What Does Dna Ligase Do
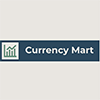
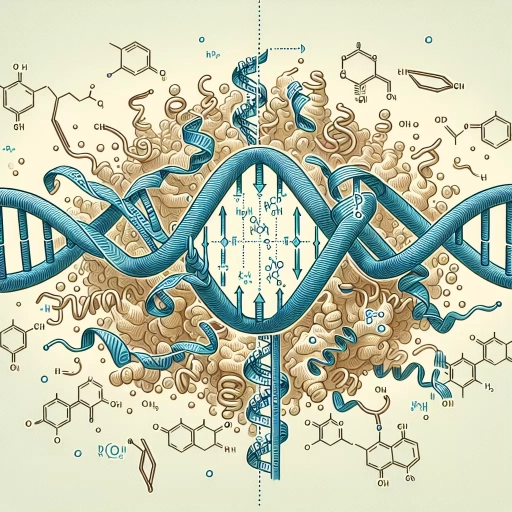
DNA ligase is a crucial enzyme that plays a pivotal role in various biological processes, including DNA replication, repair, and genetic engineering. This enzyme is responsible for forming phosphodiester bonds between the 5' phosphate end of one nucleotide and the 3' hydroxyl end of another, effectively sealing gaps in DNA strands. In DNA replication, DNA ligase ensures the continuity of the newly synthesized DNA strand by linking Okazaki fragments together. During DNA repair, it helps mend breaks in the DNA molecule, maintaining genomic integrity. Additionally, in genetic engineering, DNA ligase is instrumental in cloning and constructing recombinant DNA molecules. Understanding the multifaceted functions of DNA ligase is essential for appreciating its significance in these critical biological processes. This article will delve into the role of DNA ligase in these contexts, starting with its indispensable role in DNA replication.
Role of DNA Ligase in DNA Replication
DNA ligase plays a crucial role in the process of DNA replication, ensuring the integrity and continuity of the genetic material. This enzyme is essential for several key functions during replication. Firstly, DNA ligase is responsible for sealing the gaps between Okazaki fragments, which are short segments of DNA synthesized on the lagging strand. This process is vital for maintaining the continuity of the DNA strand. Secondly, DNA ligase helps in maintaining genome stability by repairing any breaks or gaps in the DNA, thereby preventing mutations and genetic disorders. Lastly, it ensures accurate DNA synthesis by forming phosphodiester bonds between nucleotides, which is critical for the fidelity of genetic information. Understanding these roles highlights the importance of DNA ligase in ensuring that DNA replication is both accurate and efficient. This article will delve into these critical functions, starting with the essential task of sealing gaps between Okazaki fragments.
Sealing Gaps Between Okazaki Fragments
During DNA replication, the process of synthesizing new DNA strands involves the creation of short, discontinuous segments known as Okazaki fragments on the lagging strand. These fragments are generated because DNA polymerase can only synthesize DNA in one direction, from 5' to 3', but the replication fork moves in the opposite direction on the lagging strand. As a result, multiple Okazaki fragments are produced, each approximately 1000-2000 nucleotides long in eukaryotes and 1000-2000 nucleotides in prokaryotes. However, these fragments are not yet part of a continuous DNA strand; they are separated by small gaps where the RNA primers were removed. To seal these gaps and form a continuous strand, DNA ligase plays a crucial role. DNA ligase is an enzyme responsible for joining the 3'-hydroxyl end of one Okazaki fragment to the 5'-phosphate end of the adjacent fragment. This process involves forming a phosphodiester bond between the two ends, effectively sealing the gap and creating a continuous DNA strand. The enzyme catalyzes this reaction by using energy from ATP or NAD+ to drive the ligation process. In eukaryotic cells, DNA ligase I is primarily involved in this step of DNA replication, while in prokaryotic cells, DNA ligase (also known as E. coli DNA ligase) performs this function. The sealing of gaps between Okazaki fragments is essential for maintaining genome integrity. Without this process, the newly synthesized DNA would remain fragmented, leading to genetic instability and potentially harmful mutations. DNA ligase ensures that the genetic material is accurately replicated and that the new DNA strands are complete and continuous, ready for subsequent processes such as DNA repair and transcription. In summary, the role of DNA ligase in sealing gaps between Okazaki fragments is pivotal for the completion of DNA replication. By forming phosphodiester bonds between adjacent fragments, DNA ligase ensures that the lagging strand is converted into a continuous, intact DNA strand, thereby safeguarding genetic integrity and facilitating accurate transmission of genetic information from one generation of cells to the next. This enzymatic activity underscores the critical importance of DNA ligase in maintaining the structural coherence of DNA during replication.
Maintaining Genome Stability
Maintaining genome stability is crucial for the survival and proper functioning of cells. This stability ensures that genetic information is accurately replicated and passed on to daughter cells, preventing mutations and genomic rearrangements that could lead to diseases such as cancer. Several mechanisms are in place to achieve this stability, including DNA repair pathways, checkpoint controls, and precise DNA replication processes. DNA ligase plays a pivotal role in these processes by sealing the gaps between Okazaki fragments during lagging strand synthesis in DNA replication. This enzyme catalyzes the formation of a phosphodiester bond between the 3'-hydroxyl group of one nucleotide and the 5'-phosphate group of another, effectively joining the fragments together to form a continuous strand. Without DNA ligase, these gaps would remain, leading to incomplete DNA replication and potential genomic instability. Additionally, DNA ligase is involved in various DNA repair mechanisms, such as base excision repair and non-homologous end joining, where it helps to restore the integrity of damaged DNA by sealing breaks in the DNA backbone. By ensuring that DNA is accurately replicated and repaired, DNA ligase contributes significantly to maintaining genome stability, thereby safeguarding cellular health and function. This underscores the critical importance of DNA ligase in preserving the genetic blueprint of an organism.
Ensuring Accurate DNA Synthesis
Ensuring accurate DNA synthesis is a critical process in cellular biology, particularly during DNA replication. This precision is essential to maintain the integrity of genetic information and prevent mutations that could lead to genetic disorders or cancer. Several mechanisms and enzymes work in concert to achieve this accuracy. One key player is DNA polymerase, which synthesizes new DNA strands by adding nucleotides to the growing chain. DNA polymerase has a proofreading capability that allows it to correct errors as it synthesizes DNA, ensuring a high degree of fidelity. Another crucial enzyme involved in this process is DNA ligase. While DNA polymerase synthesizes DNA in short segments called Okazaki fragments on the lagging strand, DNA ligase seals the gaps between these fragments by forming a phosphodiester bond. This sealing process is vital because it ensures that the newly synthesized DNA is continuous and free from breaks, which could otherwise lead to genetic instability. Additionally, the process of mismatch repair further enhances the accuracy of DNA synthesis. This pathway corrects mismatches that may have been missed by DNA polymerase's proofreading function. Enzymes such as MutS and MutL recognize and bind to mismatched bases, initiating a repair process that corrects the error. The interplay between these mechanisms—DNA polymerase's synthesis and proofreading, DNA ligase's sealing of gaps, and mismatch repair's correction of errors—ensures that DNA synthesis is highly accurate. This accuracy is paramount for maintaining genome stability and preventing harmful mutations that could disrupt cellular function. In summary, accurate DNA synthesis relies on a combination of precise enzymatic activities, including those of DNA polymerase, DNA ligase, and mismatch repair enzymes. These processes work together to ensure that genetic information is replicated faithfully, thereby safeguarding the integrity of the genome.
Function of DNA Ligase in DNA Repair
DNA ligase plays a crucial role in the intricate process of DNA repair, ensuring the integrity and stability of genetic material. This enzyme is essential for sealing gaps in the DNA backbone, a function that is vital in three key areas: repairing single-strand breaks, fixing double-strand breaks, and maintaining chromosomal integrity. When single-strand breaks occur, DNA ligase seals the nick in the phosphodiester backbone, restoring the continuity of the DNA strand. In the case of double-strand breaks, DNA ligase is part of a more complex repair process that involves other enzymes to rejoin the severed strands. Additionally, by maintaining chromosomal integrity, DNA ligase helps prevent genetic mutations and ensures that cellular processes proceed accurately. Understanding these functions highlights the importance of DNA ligase in safeguarding the genome. This article will delve into these critical roles, starting with the process of repairing single-strand breaks.
Repairing Single-Strand Breaks
Repairing single-strand breaks is a critical process in maintaining genomic integrity, and DNA ligase plays a pivotal role in this mechanism. Single-strand breaks occur frequently due to various factors such as oxidative stress, ionizing radiation, and errors during DNA replication. When a single-strand break happens, the cell's repair machinery is activated to restore the continuity of the DNA strand. The process involves several key enzymes, with DNA ligase being the final and essential component. First, the damaged region is recognized by sensors that trigger the recruitment of repair proteins. The enzyme DNA polymerase then fills in any gaps by synthesizing new nucleotides, while another enzyme, DNA helicase, unwinds the DNA to facilitate access to the damaged area. However, these enzymes cannot seal the break on their own; they leave behind a nick—a small gap where the phosphate backbone is interrupted. Here is where DNA ligase steps in. This enzyme catalyzes the formation of a phosphodiester bond between the 5' phosphate group of one nucleotide and the 3' hydroxyl group of another, effectively sealing the nick and restoring the integrity of the DNA strand. This reaction requires energy, which is typically provided by ATP or NAD+ in eukaryotes and prokaryotes, respectively. The precision and efficiency of DNA ligase are crucial because any misrepair can lead to mutations or genomic instability. In eukaryotic cells, there are multiple forms of DNA ligase (Ligase I, III, and IV), each specialized for different types of repair processes such as base excision repair (BER), nucleotide excision repair (NER), and non-homologous end joining (NHEJ). For instance, Ligase I is involved in BER and NER pathways, while Ligase IV is essential for NHEJ. In summary, repairing single-strand breaks is a complex but highly coordinated process that relies on the precise action of DNA ligase to ensure genomic stability. By sealing nicks in the DNA backbone, DNA ligase completes the repair cycle initiated by other enzymes, thereby protecting cells from genetic damage and maintaining the integrity of their genetic material. This underscores the vital function of DNA ligase in DNA repair mechanisms and highlights its importance in preserving cellular health.
Fixing Double-Strand Breaks
Fixing double-strand breaks is a critical process in maintaining genomic integrity, and it involves several key steps and enzymes, including DNA ligase. Double-strand breaks (DSBs) are severe DNA lesions where both strands of the double helix are severed, posing a significant threat to cellular survival. Cells employ two main pathways to repair DSBs: non-homologous end joining (NHEJ) and homologous recombination (HR). In NHEJ, the broken ends are directly joined together without the need for a template. This process involves the Ku70/Ku80 heterodimer, which binds to the broken ends, followed by the recruitment of DNA-dependent protein kinase (DNA-PK). The DNA-PK complex then activates other factors such as Artemis, which trims the ends if necessary, and DNA polymerases that fill in any gaps. Finally, DNA ligase IV seals the break by forming a phosphodiester bond between the 5' phosphate and 3' hydroxyl groups of the adjacent nucleotides. In contrast, HR is a more accurate but more complex process that requires a template with sequence homology to the damaged region. This pathway is typically active during the S and G2 phases of the cell cycle when sister chromatids are available as templates. HR involves the recognition of the break by the MRN complex (Mre11/Rad50/Nbs1), followed by the resection of one strand to create a 3' single-strand overhang. This overhang is then coated with RPA protein and later replaced by Rad51, facilitating strand invasion into the sister chromatid. After DNA synthesis extends the invaded strand, the resulting structure is resolved through either non-crossover or crossover recombination. Here again, DNA ligase plays a crucial role in sealing any remaining gaps after resolution. DNA ligase is essential in both pathways for its ability to form covalent bonds between nucleotides, thereby restoring the continuity of the DNA molecule. In NHEJ, DNA ligase IV is specifically adapted for this function due to its association with XRCC4, which enhances its activity on blunt or nearly blunt ends. In HR, while the primary sealing is often performed by other ligases like DNA ligase I or III, the final steps may still involve these enzymes to ensure complete repair. In summary, fixing double-strand breaks is a multifaceted process that relies heavily on precise enzymatic activities, with DNA ligase being a key player in both NHEJ and HR pathways. Its role in forming phosphodiester bonds is indispensable for maintaining genomic stability and preventing mutations or chromosomal rearrangements that could lead to cellular dysfunction or disease.
Maintaining Chromosomal Integrity
Maintaining chromosomal integrity is crucial for the survival and proper functioning of cells. This process involves a complex interplay of various molecular mechanisms, including DNA repair pathways, to ensure that genetic material remains intact and accurate. One key enzyme in these pathways is DNA ligase, which plays a pivotal role in sealing the gaps between DNA fragments during repair processes. Chromosomal integrity can be compromised by various factors such as environmental mutagens, errors during DNA replication, and oxidative stress, leading to DNA breaks and mutations. To mitigate these damages, cells employ several repair mechanisms including base excision repair (BER), nucleotide excision repair (NER), mismatch repair (MMR), and double-strand break repair via non-homologous end joining (NHEJ) or homologous recombination (HR). In all these pathways, DNA ligase is essential for the final step of sealing the phosphodiester backbone of DNA, thereby restoring the continuity of the genetic material. During BER, for instance, DNA ligase seals the nick created after the removal of a damaged base and its subsequent repair. In NER, it joins the newly synthesized DNA strand to the existing one after the excision of a damaged segment. In MMR, DNA ligase completes the repair by sealing any gaps left after correcting mismatched bases. For double-strand breaks, whether repaired through NHEJ or HR, DNA ligase is indispensable for forming a stable phosphodiester bond between the ends of the broken DNA strands. The function of DNA ligase in these contexts underscores its importance in maintaining chromosomal stability. Without efficient ligation, DNA breaks would persist, leading to genomic instability and potentially triggering cell cycle arrest, apoptosis, or even cancer. The precision and fidelity of DNA ligase ensure that the genetic code remains unaltered and functional, thereby safeguarding cellular homeostasis and preventing harmful mutations from being passed on to daughter cells. In summary, maintaining chromosomal integrity relies heavily on the accurate and efficient sealing of DNA breaks by DNA ligase. This enzyme's role in various DNA repair pathways highlights its critical contribution to genomic stability and overall cellular health. By ensuring that DNA is properly joined and repaired, DNA ligase helps protect against genetic damage and supports the long-term viability of cells.
Importance of DNA Ligase in Genetic Engineering
DNA ligase is a crucial enzyme in genetic engineering, playing a pivotal role in various biotechnological applications. Its importance is underscored in three key areas: cloning and recombinant DNA technology, gene editing with CRISPR/Cas9, and the construction of plasmids and vectors. In cloning and recombinant DNA technology, DNA ligase facilitates the joining of DNA fragments, enabling the creation of recombinant DNA molecules that can be replicated within host organisms. This process is fundamental for producing large quantities of specific genes or proteins. Gene editing techniques, such as CRISPR/Cas9, also rely on DNA ligase to repair DNA breaks and integrate new genetic material. Additionally, in the construction of plasmids and vectors, DNA ligase is essential for assembling these genetic vehicles that carry foreign DNA into cells. By seamlessly connecting DNA strands, DNA ligase ensures the integrity and functionality of these genetic constructs. Transitioning to the specifics of cloning and recombinant DNA technology, it becomes clear that DNA ligase is the linchpin that makes these complex processes feasible and efficient.
Cloning and Recombinant DNA Technology
Cloning and recombinant DNA technology are cornerstone techniques in genetic engineering, enabling scientists to manipulate and study genetic material with unprecedented precision. These technologies involve the creation of recombinant DNA molecules, where DNA from different sources is combined, and the subsequent cloning of these molecules to produce multiple copies. The process begins with the isolation of DNA fragments using restriction enzymes, which cut DNA at specific sequences. These fragments are then joined together using DNA ligase, an enzyme that seals the gaps between the fragments by forming phosphodiester bonds. This step is crucial because it allows the creation of a cohesive, functional DNA molecule that can be replicated and expressed in a host organism. Once the recombinant DNA molecule is constructed, it is introduced into a host cell, such as a bacterium or yeast, through a process known as transformation. The host cell then replicates the recombinant DNA along with its own genome, effectively cloning the inserted DNA. This cloned DNA can be used for various purposes, including the production of therapeutic proteins, vaccines, and other valuable biological products. For instance, insulin for diabetes treatment is produced using recombinant DNA technology, where the human insulin gene is inserted into bacterial cells that then produce insulin. The importance of DNA ligase in this process cannot be overstated. Without DNA ligase, the fragments of DNA would remain separate and unable to form a stable, functional molecule. DNA ligase ensures that the recombinant DNA is properly sealed, allowing it to be replicated and expressed correctly. This enzyme's role is pivotal in ensuring the integrity and stability of the recombinant DNA molecule, making it possible to achieve the desired genetic modifications and produce large quantities of genetically engineered products. In summary, cloning and recombinant DNA technology rely heavily on DNA ligase to facilitate the precise and efficient assembly of genetic material, thereby enabling a wide range of applications in biotechnology and medicine.
Gene Editing and CRISPR/Cas9
Gene editing, particularly through the CRISPR/Cas9 system, has revolutionized the field of genetic engineering by offering unprecedented precision and efficiency in modifying DNA sequences. CRISPR/Cas9 leverages a naturally occurring bacterial defense mechanism to target and edit specific genes. The process involves the guide RNA (gRNA) locating a specific sequence within the genome, which is then cleaved by the Cas9 enzyme. This double-stranded break triggers the cell's repair machinery, allowing researchers to introduce desired changes by providing a template for repair. However, the integration of these changes relies heavily on DNA ligase, an enzyme crucial for sealing the gaps in DNA strands during the repair process. DNA ligase plays a pivotal role in ensuring that the edited DNA is properly joined, thereby maintaining genomic integrity. Without DNA ligase, the breaks induced by CRISPR/Cas9 would remain unrepaired, leading to genomic instability and potentially harmful mutations. In the context of CRISPR/Cas9, DNA ligase facilitates both non-homologous end joining (NHEJ) and homologous recombination (HR), two primary pathways through which cells repair DNA breaks. NHEJ is a more error-prone process but is often faster, while HR is more precise but requires a template with homology to the broken region. By ensuring that these repair processes are completed accurately, DNA ligase is essential for the successful implementation of gene editing strategies. Moreover, the efficiency and accuracy of CRISPR/Cas9-mediated gene editing can be significantly enhanced by optimizing conditions that favor the activity of DNA ligase. For instance, providing an exogenous template for HR can increase the likelihood of precise editing outcomes. Additionally, understanding the mechanisms and regulation of DNA ligase activity can help in developing strategies to improve the specificity and efficiency of gene editing. In summary, while CRISPR/Cas9 is the tool that enables precise targeting and cleavage of DNA, DNA ligase is the enzyme that ensures the seamless integration of edited sequences into the genome. The importance of DNA ligase in genetic engineering cannot be overstated; it is a critical component that bridges the gap between the initial DNA break and the final repaired product, making gene editing a reliable and powerful tool for research and therapeutic applications.
Construction of Plasmids and Vectors
In the realm of genetic engineering, the construction of plasmids and vectors is a crucial step that relies heavily on the enzyme DNA ligase. Plasmids and vectors are circular or linear DNA molecules designed to carry genetic material into host cells, such as bacteria, yeast, or mammalian cells. The process begins with the selection of a suitable vector, which can be a plasmid, virus, or other vehicle capable of transporting DNA. Once the vector is chosen, it is cut open using restriction enzymes that recognize specific DNA sequences and cleave the DNA at those sites. This creates sticky ends or blunt ends, depending on the enzyme used. The next step involves inserting the desired DNA fragment into the vector. This fragment is typically prepared by cutting the donor DNA with the same restriction enzymes used to cut the vector, ensuring compatible sticky ends. The DNA fragment is then mixed with the cut vector in a reaction buffer. Here, DNA ligase plays its pivotal role by sealing the gaps between the DNA fragments and the vector, forming phosphodiester bonds. This enzyme catalyzes the covalent joining of the 5' phosphate group of one nucleotide to the 3' hydroxyl group of another, effectively closing the gaps and reconstituting the circular or linear structure of the vector. The ligation reaction is often performed under optimal conditions, such as the right temperature and pH, to maximize the efficiency of DNA ligase. After ligation, the recombinant plasmids or vectors are introduced into host cells through a process known as transformation or transfection. Once inside the host cells, these recombinant vectors can replicate and express the inserted genes, allowing for various applications in biotechnology, medicine, and research. The importance of DNA ligase in this process cannot be overstated; without it, the construction of functional plasmids and vectors would be impossible. DNA ligase ensures that the genetic material is accurately and stably integrated into the vector, enabling precise genetic manipulation and expression. This precision is critical for ensuring that the desired traits are expressed correctly and consistently in the host organism, making DNA ligase an indispensable tool in genetic engineering.