What Is Electrical Discharge
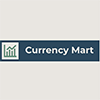
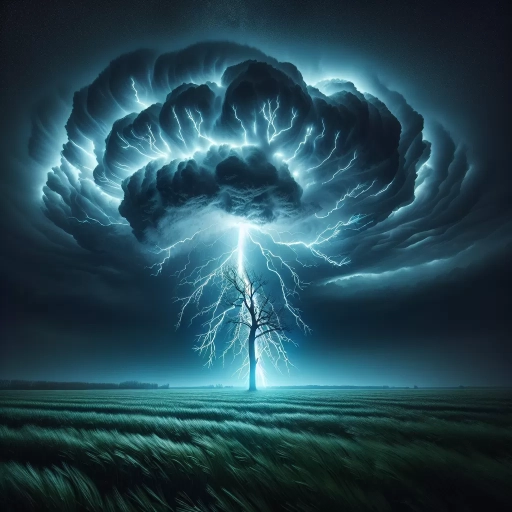
Electrical discharge, a phenomenon where an electric current flows through a medium such as air or a gas, is a fundamental concept in physics and engineering. This process involves the ionization of the medium, leading to the creation of plasma and the release of energy. Understanding electrical discharge is crucial due to its wide-ranging applications across various fields, including manufacturing, medical treatments, and environmental remediation. However, it also raises important safety considerations and environmental impacts that must be addressed. In this article, we will delve into the definition and principles of electrical discharge, explore its diverse applications, and discuss the safety and environmental implications associated with it. To begin, let's first examine the definition and principles of electrical discharge to lay the groundwork for a comprehensive understanding of this complex and multifaceted topic.
Definition and Principles of Electrical Discharge
Electrical discharge, a fundamental phenomenon in physics and engineering, involves the release of electrical energy through a medium, such as air or a gas, resulting in the ionization of the medium and the flow of electric current. Understanding electrical discharge is crucial for various applications, including plasma technology, high-voltage engineering, and materials processing. This article delves into the definition and principles of electrical discharge, exploring three key aspects: the basic mechanism of electrical discharge, the various types of electrical discharges, and the key factors influencing these discharges. By examining the basic mechanism, we uncover how electrons and ions interact to create a conductive pathway. The discussion on types of electrical discharges highlights the differences between phenomena like spark discharges, glow discharges, and arc discharges. Finally, understanding the key factors influencing electrical discharges, such as voltage, pressure, and electrode geometry, provides insights into how these discharges can be controlled and optimized. To begin, let's dive into the **Basic Mechanism of Electrical Discharge**, which forms the foundation of this complex and fascinating field.
Basic Mechanism of Electrical Discharge
The basic mechanism of electrical discharge involves the movement of charged particles, such as electrons and ions, through a medium like air or a gas. This process begins with the application of an electric field strong enough to overcome the resistance of the medium. Here’s a detailed breakdown: 1. **Ionization**: When an electric field is applied, it can strip away electrons from neutral atoms or molecules in the medium, creating ions and free electrons. This initial ionization can occur due to thermal energy, photoionization, or collisional ionization. 2. **Avalanche Effect**: The free electrons accelerated by the electric field collide with other neutral atoms or molecules, causing further ionization. This cascade effect, known as the avalanche process, rapidly increases the number of charged particles. 3. **Conductivity**: As more ions and electrons are generated, the medium becomes conductive. The increased conductivity allows more current to flow, which in turn strengthens the electric field and accelerates the ionization process. 4. **Breakdown Voltage**: There is a critical voltage threshold, known as the breakdown voltage, beyond which the electric field becomes strong enough to sustain an electrical discharge. Below this threshold, the discharge cannot be maintained. 5. **Discharge Types**: Electrical discharges can manifest in various forms depending on conditions such as pressure and voltage. Common types include spark discharges (e.g., lightning), glow discharges (e.g., neon signs), and arc discharges (e.g., welding arcs). 6. **Energy Transfer**: During an electrical discharge, energy is transferred from the electric field to the charged particles. This energy can be dissipated as heat, light, or sound, depending on the nature of the discharge. 7. **Stability and Sustenance**: For an electrical discharge to be sustained, there must be a continuous supply of energy to maintain the ionization process. This can be achieved through external power sources or self-sustaining mechanisms like those in plasma arcs. Understanding these mechanisms is crucial for applications ranging from high-voltage engineering to plasma physics and materials processing. Each step in the process contributes to the complex dynamics that govern electrical discharges, making them both fascinating and highly useful phenomena in various fields of science and technology.
Types of Electrical Discharge
Electrical discharge encompasses various phenomena where electric current flows through a medium, often resulting in the emission of light, heat, and other forms of energy. There are several key types of electrical discharge, each with distinct characteristics and applications. 1. **Spark Discharge**: This type occurs when a high voltage is applied across a small gap between two electrodes, causing a sudden release of energy. Spark discharges are commonly seen in spark plugs of internal combustion engines and are used to ignite fuel-air mixtures. 2. **Arc Discharge**: Unlike spark discharges, arc discharges involve a sustained flow of current between electrodes. Arcs are characterized by their continuous nature and are used in welding processes, such as shielded metal arc welding (SMAW) and gas metal arc welding (GMAW). 3. **Glow Discharge**: This type of discharge occurs at lower voltages than spark or arc discharges and is typically seen in neon signs and plasma TVs. Glow discharges involve the ionization of gas molecules, leading to the emission of light. 4. **Corona Discharge**: Corona discharges happen when there is a high voltage gradient near a conductor, causing ionization of the surrounding air or gas. This type is often observed around high-voltage transmission lines and can be seen as a faint glow. 5. **Dielectric Breakdown**: This occurs when an insulating material fails under the application of an electric field, leading to a sudden flow of current through the material. Dielectric breakdown can result in the destruction of the insulator and is a critical consideration in the design of electrical systems. 6. **Thermal Ionization**: Also known as thermal discharge, this type involves the ionization of a gas due to high temperatures rather than an external electric field. Thermal ionization is crucial in processes like plasma cutting and plasma etching. 7. **Radio-Frequency Discharge**: This type uses radio-frequency energy to create and sustain a plasma. Radio-frequency discharges are widely used in semiconductor manufacturing for etching and deposition processes. Understanding these different types of electrical discharges is essential for various industrial applications, from power transmission and distribution to manufacturing and medical treatments. Each type has its unique principles and applications, making electrical discharge a versatile and critical aspect of modern technology.
Key Factors Influencing Electrical Discharge
Key factors influencing electrical discharge include several critical parameters that determine the characteristics and efficiency of the discharge process. **Voltage** is a primary factor, as it dictates the potential difference driving the discharge. Higher voltages typically lead to more energetic discharges, while lower voltages may result in weaker or unstable discharges. **Current** also plays a crucial role, as it affects the amount of charge transferred during the discharge. Higher currents can lead to more intense discharges but may also increase the risk of overheating or damage to equipment. **Electrode Material** is another significant factor, influencing both the initiation and sustainability of the discharge. Different materials have varying work functions and thermal properties, which can affect the ease of electron emission and heat dissipation. For instance, electrodes made from materials with low work functions (like tungsten) are more conducive to electron emission and thus facilitate easier discharge initiation. **Gap Distance** between electrodes is critical in determining the likelihood and characteristics of electrical discharge. The shorter the gap, the lower the voltage required for discharge initiation. Conversely, larger gaps require higher voltages to overcome the air resistance and initiate a discharge. **Atmospheric Conditions**, such as air pressure and humidity, also impact electrical discharges. Lower air pressures reduce the resistance to electron flow, making discharges easier to initiate, while high humidity can lead to increased ionization and conductivity in the air. **Temperature** affects the thermal stability of both the electrodes and the surrounding medium, influencing how efficiently heat is dissipated during a discharge. Higher temperatures can increase the likelihood of secondary discharges due to thermal ionization. **Magnetic Fields** can influence the path and stability of electrical discharges by exerting forces on moving charges, potentially altering the discharge trajectory or enhancing plasma confinement. Lastly, **Pulse Duration** and **Frequency** are important in pulsed electrical discharges. Shorter pulse durations can lead to higher peak currents and more localized heating effects, while higher frequencies may result in more stable plasma formation due to repeated ionization events. Understanding these key factors is essential for optimizing electrical discharge processes in various applications, from industrial machining to medical treatments and plasma physics research. By carefully controlling these parameters, engineers can tailor electrical discharges to achieve specific outcomes with enhanced efficiency and safety.
Applications of Electrical Discharge in Various Fields
Electrical discharge, a phenomenon characterized by the flow of electric current through a gas or plasma, has diverse and significant applications across various fields. This versatile technology is not only crucial in industrial settings but also plays a vital role in medical and scientific research. In industrial contexts, electrical discharge machining (EDM) is a key process for precision machining and material processing, allowing for the creation of complex shapes and precise cuts in hard materials. Beyond industry, medical applications leverage electrical discharge for surgical tools and diagnostic equipment, enhancing precision and efficacy in healthcare. Additionally, in scientific research, electrical discharge is integral to the study of plasma physics and astrophysics, providing insights into high-energy phenomena. This article will delve into these applications, starting with the industrial uses of electrical discharge in machining and material processing, where its precision and versatility are particularly evident.
Industrial Uses: Machining and Material Processing
Industrial machining and material processing are pivotal applications of electrical discharge, particularly through Electrical Discharge Machining (EDM) and Wire Electrical Discharge Machining (WEDM). EDM is a non-traditional machining method that uses electrical discharges to remove material from a workpiece, making it ideal for intricate and complex geometries that conventional machining techniques cannot achieve. This process is highly precise, allowing for the creation of fine details and micro-features without the need for mechanical contact, thus minimizing the risk of damage to the workpiece. In EDM, an electrode and the workpiece are submerged in a dielectric fluid, and a high-frequency electrical discharge is generated between them. The discharge erodes the material, creating the desired shape. This method is particularly useful in aerospace, automotive, and medical industries where precision and accuracy are paramount. For instance, EDM can be used to create complex shapes in hardened steel or other hard materials that are difficult to machine using traditional methods. WEDM, a variant of EDM, employs a thin wire as the electrode to cut through conductive materials. This technique is widely used in the production of dies and molds, as well as in the aerospace and automotive sectors for cutting intricate profiles and shapes. WEDM offers superior accuracy and surface finish compared to traditional cutting methods, making it an essential tool in modern manufacturing. Another significant application of electrical discharge in material processing is in the field of surface modification. Electrical discharge can be used to create textured surfaces or to apply coatings, enhancing the wear resistance and other mechanical properties of materials. This is particularly beneficial in industries such as textiles and biomedical engineering, where surface characteristics play a critical role in product performance. Furthermore, electrical discharge is utilized in material joining processes like Electrical Discharge Welding (EDW), which combines the principles of EDM with welding techniques. EDW is advantageous for joining thin sheets or delicate components without causing thermal distortion, making it suitable for applications in electronics and precision engineering. In summary, the industrial uses of electrical discharge in machining and material processing are diverse and critical. From the precision cutting capabilities of EDM and WEDM to surface modification and material joining, these techniques have revolutionized various manufacturing processes, enabling the production of complex components with high accuracy and reliability. As technology continues to evolve, the role of electrical discharge in industrial applications is likely to expand further, driving innovation and efficiency across multiple sectors.
Medical Applications: Surgical and Diagnostic Tools
In the realm of medical applications, electrical discharge plays a crucial role in both surgical and diagnostic tools, enhancing precision, safety, and efficacy. One of the most significant applications is in electrosurgery, where electrical discharge is used to cut, coagulate, and desiccate tissues. Electrosurgical units (ESUs) utilize high-frequency electrical currents to achieve these functions, minimizing blood loss and reducing the risk of infection. For instance, in procedures such as laparoscopic surgery or neurosurgery, ESUs enable surgeons to make precise incisions and control bleeding with minimal thermal spread, thereby preserving surrounding healthy tissue. Diagnostic tools also leverage electrical discharge technology. For example, in the field of cardiology, defibrillators use electrical discharges to restore normal heart rhythm in patients experiencing life-threatening arrhythmias. These devices deliver controlled shocks to the heart muscle, helping to reestablish a stable heartbeat. Additionally, electrocautery devices are used in various medical specialties for procedures like skin biopsies or removal of benign lesions, where precise tissue destruction is required without causing significant damage to adjacent tissues. Furthermore, electrical discharge is integral to certain imaging technologies. In radiofrequency ablation (RFA), electrical energy is used to heat and destroy abnormal cells, such as those found in tumors. This technique is often guided by imaging modalities like ultrasound or MRI, ensuring accurate targeting of the affected area. Similarly, in some types of lithotripsy for kidney stone treatment, focused shockwaves generated by electrical discharges are used to break down stones into smaller fragments that can then be easily passed out of the body. The precision and control offered by electrical discharge technology have also led to its adoption in ophthalmology. For example, in cataract surgery, phacoemulsification involves using ultrasonic waves generated by an electrical discharge to break up the lens before it is aspirated out of the eye. This method allows for smaller incisions and faster recovery times compared to traditional surgical techniques. In summary, the application of electrical discharge in medical tools has revolutionized various aspects of healthcare by providing surgeons and diagnosticians with highly precise and effective instruments. From electrosurgery and defibrillation to imaging-guided therapies and specialized treatments like RFA and phacoemulsification, these technologies continue to improve patient outcomes and advance the field of medicine.
Scientific Research: Plasma Physics and Astrophysics
Scientific research in plasma physics and astrophysics has revolutionized our understanding of the universe and its fundamental processes. Plasma, a high-energy state of matter characterized by the presence of ions and free electrons, is ubiquitous in astrophysical environments, from the solar corona to interstellar space. In plasma physics, researchers delve into the dynamics of plasmas under various conditions, including magnetic confinement, which is crucial for fusion energy research. This field has significant implications for developing sustainable energy sources, such as tokamaks and stellarators, which aim to harness the energy released by nuclear fusion reactions. In astrophysics, the study of plasmas helps scientists understand phenomena like solar flares, coronal mass ejections, and the behavior of black holes. Advanced computational models and experimental techniques, such as particle-in-cell simulations and laboratory experiments using high-powered lasers, are employed to mimic these astrophysical conditions. These studies not only enhance our comprehension of cosmic events but also provide insights into the formation and evolution of stars and galaxies. The intersection of plasma physics and astrophysics also drives technological innovation. For instance, understanding plasma behavior in space plasmas informs the design of spacecraft shielding against harmful radiation and charged particles. Additionally, research on plasma instabilities and turbulence has led to advancements in materials science and nanotechnology. Moreover, the principles derived from plasma physics are applied in various industrial fields. For example, plasma etching and deposition techniques are essential in semiconductor manufacturing, allowing for the precise creation of microelectronic devices. Similarly, plasma-based surface modification improves the properties of materials used in biomedical applications, such as implantable devices. The synergy between fundamental scientific inquiry and practical applications underscores the importance of continued research in plasma physics and astrophysics. As scientists continue to explore the complexities of plasmas, they uncover new avenues for technological innovation that can transform multiple fields, from energy production to space exploration and beyond. This interdisciplinary approach ensures that the advancements in plasma physics and astrophysics contribute significantly to both our understanding of the universe and the development of cutting-edge technologies.
Safety Considerations and Environmental Impact
When discussing safety considerations and environmental impact, it is crucial to approach the topic with a comprehensive understanding of the various factors involved. This includes conducting thorough risk assessments and implementing robust safety protocols to mitigate potential hazards. Additionally, it is essential to examine the environmental effects of industrial activities, particularly air and water pollution, which can have severe and long-lasting consequences. Regulatory standards and compliance also play a critical role in ensuring that operations are conducted in a manner that minimizes harm to both human health and the environment. By understanding these interconnected elements, we can better navigate the complexities of safety and environmental management. This article will delve into these key areas, starting with the importance of risk assessment and safety protocols, which form the foundation of any effective safety strategy.
Risk Assessment and Safety Protocols
Risk assessment and safety protocols are crucial components in any environment where electrical discharge is involved. These measures ensure the protection of personnel, equipment, and the environment from potential hazards. Here’s a detailed look at how these protocols are implemented: **Risk Assessment:** 1. **Identification of Hazards:** The first step involves identifying all possible risks associated with electrical discharge, such as electrical shock, fire, and explosions. 2. **Evaluation of Risks:** Each identified hazard is evaluated to determine its likelihood and potential impact. 3. **Prioritization:** Risks are prioritized based on their severity and likelihood, allowing for focused mitigation efforts. **Safety Protocols:** 1. **Personal Protective Equipment (PPE):** Workers are equipped with PPE such as insulating gloves, safety glasses, and fire-resistant clothing to protect against electrical shock and other hazards. 2. **Isolation and Lockout/Tagout Procedures:** Ensuring that equipment is properly isolated from power sources before maintenance or repairs reduces the risk of accidental electrical discharge. 3. **Grounding and Bonding:** Proper grounding and bonding practices prevent the buildup of static electricity and ensure safe operation of equipment. 4. **Regular Maintenance:** Regular inspection and maintenance of electrical systems help identify and rectify potential issues before they become hazardous. 5. **Training and Awareness:** Comprehensive training programs ensure that all personnel understand the risks associated with electrical discharge and know how to respond in case of emergencies. 6. **Emergency Response Plans:** Detailed emergency response plans are in place to handle incidents such as electrical fires or shocks, ensuring prompt and effective action. **Environmental Impact Considerations:** 1. **Waste Management:** Proper disposal of materials used in electrical discharge processes minimizes environmental contamination. 2. **Energy Efficiency:** Implementing energy-efficient practices reduces the overall environmental impact by minimizing energy consumption. 3. **Compliance with Regulations:** Adhering to local and international regulations ensures that operations do not harm the environment. By integrating thorough risk assessments with robust safety protocols, organizations can significantly reduce the risks associated with electrical discharge while also mitigating their environmental impact. This holistic approach not only protects human life but also contributes to a sustainable future.
Environmental Effects: Air and Water Pollution
Environmental Effects: Air and Water Pollution The process of electrical discharge, while crucial for various industrial and technological applications, poses significant environmental concerns, particularly in terms of air and water pollution. Electrical discharges, such as those used in plasma cutting, welding, and other high-energy processes, can release harmful pollutants into the atmosphere. These emissions include particulate matter, volatile organic compounds (VOCs), and gases like nitrogen oxides (NOx) and sulfur dioxide (SO2), which contribute to air pollution. NOx and SO2 are precursors to acid rain and ground-level ozone formation, exacerbating respiratory issues and damaging ecosystems. Additionally, the generation of ozone (O3) during electrical discharges can lead to local air quality degradation, posing health risks to nearby populations. In terms of water pollution, the cooling systems associated with electrical discharge processes can discharge heated water into natural water bodies, causing thermal pollution. This can disrupt aquatic life by altering the natural temperature balance, affecting the metabolism and survival of aquatic organisms. Furthermore, chemical contaminants from the discharge processes can enter waterways through runoff or improper disposal, leading to chemical pollution. For instance, heavy metals and other toxic substances used in some electrical discharge applications can contaminate water sources, posing long-term risks to both human health and the environment. To mitigate these environmental impacts, it is essential to implement stringent safety measures and environmental regulations. This includes the use of advanced filtration systems to capture airborne pollutants, proper wastewater treatment facilities to handle chemical contaminants, and adherence to best practices for minimizing thermal pollution. Regular monitoring of emissions and effluents is also crucial to ensure compliance with environmental standards. By integrating these measures into the operational framework of electrical discharge processes, we can significantly reduce their adverse effects on air and water quality, thereby protecting both human health and the environment.
Regulatory Standards and Compliance
Regulatory standards and compliance are crucial components in the realm of electrical discharge, particularly when considering safety considerations and environmental impact. These standards ensure that electrical discharge processes, such as those used in machining, surface treatment, and other industrial applications, are conducted in a manner that minimizes risks to both human health and the environment. Regulatory bodies like the Occupational Safety and Health Administration (OSHA) in the United States and the European Union's Machinery Directive set forth specific guidelines to mitigate hazards associated with electrical discharges. For instance, OSHA mandates the use of personal protective equipment (PPE) and proper ventilation systems to prevent exposure to harmful fumes and electrical shock. Similarly, environmental regulations such as those enforced by the Environmental Protection Agency (EPA) dictate the handling and disposal of waste materials generated during electrical discharge processes to prevent pollution. Compliance with these standards involves regular audits, training programs for personnel, and the implementation of safety protocols that are continuously updated to reflect the latest technological advancements and best practices. Additionally, adherence to international standards like ISO 9001 for quality management and ISO 14001 for environmental management further ensures that electrical discharge operations are not only safe but also sustainable. By adhering to these regulatory standards, industries can significantly reduce the risk of accidents, environmental degradation, and legal liabilities, thereby fostering a safer and more responsible operational environment. This holistic approach to compliance underscores the importance of integrating safety considerations and environmental impact into the core of electrical discharge practices.