What Does Hydro Include
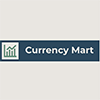
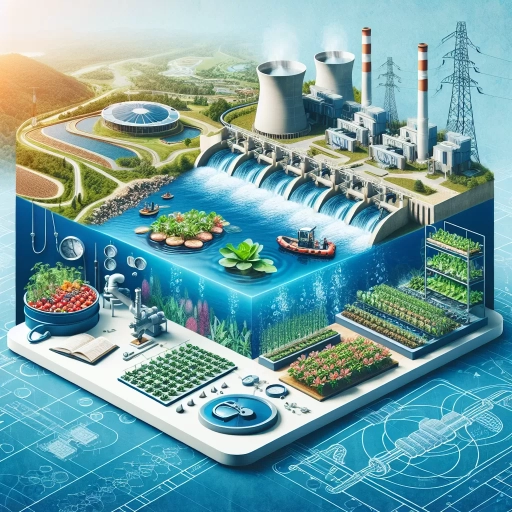
Hydro, short for hydroelectric power, is a cornerstone of renewable energy that harnesses the force of water to generate electricity. This clean and sustainable source of power has been a vital component of global energy production for over a century. In this article, we will delve into the multifaceted world of hydroelectricity, exploring its definition and scope, the intricate components and processes involved in hydro systems, and the significant environmental and economic impacts it has on our planet. Understanding the fundamentals of hydroelectric power is crucial for appreciating its role in the energy landscape. By examining the definition and scope of hydroelectricity, we set the stage for a deeper analysis of its operational mechanisms and broader implications. This comprehensive approach will provide readers with a holistic view of hydroelectric power, from its basic principles to its far-reaching effects on both the environment and the economy. Let us begin by understanding hydro: its definition and scope.
Understanding Hydro: Definition and Scope
Hydro, a term often associated with water and its various applications, encompasses a broad spectrum of scientific, historical, and industrial contexts. To fully grasp the concept of hydro, it is essential to delve into its multifaceted nature. This article will explore the historical context of hydro, tracing its evolution from ancient civilizations to modern times. It will also provide a scientific definition, clarifying the technical aspects and principles that underpin hydro-related phenomena. Additionally, we will examine the industrial applications of hydro, highlighting how it is harnessed in various sectors such as energy production, agriculture, and engineering. By understanding these three key dimensions—historical context, scientific definition, and industrial applications—we can gain a comprehensive insight into the definition and scope of hydro. This article aims to provide a detailed and engaging exploration of these aspects, ultimately leading to a deeper understanding of hydro: its definition and scope.
Historical Context of Hydro
The historical context of hydroelectric power is a rich and transformative narrative that spans centuries, reflecting human ingenuity and the relentless pursuit of harnessing nature's energy. The earliest recorded use of hydro power dates back to ancient civilizations, where waterwheels were employed for milling grain and performing other mechanical tasks. In ancient Greece and Rome, these primitive hydro machines were integral to agricultural and industrial processes, laying the groundwork for future innovations. The Industrial Revolution marked a significant turning point in the history of hydroelectricity. As demand for power surged with the advent of mechanized manufacturing, engineers began exploring ways to convert the kinetic energy of water into electrical energy. In 1832, French engineer Benoit Fourneyron invented the first practical water turbine, which could generate mechanical power efficiently. This breakthrough paved the way for the development of hydroelectric generators. The late 19th century saw the first successful experiments in generating electricity from water. In 1882, William Armstrong demonstrated a hydroelectric system in Cragside, England, illuminating a house using water from a lake. This pioneering work was soon followed by the construction of the first commercial hydroelectric plant in Appleton, Wisconsin, USA in 1882 by H.J. Rogers. The plant harnessed the power of the Fox River to light a paper mill and a nearby residence. The early 20th century witnessed a rapid expansion of hydroelectric power generation as technology improved and demand for electricity grew exponentially. The construction of large dams like the Hoover Dam in the United States and the Aswan Dam in Egypt became iconic symbols of human engineering prowess. These mega-projects not only provided immense power but also transformed landscapes and communities. Throughout the 20th century, advancements in materials science, turbine design, and transmission technologies further enhanced the efficiency and reach of hydroelectric power. Today, hydroelectricity remains one of the cleanest and most reliable forms of renewable energy globally. It accounts for a significant portion of electricity generation worldwide, particularly in regions with abundant water resources such as Norway, Brazil, and Canada. Understanding this historical context is crucial for appreciating the scope and definition of hydroelectric power today. From its humble beginnings with ancient waterwheels to its current status as a cornerstone of renewable energy, hydroelectricity has evolved through centuries of innovation driven by human necessity and ingenuity. This evolution underscores not only the importance of hydroelectric power but also its potential to continue shaping our energy future sustainably.
Scientific Definition of Hydro
**Understanding Hydro: Definition and Scope** ### Scientific Definition of Hydro Hydro, in its scientific context, encompasses a broad spectrum of disciplines and phenomena related to water. The term "hydro" is derived from the Greek word "hydor," meaning water, and it forms the basis for various scientific fields that study the properties, behavior, and applications of water. At its core, hydrology—the primary discipline under the hydro umbrella—focuses on the Earth's water resources, including their distribution, circulation, and physical and chemical properties. This includes understanding precipitation patterns, runoff processes, groundwater dynamics, and the interactions between water and the environment. In a more specialized sense, hydro can refer to hydrodynamics, which is the study of fluids in motion. This branch delves into the mechanics of water flow, pressure, and energy transfer within aquatic systems such as rivers, oceans, and lakes. Hydrodynamics is crucial for engineering applications like dam design, irrigation systems, and oceanographic research. Another significant aspect is hydrochemistry, which examines the chemical composition of water bodies. This field is essential for understanding water quality, pollution dynamics, and the geochemical cycles that affect aquatic ecosystems. Hydrochemistry informs strategies for water treatment, conservation, and environmental monitoring. Additionally, hydrogeology explores the occurrence, movement, and quality of groundwater. This discipline is vital for managing groundwater resources sustainably, assessing aquifer health, and mitigating issues related to groundwater contamination. The scope of hydro also extends to hydroelectricity, which involves harnessing the energy potential of moving water to generate electricity. This renewable energy source is a key component of sustainable energy strategies globally. Furthermore, hydrobiology investigates the biological aspects of aquatic systems, including the diversity of aquatic life and the ecological interactions within these environments. This knowledge is critical for conservation efforts, fisheries management, and maintaining ecosystem balance. In summary, the scientific definition of hydro encompasses a multifaceted approach to understanding water in all its forms and functions. From hydrology and hydrodynamics to hydrochemistry, hydrogeology, hydroelectricity, and hydrobiology, each discipline contributes to a comprehensive understanding of water's role in our planet's systems and its importance for human society and the environment. This integrated perspective is essential for addressing global challenges related to water resources, environmental sustainability, and human well-being.
Industrial Applications of Hydro
Industrial applications of hydro power are multifaceted and pivotal, underscoring its significance in modern energy landscapes. Hydroelectric power, generated from the movement of water in rivers, oceans, or man-made reservoirs, is a clean and renewable energy source that plays a crucial role in meeting global energy demands. One of the primary industrial applications is electricity generation. Hydroelectric plants harness the kinetic energy of water to drive turbines, which in turn generate electricity. This method is not only environmentally friendly but also highly efficient, with some hydroelectric plants achieving efficiency rates of up to 90%, far surpassing the efficiency of thermal power plants. The reliability and predictability of hydroelectric power make it an essential component of grid stability, allowing for better management of peak demand periods. Beyond electricity generation, hydro power has various other industrial applications. For instance, pumped storage hydroelectricity serves as a form of energy storage. During off-peak hours, excess energy is used to pump water from a lower reservoir to an upper reservoir. When demand increases, the water is released back down through turbines to generate electricity, providing a buffer against sudden spikes in energy demand. This technology is vital for maintaining grid balance and ensuring a stable supply of electricity. In addition to power generation and storage, hydro power is used in irrigation systems, particularly in agricultural sectors. Hydroelectric dams can regulate water flow, providing a consistent supply of water for crops during dry seasons. This application is crucial for food security and economic stability in regions heavily reliant on agriculture. Moreover, hydro power supports industrial processes directly. For example, in aluminum smelting, hydroelectric power is often preferred due to its high energy density and low operating costs. The electrolysis process required for aluminum production is highly energy-intensive, making hydroelectric power an ideal choice. Furthermore, hydroelectric facilities can also serve as flood control mechanisms and water supply systems. By managing water levels and flow rates, these facilities help mitigate the risks associated with flooding and ensure a steady supply of clean water for both industrial and domestic use. In summary, the industrial applications of hydro power are diverse and critical. From electricity generation and energy storage to irrigation and industrial processes, hydro power contributes significantly to economic development while offering a sustainable alternative to fossil fuels. Its role in maintaining grid stability, supporting agriculture, and facilitating various industrial processes underscores its importance in the global transition towards renewable energy sources. As the world continues to seek cleaner and more reliable forms of energy, the significance of hydro power will only continue to grow.
Components and Processes of Hydro Systems
Hydro systems, a cornerstone of renewable energy, harness the power of water to generate electricity, providing a sustainable and reliable source of energy. These systems are complex, involving multiple components and processes that work in harmony to convert the kinetic energy of water into electrical power. At the heart of any hydro system lies the water source, which can be collected through various methods such as dams, run-of-river systems, and pumped storage facilities. The energy conversion process is crucial, as it involves the use of turbines and generators to transform the mechanical energy of moving water into electrical energy. Additionally, the infrastructure and maintenance requirements are significant, ensuring that these systems operate efficiently and safely over their lifespan. Understanding these key aspects—water sources and collection methods, energy conversion and generation, and infrastructure and maintenance requirements—is essential for grasping the full scope of hydro systems. This article will delve into each of these components in detail, providing a comprehensive overview that transitions seamlessly into understanding hydro: definition and scope.
Water Sources and Collection Methods
Water sources and collection methods are crucial components of hydro systems, ensuring the efficient and sustainable management of this vital resource. Hydro systems, which encompass various technologies and processes for harnessing, treating, and distributing water, rely heavily on the availability and quality of water sources. These sources can be broadly categorized into surface water and groundwater. Surface water includes rivers, lakes, reservoirs, and wetlands, which are often more accessible but can be subject to seasonal variations and environmental impacts. Groundwater, on the other hand, is stored beneath the Earth's surface in aquifers and can provide a more consistent supply but requires careful management to avoid depletion. The collection of water from these sources involves several methods tailored to the specific characteristics of each source. For surface water, common collection methods include damming rivers to create reservoirs, constructing intake structures to draw water directly from rivers or lakes, and using canals or pipelines to transport the collected water to treatment facilities. In contrast, groundwater collection typically involves drilling wells or boreholes into aquifers, followed by the installation of pumps to extract the water. Rainwater harvesting is another method that collects and stores rainwater from rooftops and other surfaces for non-potable uses such as irrigation and toilet flushing. Advanced technologies are increasingly being integrated into these traditional methods to enhance efficiency and sustainability. For instance, remote sensing and satellite imaging can help identify optimal locations for groundwater wells by analyzing geological formations and soil moisture levels. Additionally, smart sensors and IoT devices can monitor water quality in real-time, enabling prompt responses to any changes or contaminants detected in the water supply. Effective water collection also involves considerations of environmental impact and community engagement. Hydro systems must be designed with minimal disruption to natural ecosystems and with respect for local communities' rights to access clean water. This includes implementing measures to protect aquatic habitats during dam construction and ensuring that groundwater extraction does not lead to land subsidence or saltwater intrusion. In summary, the selection and collection of water sources are foundational elements of hydro systems, requiring a balanced approach that considers both technical feasibility and environmental sustainability. By leveraging a mix of traditional methods and cutting-edge technologies, hydro systems can ensure reliable access to clean water while preserving natural resources for future generations. This holistic approach underscores the importance of integrated water management within the broader context of hydro systems.
Energy Conversion and Generation
Energy conversion and generation are pivotal components in the broader context of hydro systems, which encompass a range of technologies and processes designed to harness the power of water. At its core, energy conversion in hydro systems involves transforming the kinetic energy of flowing water into electrical energy. This process begins with the capture of water flow, typically through dams or run-of-river systems, where the potential energy of the water is converted into kinetic energy as it flows downhill. The kinetic energy is then channeled towards turbines, which are mechanical devices that convert this energy into rotational energy. This rotational energy is subsequently transferred to generators, where it is converted into electrical energy through electromagnetic induction. The efficiency and effectiveness of this energy conversion process depend on several key factors, including the head (vertical distance) of the water flow, the volume of water available, and the design of the turbines and generators. Modern hydroelectric power plants often employ advanced technologies such as Francis turbines, Kaplan turbines, and Pelton wheels, each optimized for different water flow conditions to maximize energy output. Additionally, hydro systems can be integrated with other renewable energy sources and grid management systems to enhance stability and reliability. Beyond the technical aspects, hydro energy generation offers several environmental and economic benefits. Hydroelectric power is a clean source of energy, producing no greenhouse gas emissions during operation and thus contributing significantly to reducing carbon footprints. It also provides flood control, irrigation support, and recreational opportunities, making it a multifaceted resource. Economically, hydroelectric power can be a cost-effective option over the long term due to low operational costs once the initial infrastructure investment is made. However, hydro systems also face challenges such as geographical limitations, high upfront costs for dam construction, and potential environmental impacts such as altering ecosystems and displacing communities. Addressing these challenges requires careful planning, stakeholder engagement, and innovative solutions that balance energy needs with environmental and social considerations. In summary, energy conversion and generation in hydro systems represent a sophisticated interplay of engineering, technology, and environmental stewardship. By leveraging the natural flow of water to produce electricity, these systems not only contribute to global energy security but also offer a sustainable path forward in the quest for cleaner and more reliable power sources. As part of the broader components and processes of hydro systems, understanding these dynamics is crucial for optimizing performance, mitigating risks, and ensuring that hydroelectric power continues to play a vital role in meeting our energy demands.
Infrastructure and Maintenance Requirements
Infrastructure and maintenance are crucial components of hydro systems, ensuring the efficient and reliable operation of these complex networks. Hydroelectric power plants, for instance, require robust infrastructure to harness the energy potential of water. This includes dams, turbines, generators, and transmission lines. Dams must be constructed with high-quality materials and designed to withstand various environmental conditions such as floods and earthquakes. Turbines and generators need regular maintenance to optimize performance and extend their lifespan. This involves routine inspections, lubrication of moving parts, and replacement of worn-out components. In addition to the mechanical aspects, hydro systems also depend on sophisticated control systems and monitoring technologies. These systems enable real-time monitoring of water levels, flow rates, and equipment performance, allowing for swift intervention in case of anomalies. Advanced sensors and data analytics tools help predict maintenance needs, reducing downtime and enhancing overall system reliability. The transmission infrastructure is another critical element that requires meticulous maintenance. Power lines and substations must be regularly inspected for signs of wear or damage, such as corrosion or insulation degradation. Vegetation management around transmission lines is also essential to prevent power outages caused by tree branches or other foliage coming into contact with the lines. Furthermore, environmental considerations play a significant role in hydro system maintenance. Ensuring that the ecosystem surrounding the hydroelectric facility remains healthy is vital. This includes managing water quality, preserving aquatic habitats, and mitigating the impact of altered water flows on downstream ecosystems. Regular training for maintenance personnel is also indispensable. Technicians need to stay updated with the latest technologies and best practices to perform their duties effectively. This includes understanding safety protocols and adhering to industry standards to prevent accidents and ensure compliance with regulatory requirements. In summary, the infrastructure and maintenance requirements of hydro systems are multifaceted and demanding. By investing in robust infrastructure, advanced monitoring technologies, and comprehensive maintenance practices, hydroelectric facilities can operate efficiently, reliably, and sustainably over their lifespan. This not only ensures the continuous supply of renewable energy but also protects the environment and supports local communities dependent on these systems. Effective infrastructure and maintenance are thus foundational to the successful operation of hydro systems.
Environmental and Economic Impact of Hydro
Hydro power, one of the oldest and most reliable forms of renewable energy, has been a cornerstone in the global transition towards sustainable energy sources. The environmental and economic impact of hydro power is multifaceted, offering both significant benefits and challenges. On the environmental front, hydro power generation can mitigate greenhouse gas emissions and support biodiversity, yet it also poses risks such as altering ecosystems and displacing communities. Economically, hydro power plants can provide stable employment opportunities and stimulate local economies, but they also come with substantial upfront costs and potential long-term liabilities. This article delves into these complexities through three key sections: **Environmental Benefits and Challenges**, which explores the ecological implications of hydro power; **Economic Advantages and Disadvantages**, which examines the financial aspects; and **Case Studies and Real-World Examples**, which illustrates practical applications. By understanding these dimensions, readers will gain a comprehensive insight into the role of hydro power in our energy landscape. To fully appreciate these impacts, it is essential to begin with a clear understanding of **Understanding Hydro: Definition and Scope**.
Environmental Benefits and Challenges
The environmental benefits and challenges of hydroelectric power are multifaceted and significant, making it a crucial component of the broader discussion on the environmental and economic impact of hydro energy. On the positive side, hydroelectric power is one of the cleanest and most renewable forms of energy production. Unlike fossil fuels, hydroelectric plants do not emit greenhouse gases or other pollutants that contribute to climate change and air pollution. This makes hydroelectric power an essential tool in reducing carbon footprints and mitigating the adverse effects of global warming. Additionally, hydroelectric facilities can provide flood control, irrigation, and water supply benefits, enhancing overall water management and supporting agricultural activities. The reservoirs created by dams can also serve as recreational areas, boosting local economies through tourism. However, the environmental challenges associated with hydroelectric power cannot be overlooked. The construction of dams and reservoirs often disrupts natural habitats and ecosystems, leading to the displacement of wildlife and local communities. For instance, the alteration of river flows can impact fish migration patterns and aquatic biodiversity, necessitating careful planning and mitigation strategies to minimize these effects. Furthermore, large dams can have significant social impacts, particularly in regions where indigenous communities rely on rivers for their livelihoods. The reservoirs formed by dams can also lead to the loss of fertile land and cultural heritage sites, highlighting the need for thorough environmental impact assessments and community engagement before any project is undertaken. Despite these challenges, advancements in technology and policy frameworks are helping to mitigate the negative impacts while maximizing the benefits of hydroelectric power. Modern dam designs incorporate features such as fish ladders and spillways that help maintain natural river flows and support aquatic life. Moreover, there is a growing emphasis on smaller-scale hydro projects that have lower environmental footprints compared to large dams. These smaller projects can be more adaptable to local conditions and often involve greater community participation, ensuring that the benefits are shared equitably while minimizing the risks. In conclusion, the environmental benefits and challenges of hydroelectric power underscore the complexity of balancing energy needs with environmental stewardship. While hydroelectric power offers a clean and renewable source of energy, it requires careful planning, robust regulatory frameworks, and innovative technologies to ensure that its implementation is sustainable and equitable. By addressing these challenges proactively, we can harness the full potential of hydroelectric power to support both economic development and environmental protection.
Economic Advantages and Disadvantages
The economic advantages and disadvantages of hydroelectric power are multifaceted, influencing both local communities and the broader economy. On the positive side, hydroelectric power plants offer several economic benefits. Firstly, they provide a reliable source of renewable energy, reducing dependence on fossil fuels and mitigating the volatility of energy prices. This stability can attract businesses and industries that require consistent power supply, thereby boosting local economic growth. Additionally, hydroelectric projects often create significant employment opportunities during the construction phase and ongoing maintenance, contributing to job creation and income generation in rural areas where these projects are typically located. Furthermore, hydroelectric power is generally cheaper to produce compared to other forms of electricity generation once the initial investment in infrastructure is made, leading to lower operational costs and potentially lower consumer electricity rates. However, there are also substantial economic disadvantages associated with hydroelectric power. The initial investment required to build a hydroelectric dam is extremely high, involving substantial capital outlays for construction, land acquisition, and environmental mitigation measures. This high upfront cost can be a significant barrier to entry for many countries or regions with limited financial resources. Moreover, the construction of dams can lead to displacement of communities and loss of agricultural land, resulting in significant social and economic costs for affected populations. The alteration of natural water flows can also impact downstream fisheries and agriculture, potentially leading to economic losses in these sectors. Additionally, while hydroelectric power is renewable, it is not entirely without environmental costs; the creation of reservoirs can lead to methane emissions due to organic matter decomposition, which has economic implications in terms of carbon credits and compliance with environmental regulations. In summary, while hydroelectric power offers several economic advantages such as reliability, job creation, and lower operational costs, it also comes with significant economic drawbacks including high initial investment, community displacement, and potential impacts on downstream industries. Balancing these factors is crucial for maximizing the economic benefits of hydroelectric power while minimizing its negative impacts. This balance is essential for ensuring that hydroelectric projects contribute positively to both environmental sustainability and economic development.
Case Studies and Real-World Examples
Case studies and real-world examples are invaluable tools for understanding the environmental and economic impact of hydroelectric power. These practical illustrations provide concrete evidence of how hydroelectric projects can both benefit and challenge local ecosystems and economies. For instance, the Hoover Dam in the United States is a seminal case study that highlights the dual nature of hydroelectric power. On one hand, it has been a cornerstone of renewable energy production since its inception, generating enough electricity to power millions of homes. However, its construction also had significant environmental impacts, including altering natural water flows and disrupting habitats for various species. This dichotomy underscores the need for careful planning and mitigation strategies to minimize adverse effects while maximizing the benefits of hydroelectric power. Another compelling example is the Three Gorges Dam in China, which is one of the world's largest hydroelectric dams. While it has significantly reduced China's reliance on fossil fuels and provided substantial economic benefits through electricity generation and flood control, it has also led to the displacement of over a million people and raised concerns about water quality and biodiversity loss. These real-world examples emphasize the importance of conducting thorough environmental impact assessments and engaging in community outreach to ensure that the benefits of hydroelectric power are equitably distributed. In contrast, smaller-scale hydroelectric projects, such as those implemented in rural communities, can demonstrate more balanced outcomes. For example, community-based hydroelectric initiatives in Nepal have not only provided reliable electricity but also supported local economic development by powering small industries and improving agricultural productivity. These projects often involve local stakeholders in the planning process, ensuring that environmental concerns are addressed and benefits are shared equitably among community members. Furthermore, case studies from countries like Norway and Switzerland illustrate how hydroelectric power can be integrated into a broader renewable energy strategy with minimal environmental disruption. These nations have implemented robust regulatory frameworks and technological innovations that minimize the ecological footprint of hydroelectric projects while maximizing their economic benefits. In summary, case studies and real-world examples serve as critical benchmarks for evaluating the environmental and economic impact of hydroelectric power. They highlight the complexities involved in balancing energy production with environmental stewardship and economic development. By examining these examples, policymakers, engineers, and stakeholders can gain valuable insights into best practices for implementing sustainable hydroelectric projects that benefit both people and the planet.