allowing each group to evolve independently. This independence enables each subpopulation to adapt further to its specific environment without being constrained by the need for compatibility with other groups. For example, in the case of the Galapagos finches, disruptive selection has led to the evolution of different beak shapes and sizes that are better suited for different food sources. This specialization has contributed significantly to the diversification of these finches into distinct species. Moreover, disruptive selection can also influence the evolution of mating behaviors and other prezygotic barriers that reinforce reproductive isolation. For instance, if individuals with certain traits are more likely to mate with others having similar traits due to shared ecological preferences or behaviors, this assortative mating can further reduce gene flow between subpopulations. In conclusion, disruptive selection is a powerful force in evolutionary biology that drives adaptation and speciation by favoring extreme phenotypes and promoting niche partitioning. By creating and reinforcing reproductive barriers, it facilitates the emergence of new species that are better adapted to their environments. Understanding the role of disruptive selection provides valuable insights into how biodiversity arises and how species evolve over time in response to changing environmental pressures. This knowledge has significant implications for conservation biology and our ability to predict how populations might respond to future environmental challenges.
Conservation and Management Implications
**Conservation and Management Implications** The implications of disruptive selection on conservation and management practices are profound and multifaceted. Disruptive selection, which favors extreme phenotypes over intermediate ones, can lead to the formation of new species or the enhancement of existing genetic diversity. However, this process also poses significant challenges for conservation efforts. For instance, when populations are fragmented or reduced in size, the increased genetic drift and reduced gene flow can exacerbate the effects of disruptive selection, potentially leading to the loss of adaptive genetic variation. This scenario is particularly concerning in the context of endangered species, where maintaining genetic diversity is crucial for long-term survival. Conservation strategies must therefore be tailored to mitigate these risks. One approach is to ensure connectivity between isolated populations, facilitating gene flow and reducing the likelihood of genetic drift dominating evolutionary processes. Habitat restoration and corridor creation can be effective tools in achieving this goal. Additionally, conservation programs should prioritize the preservation of genetic diversity by managing populations in a way that maintains a balance between different phenotypic extremes. This might involve protecting a range of habitats that support various phenotypes, thereby allowing natural selection to act on a broader spectrum of traits. In managed ecosystems, such as agricultural or forestry settings, understanding disruptive selection can inform breeding programs and resource management practices. For example, in agriculture, recognizing the potential for disruptive selection can help breeders develop crop varieties that are more resilient to environmental changes by maintaining a diverse gene pool. Similarly, in forestry, managing for genetic diversity can enhance the adaptability of tree populations to climate change and other environmental stressors. Moreover, disruptive selection highlights the importance of adaptive management in conservation. Traditional conservation approaches often focus on maintaining historical conditions or restoring ecosystems to a perceived pristine state. However, disruptive selection suggests that ecosystems are dynamic and that evolutionary processes are ongoing. Therefore, conservation strategies should be flexible and adaptive, allowing for the evolution of populations in response to changing environmental conditions. Finally, the study of disruptive selection underscores the need for interdisciplinary collaboration between ecologists, evolutionary biologists, and conservation practitioners. By integrating insights from these fields, conservation efforts can be more effectively targeted to address the complex interplay between genetic variation, environmental change, and population dynamics. This holistic approach is essential for developing robust conservation strategies that account for the dynamic nature of evolutionary processes and ensure the long-term sustainability of ecosystems. In summary, disruptive selection has significant implications for conservation and management practices. It emphasizes the importance of preserving genetic diversity, ensuring population connectivity, and adopting adaptive management strategies. By understanding and addressing these implications, we can better protect and manage ecosystems in a way that supports their resilience and adaptability in the face of ongoing environmental changes.