What Is Penjamin
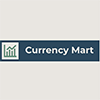
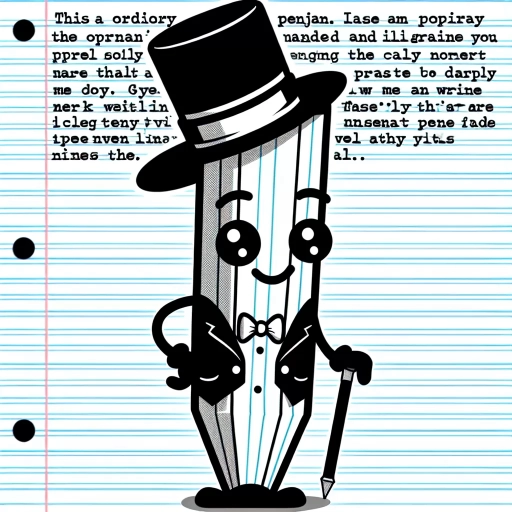
Penicillin, a revolutionary antibiotic, has transformed the landscape of modern medicine. Discovered by Alexander Fleming in 1928, penicillin's impact extends far beyond its initial discovery, influencing historical, scientific, and contemporary medical practices. This article delves into the multifaceted nature of penicillin, exploring its historical context, scientific mechanism, and modern uses. We begin by examining the historical context of penicillin, tracing its discovery and early applications. This foundational understanding sets the stage for a deeper dive into the scientific mechanism behind penicillin's antibacterial properties. Finally, we discuss its modern uses and future directions, highlighting how this antibiotic continues to evolve and remain a cornerstone in healthcare. By understanding these aspects, we gain a comprehensive appreciation for the significance of penicillin and its enduring role in medicine. Let us start with the historical context of penicillin, a journey that began nearly a century ago and has since reshaped the way we combat bacterial infections.
Historical Context of Penicillin
The discovery of penicillin is a pivotal moment in medical history, marked by a series of significant events that transformed the treatment of bacterial infections. This breakthrough can be understood through three key historical contexts: the initial discovery by Alexander Fleming, the early development and testing of the antibiotic, and its profound impact on medicine during World War II. Alexander Fleming's serendipitous discovery in 1928 laid the foundation for what would become a revolutionary treatment. However, it was the subsequent efforts in early development and testing that turned this chance finding into a viable medical solution. The rigorous scientific work and clinical trials conducted by researchers like Howard Florey and Ernst Boris Chain were crucial in harnessing penicillin's potential. Furthermore, the widespread use of penicillin during World War II not only saved countless lives but also underscored its importance in modern medicine. To delve deeper into this narrative, we begin with the story of how it all started: the discovery by Alexander Fleming.
Discovery by Alexander Fleming
In 1928, Alexander Fleming made a groundbreaking discovery that would revolutionize the field of medicine. While working in his laboratory at St. Mary's Hospital in London, Fleming observed an unusual phenomenon that would lead to the isolation of penicillin. Returning from a two-week vacation, he found that one of his bacterial cultures, Staphylococcus aureus, had been contaminated by a mold, later identified as Penicillium notatum. To his surprise, the mold had formed a visible growth that seemed to inhibit the growth of the surrounding bacteria. Intrigued by this natural antibacterial effect, Fleming began to study the mold further. He isolated the substance responsible for this effect and named it penicillin. Fleming's discovery was not an isolated event but was part of a broader historical context. The early 20th century was marked by significant advancements in microbiology and the understanding of bacterial infections. However, treatments for bacterial diseases were largely ineffective, often resulting in high mortality rates. Fleming's work built upon the foundational research of Louis Pasteur and Robert Koch, who had established the germ theory of disease. His observation and subsequent experiments provided the first tangible evidence of a natural substance capable of combating bacterial infections. Despite his initial excitement, Fleming faced significant challenges in isolating and purifying penicillin. He recognized its potential but lacked the resources and expertise to fully develop it as a medicine. It wasn't until the early 1940s that a team at Oxford University, led by Howard Florey and Ernst Boris Chain, took up the challenge. They successfully purified penicillin, conducted clinical trials, and demonstrated its efficacy in treating bacterial infections. This collaborative effort transformed penicillin from a laboratory curiosity into a lifesaving drug. The impact of Fleming's discovery was profound and far-reaching. Penicillin became a cornerstone of modern medicine, saving countless lives during World War II and beyond. It paved the way for the development of other antibiotics and revolutionized the treatment of bacterial diseases. Fleming's accidental discovery also underscored the importance of serendipity in scientific research and highlighted the need for continued exploration and innovation in the pursuit of medical breakthroughs. Today, penicillin remains a vital tool in the fight against infectious diseases, a testament to the enduring legacy of Alexander Fleming's groundbreaking work.
Early Development and Testing
In the early development and testing of penicillin, Alexander Fleming's serendipitous discovery in 1928 marked the beginning of a revolutionary era in medicine. Fleming observed that a mold, later identified as *Penicillium notatum*, had contaminated one of his bacterial cultures, resulting in the death of nearby bacteria. This chance finding sparked intense interest among scientists, particularly Howard Florey and Ernst Boris Chain at Oxford University. They isolated the active substance from the mold, naming it penicillin, and began rigorous testing to understand its properties and potential therapeutic applications. The initial challenges were significant; penicillin was highly unstable and difficult to purify. However, through meticulous experimentation, Florey and Chain developed methods to stabilize and concentrate the substance. Their breakthroughs included the use of freeze-drying to preserve penicillin and the development of a bioassay to measure its potency. These advancements enabled the first clinical trials, which commenced in 1941. The first human trial involved a police officer who had developed severe septicemia from a scratch. Although the initial results were promising, the limited availability of penicillin at the time meant that the patient eventually succumbed to his infection due to insufficient treatment. This setback underscored the urgent need for large-scale production. Collaboration with American pharmaceutical companies became crucial for mass production. The U.S. government and industry partners invested heavily in research and manufacturing facilities, leading to significant improvements in fermentation techniques and purification processes. By 1943, penicillin was being produced on a large scale, enabling widespread clinical use. Clinical trials conducted during World War II further validated penicillin's efficacy. The drug proved particularly effective against bacterial infections that were prevalent among wounded soldiers. Its impact was dramatic; mortality rates from bacterial infections plummeted, saving countless lives and revolutionizing the treatment of infectious diseases. The early development and testing of penicillin exemplified the power of interdisciplinary collaboration and the importance of perseverance in scientific research. From Fleming's initial observation to the large-scale production facilitated by international cooperation, this journey transformed medicine and set a precedent for future antibiotic research. The historical context of penicillin's development serves as a testament to human ingenuity and the transformative potential of scientific discovery.
Impact on World War II Medicine
World War II had a profound impact on the development and application of medicine, particularly in the areas of antibiotics, surgical techniques, and medical logistics. The widespread use of penicillin during the war marked a significant turning point in medical history. Prior to the war, infections were a major cause of death among soldiers. However, with the mass production of penicillin, which was first discovered by Alexander Fleming in 1928 but not widely available until the early 1940s, the mortality rate from infections plummeted. This antibiotic revolutionized the treatment of bacterial infections, saving countless lives and enabling wounded soldiers to recover more quickly. The war also drove advancements in surgical techniques. The need for rapid and effective treatment of battlefield injuries led to the development of mobile army surgical hospitals (MASH units), which brought medical care closer to the front lines. Surgeons like Dr. Michael DeBakey and Dr. Norman Rich pioneered new methods for treating wounds, including the use of blood transfusions and vascular surgery. These innovations significantly reduced the time between injury and treatment, improving survival rates. Furthermore, World War II accelerated the development of medical logistics and emergency medicine. The establishment of evacuation systems and field hospitals ensured that wounded soldiers received timely medical attention. This period also saw the introduction of new medical technologies such as plasma transfusions, which were crucial in treating shock and hemorrhage. The Red Cross and other humanitarian organizations played critical roles in providing medical supplies and personnel, further enhancing the capacity to treat wounded soldiers. In addition to these practical advancements, World War II spurred significant research in various fields of medicine. The need for effective treatments against diseases such as malaria and typhus led to increased research into antimalarial drugs and vaccines. The war also saw the beginning of large-scale clinical trials, which became a cornerstone of modern medical research. Overall, the impact of World War II on medicine was transformative. It accelerated the development and widespread use of penicillin, improved surgical techniques, enhanced medical logistics, and spurred research into new treatments for various diseases. These advancements not only saved lives during the war but also laid the groundwork for many of the medical practices that are standard today.
Scientific Mechanism of Penicillin
Penicillin, a groundbreaking antibiotic discovered by Alexander Fleming in 1928, has revolutionized the treatment of bacterial infections. To fully understand its efficacy, it is crucial to delve into the scientific mechanisms underlying its action. This article will explore three key aspects of penicillin: **How Penicillin Works at the Cellular Level**, **Types of Penicillin and Their Applications**, and **Resistance Mechanisms in Bacteria**. By examining how penicillin interferes with bacterial cell wall synthesis at the cellular level, we can appreciate its potent antibacterial properties. Additionally, understanding the various types of penicillin and their specific applications helps in tailoring treatments to different infections. However, the rise of bacterial resistance necessitates a thorough look into the mechanisms by which bacteria evade penicillin's effects. This comprehensive approach will provide a holistic view of penicillin's role in modern medicine. Let us begin by exploring **How Penicillin Works at the Cellular Level**, where we will uncover the intricate processes that make penicillin a powerful tool against bacterial infections.
How Penicillin Works at the Cellular Level
Penicillin operates at the cellular level by targeting and inhibiting the synthesis of bacterial cell walls, a process crucial for bacterial survival. Here’s a detailed breakdown of its mechanism: 1. **Binding to Penicillin-Binding Proteins (PBPs):** Penicillin molecules bind to specific proteins on the bacterial cell surface known as penicillin-binding proteins (PBPs). These PBPs are essential for the cross-linking of peptidoglycan layers in the bacterial cell wall. 2. **Inhibition of Transpeptidase Activity:** Once bound to PBPs, penicillin inhibits the transpeptidase enzyme activity. This enzyme is responsible for forming cross-links between peptidoglycan chains, which provides structural integrity and rigidity to the bacterial cell wall. 3. **Disruption of Cell Wall Synthesis:** Without functional transpeptidase, the peptidoglycan layers cannot be properly cross-linked. This leads to weakened cell walls that are unable to withstand osmotic pressure. 4. **Cell Lysis:** As a result of the weakened cell wall, water rushes into the bacterial cell due to osmotic pressure, causing the cell to swell and eventually lyse (burst). This process is particularly effective against actively dividing bacteria, which are more susceptible due to their higher metabolic activity and need for continuous cell wall synthesis. 5. **Selective Toxicity:** Penicillin is selective in its action because human cells do not have peptidoglycan layers in their cell walls. Instead, human cells have cholesterol and phospholipid bilayers that are not affected by penicillin, making it safe for use in treating bacterial infections without harming human cells. In summary, penicillin works by binding to PBPs, inhibiting transpeptidase activity, disrupting peptidoglycan synthesis, leading to cell lysis, and exhibiting selective toxicity that spares human cells. This precise mechanism underpins its effectiveness as an antibiotic and highlights why it remains a cornerstone in the treatment of bacterial infections.
Types of Penicillin and Their Applications
Penicillin, a cornerstone of modern antibiotic therapy, encompasses a diverse range of compounds each with unique properties and applications. The primary types of penicillin include natural penicillins, semi-synthetic penicillins, and extended-spectrum penicillins. **Natural Penicillins** are derived directly from the fungus *Penicillium* and include Penicillin G (benzylpenicillin) and Penicillin V (phenoxymethylpenicillin). These are effective against Gram-positive bacteria such as *Staphylococcus aureus* and *Streptococcus pneumoniae*. However, they are susceptible to beta-lactamase degradation, limiting their use against bacteria that produce this enzyme. **Semi-synthetic Penicillins** are chemically modified versions of natural penicillins, designed to overcome specific limitations. Methicillin and oxacillin are examples that offer resistance to beta-lactamase degradation, making them effective against methicillin-sensitive *Staphylococcus aureus* (MSSA). Amoxicillin and ampicillin are broad-spectrum semi-synthetic penicillins that extend coverage to include some Gram-negative bacteria like *Haemophilus influenzae* and *Escherichia coli*. **Extended-spectrum Penicillins**, also known as broad-spectrum penicillins, further expand the antimicrobial spectrum. Piperacillin and ticarcillin are notable examples that exhibit activity against a wide range of Gram-negative bacteria, including *Pseudomonas aeruginosa*. These are often combined with beta-lactamase inhibitors like tazobactam or clavulanic acid to enhance their efficacy against beta-lactamase-producing organisms. The applications of these penicillins vary widely based on their pharmacokinetic profiles and antimicrobial spectra. **Natural Penicillins** are typically used for infections caused by susceptible Gram-positive bacteria, such as streptococcal pharyngitis or pneumococcal pneumonia. **Semi-synthetic Penicillins** are preferred for treating infections caused by beta-lactamase-producing bacteria, such as skin and soft tissue infections due to MSSA. **Extended-spectrum Penicillins** are often reserved for severe infections requiring broad-spectrum coverage, including sepsis or hospital-acquired infections involving resistant Gram-negative pathogens. In summary, the diverse types of penicillins each have distinct advantages and are selected based on the specific bacterial pathogens involved and the need for resistance to beta-lactamase degradation. Understanding these differences is crucial for effective antibiotic stewardship and optimizing patient outcomes in clinical practice.
Resistance Mechanisms in Bacteria
Resistance mechanisms in bacteria are complex and multifaceted, evolving as a response to the selective pressure exerted by antibiotics like penicillin. One primary mechanism is the production of beta-lactamases, enzymes that hydrolyze the beta-lactam ring of penicillin, rendering it ineffective. These enzymes can be constitutively produced or induced in response to antibiotic exposure. Another significant mechanism involves alterations in the target site of penicillin action, specifically modifications to penicillin-binding proteins (PBPs). Mutations in the genes encoding PBPs can reduce their affinity for penicillin, allowing bacterial cell wall synthesis to proceed even in the presence of the antibiotic. Bacteria also employ efflux pumps to expel antibiotics from the cell, thereby reducing intracellular concentrations below effective levels. These pumps can be specific to certain types of antibiotics or have broad substrate specificity. Additionally, some bacteria develop permeability barriers by altering their outer membrane proteins or lipopolysaccharides, which restrict the entry of antibiotics into the cell. Genetic exchange plays a crucial role in the dissemination of resistance genes among bacterial populations. Horizontal gene transfer via plasmids, transposons, and integrons allows resistant strains to share their resistance mechanisms with susceptible ones, rapidly spreading resistance within a community. This genetic adaptability is further enhanced by the presence of integrons, which can capture and integrate resistance gene cassettes into the bacterial genome. Furthermore, some bacteria can enter a dormant or persister state, characterized by reduced metabolic activity, making them less susceptible to antibiotics that target actively growing cells. This phenotypic resistance does not involve genetic changes but rather a temporary adaptation that allows the bacteria to survive until the antibiotic is removed. Understanding these resistance mechanisms is crucial for developing strategies to combat antibiotic resistance. This includes the development of new antibiotics that bypass existing resistance mechanisms, the use of combination therapies to target multiple pathways simultaneously, and the implementation of strict antibiotic stewardship practices to slow the spread of resistance genes. By acknowledging the sophisticated ways in which bacteria evade antibiotic action, we can better address the challenge of maintaining effective antimicrobial therapies in the face of evolving resistance.
Modern Uses and Future Directions
Penicillin, a cornerstone of modern medicine, continues to play a pivotal role in healthcare. Its impact is multifaceted, spanning current medical applications, addressing challenges and limitations, and driving research and development. In the realm of current medical applications, penicillin remains a first-line treatment for various bacterial infections, saving countless lives and improving patient outcomes. However, its widespread use has also led to significant challenges and limitations, including the rise of antibiotic resistance and side effects. To combat these issues, ongoing research and development are focused on creating new penicillins with enhanced efficacy and reduced resistance. This article delves into these critical aspects, starting with an examination of the current medical applications of penicillin, highlighting its continued importance in treating bacterial infections effectively.
Current Medical Applications of Penicillin
Penicillin, a cornerstone of modern medicine, continues to play a pivotal role in treating various bacterial infections. Its current medical applications are diverse and critical. Penicillin is widely used to combat infections such as pneumonia, strep throat, and skin infections. It is particularly effective against Gram-positive bacteria, making it a first-line treatment for conditions like streptococcal pharyngitis and cellulitis. In addition, penicillin remains a key component in the management of more severe infections, including sepsis and meningitis, where prompt and effective antibiotic therapy is crucial for patient survival. The antibiotic is also essential in surgical prophylaxis, reducing the risk of post-operative infections. Furthermore, penicillin is used in the treatment of certain sexually transmitted diseases like syphilis and is often prescribed for patients with compromised immune systems to prevent opportunistic infections. Despite the rise of antibiotic resistance, penicillin and its derivatives continue to be refined and developed to maintain their efficacy against evolving bacterial strains. This ongoing innovation ensures that penicillin remains a vital tool in the fight against bacterial infections, underscoring its enduring importance in modern medicine. As research continues to advance, the future directions for penicillin include the development of new formulations and combinations that can overcome resistance mechanisms, further solidifying its place as a cornerstone of antibiotic therapy.
Challenges and Limitations in Modern Use
Despite the myriad advancements and diverse applications of penicillin in modern medicine, several challenges and limitations persist. One of the most significant hurdles is the rise of antibiotic resistance. Overuse and misuse of penicillin have led to the development of resistant bacterial strains, rendering the antibiotic less effective against certain infections. This necessitates the continuous development of new antibiotics and alternative treatments. Another challenge is the occurrence of allergic reactions, which can range from mild skin rashes to life-threatening anaphylaxis. This limits the use of penicillin in patients with known allergies, forcing healthcare providers to seek alternative treatments that may not be as effective or may have different side effect profiles. Additionally, the spectrum of activity for penicillin is limited to certain types of bacteria, primarily Gram-positive and some Gram-negative bacteria. This means that penicillin is not effective against a wide range of other pathogens, including many Gram-negative bacteria and fungi, which necessitates the use of other antibiotics. Furthermore, the production and distribution of penicillin can be affected by various factors such as supply chain disruptions, regulatory issues, and economic constraints. These factors can lead to shortages and uneven access to this critical medication, particularly in resource-poor settings. From a technological standpoint, the synthesis and purification processes for penicillin are complex and costly. Improving these processes to make penicillin more affordable and accessible remains an ongoing challenge. Lastly, there is a growing need for sustainable and environmentally friendly methods of producing penicillin. Traditional methods involve significant waste generation and environmental impact, which must be addressed to ensure long-term viability. Addressing these challenges will be crucial for maintaining the efficacy and availability of penicillin in modern healthcare, as well as ensuring its continued relevance in future medical practices.
Research and Development of New Penicillins
The research and development of new penicillins have been pivotal in combating bacterial infections, particularly in the face of rising antibiotic resistance. Since the discovery of penicillin by Alexander Fleming in 1928, scientists have continuously sought to enhance its efficacy and broaden its spectrum of activity. Modern research focuses on several key areas: improving stability, enhancing bioavailability, and expanding the range of target bacteria. One significant advancement is the development of beta-lactamase inhibitors, which protect penicillins from degradation by bacterial enzymes. This has led to the creation of combination therapies like amoxicillin-clavulanate (Augmentin) and piperacillin-tazobactam (Zosyn), which are effective against a wider range of pathogens. Additionally, efforts to modify the chemical structure of penicillins have resulted in the synthesis of new generations such as carbenicillin and ticarcillin, which exhibit greater resistance to beta-lactamases. Another critical area of research involves optimizing delivery systems to improve bioavailability and reduce side effects. For instance, the development of extended-release formulations allows for less frequent dosing, enhancing patient compliance. Furthermore, research into novel routes of administration, such as inhalation for respiratory infections or topical application for skin infections, aims to target specific infection sites more effectively. Genomic and proteomic studies have also played a crucial role in understanding bacterial resistance mechanisms at a molecular level. This knowledge enables the design of new penicillins that can evade or inhibit these resistance pathways. For example, the discovery of new targets within bacterial cell walls has led to the development of drugs like mecillinam, which targets the bacterial enzyme MurA. Future directions in penicillin research include leveraging advanced technologies such as artificial intelligence and machine learning to predict and design novel antibiotic structures. Moreover, collaborative efforts between academia, industry, and regulatory bodies are essential for accelerating the development and approval of new antibiotics. The integration of antimicrobial stewardship programs alongside R&D initiatives is also vital to ensure that these new penicillins are used judiciously to combat resistance. In summary, the ongoing research and development of new penicillins are driven by the need to stay ahead of bacterial resistance. By combining innovative chemistry, advanced delivery systems, and cutting-edge biological insights, scientists are working towards creating more effective and sustainable antibiotic therapies for the future. These advancements are crucial for maintaining the efficacy of penicillins as a cornerstone in modern medicine's arsenal against bacterial infections.