What Is Nm
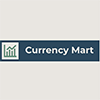
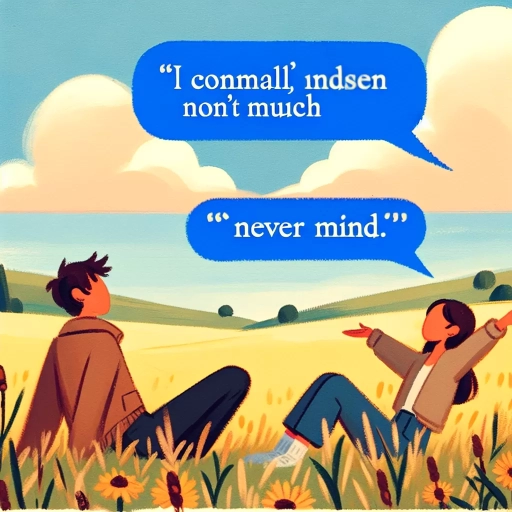
In the realm of advanced materials and technologies, Nanomaterials (NM) have emerged as a transformative force, revolutionizing various fields from medicine to electronics. These microscopic materials, engineered at the nanoscale, exhibit unique properties that distinguish them from their bulk counterparts. The significance of NM lies in their potential to enhance performance, efficiency, and functionality across diverse applications. This article delves into the multifaceted world of NM, beginning with **Understanding the Basics of NM**, where we explore the fundamental principles and characteristics that define these materials. We then examine **Applications and Uses of NM**, highlighting how they are being utilized in cutting-edge technologies and everyday products. Finally, we discuss **Future Prospects and Challenges of NM**, considering the potential breakthroughs and hurdles that lie ahead. By grasping these core aspects, readers will gain a comprehensive understanding of the role NM plays in shaping our future. Let's start by **Understanding the Basics of NM** to lay the groundwork for this insightful journey into the world of nanomaterials.
Understanding the Basics of NM
Understanding the basics of Network Management (NM) is crucial for anyone involved in the IT industry, as it forms the backbone of maintaining and optimizing network performance. This article delves into the fundamental aspects of NM, providing a comprehensive overview that is both informative and engaging. To grasp NM fully, it is essential to explore three key areas: its definition and origin, the key components and structure, and its historical context and evolution. Starting with the **Definition and Origin**, we will uncover how NM emerged as a critical discipline within the realm of computer networking. This section will clarify what NM entails and its significance in modern IT infrastructure. Next, we will examine the **Key Components and Structure** of NM, detailing the various tools, protocols, and methodologies that are integral to its operation. This will help readers understand how NM functions on a practical level. Finally, the **Historical Context and Evolution** of NM will be discussed, highlighting how it has developed over time to meet the changing needs of network technology. By understanding these three facets, readers will gain a holistic view of NM and its importance in today's digital landscape. Let's begin by exploring the **Definition and Origin** of Network Management, which sets the stage for a deeper understanding of this vital field.
Definition and Origin
**Understanding the Basics of NM: Definition and Origin** To delve into the fundamentals of "NM," it is crucial to first establish a clear definition and explore its origins. "NM" can stand for various terms depending on the context, but in many technical and scientific fields, it often refers to "nanometer," a unit of measurement in the International System of Units (SI). A nanometer is one billionth of a meter, or 10^-9 meters, making it an essential unit for describing dimensions at the atomic and molecular scale. The concept of the nanometer originates from the broader field of nanotechnology, which involves the manipulation of matter on an atomic, molecular, and supramolecular scale. The term "nanotechnology" was first coined by Japanese engineer Norio Taniguchi in 1974, although the idea of working at such small scales dates back to the 1950s and 1960s with pioneers like physicist Richard Feynman. Feynman's famous lecture "There's Plenty of Room at the Bottom," delivered in 1959, is often cited as a foundational moment in the development of nanotechnology. The nanometer as a unit of measurement became increasingly important as scientists and engineers began to explore and manipulate materials at the nanoscale. This scale is critical because it is here that many unique properties of materials emerge due to quantum effects and surface area considerations. For instance, nanoparticles exhibit different optical, electrical, and mechanical properties compared to their bulk counterparts, making them highly valuable in various applications such as medicine, electronics, and energy storage. Understanding the nanometer is essential for grasping how materials behave at this scale and how they can be engineered for specific purposes. For example, in medicine, nanoparticles are used in drug delivery systems to target specific cells or tissues with greater precision and efficacy. In electronics, nanoscale materials are used to create smaller, faster, and more efficient devices. The precision required to work at this scale necessitates a deep understanding of the nanometer as a fundamental unit of measurement. In summary, the definition of "NM" as a nanometer is rooted in the broader context of nanotechnology, which has evolved significantly since its conceptual beginnings in the mid-20th century. The nanometer's importance lies in its role as a critical unit for describing dimensions at the atomic and molecular level, enabling groundbreaking advancements across multiple scientific and technological disciplines. As research continues to push the boundaries of what is possible at the nanoscale, understanding this fundamental unit remains pivotal for innovation and progress.
Key Components and Structure
Understanding the basics of Network Management (NM) begins with grasping its key components and structure. At its core, NM is a systematic approach to managing, monitoring, and maintaining network resources to ensure optimal performance, reliability, and security. The structure of NM can be broken down into several critical components. **1. Network Monitoring:** This involves the continuous observation of network performance metrics such as bandwidth usage, latency, packet loss, and device status. Tools like Simple Network Management Protocol (SNMP) and network management systems (NMS) are essential for real-time monitoring. **2. Configuration Management:** This component focuses on managing the configuration of network devices to ensure consistency and compliance with organizational policies. It includes tasks such as device discovery, configuration backup, and change management. **3. Fault Management:** Identifying and resolving network issues promptly is crucial for maintaining uptime. Fault management includes alarm generation, event correlation, and root cause analysis to quickly diagnose and fix problems. **4. Performance Management:** This involves analyzing historical data to optimize network performance. It includes metrics like throughput, response times, and resource utilization to identify bottlenecks and areas for improvement. **5. Security Management:** Ensuring the security of the network is paramount. This component includes measures such as access control, intrusion detection, and vulnerability assessment to protect against unauthorized access and malicious activities. **6. Accounting Management:** Also known as billing or chargeback management, this component tracks resource usage for billing purposes or internal cost allocation. It helps in managing network resources efficiently by attributing costs to specific users or departments. The structure of NM typically follows a hierarchical model: - **Network Management Station (NMS):** The central console from which network administrators manage the network. - **Managed Devices:** These are the network elements such as routers, switches, servers, and workstations that are monitored and managed. - **Agents:** Software components running on managed devices that collect data and report back to the NMS. - **Management Information Base (MIB):** A database that stores information about the managed devices in a structured format. Effective NM also relies on standards and protocols like SNMP, which facilitates communication between the NMS and managed devices. By integrating these components into a cohesive structure, organizations can achieve better network reliability, improved performance, enhanced security, and reduced operational costs. Understanding these key components and their interplay is fundamental to mastering the basics of NM and leveraging its full potential in maintaining a robust and efficient network infrastructure.
Historical Context and Evolution
Understanding the basics of Network Management (NM) requires a deep dive into its historical context and evolution. The journey of NM began in the 1980s, a time when computer networks were in their infancy. As networks grew in complexity and size, the need for systematic management became paramount. The first significant milestone was the development of the Simple Network Management Protocol (SNMP) in 1988 by the Internet Engineering Task Force (IETF). SNMP provided a standardized framework for monitoring and managing network devices, marking the beginning of a structured approach to NM. In the early 1990s, the introduction of Remote Monitoring (RMON) further enhanced NM capabilities by allowing real-time monitoring of network segments. This period also saw the rise of network management systems like HP OpenView and IBM NetView, which integrated various tools for network monitoring, configuration, and troubleshooting. These systems were crucial in managing the burgeoning number of network devices and ensuring network reliability. The late 1990s and early 2000s witnessed significant advancements with the advent of more sophisticated NM tools. The emergence of network management frameworks such as FCAPS (Fault, Configuration, Accounting, Performance, and Security) provided a comprehensive model for managing networks holistically. This framework ensured that all aspects of network operations were covered, from fault detection to security management. The 21st century brought about a new era in NM with the integration of technologies like IP version 6 (IPv6), Software-Defined Networking (SDN), and Network Functions Virtualization (NFV). These technologies introduced greater flexibility and scalability, enabling networks to adapt dynamically to changing demands. The rise of cloud computing and virtualization also necessitated the development of cloud-based NM solutions that could manage distributed and virtualized environments efficiently. In recent years, the evolution of NM has been influenced by advancements in artificial intelligence (AI), machine learning (ML), and big data analytics. Modern NM systems leverage these technologies to predict network issues before they occur, optimize performance in real-time, and enhance security through anomaly detection. The Internet of Things (IoT) has further expanded the scope of NM, requiring solutions that can manage a vast number of interconnected devices. Today, NM is not just about monitoring and troubleshooting but also about proactive management and optimization. The integration of automation tools like Ansible and Puppet has streamlined network configuration and deployment processes, reducing human error and increasing efficiency. Additionally, the adoption of DevOps practices has bridged the gap between network operations and development teams, fostering a culture of continuous improvement and collaboration. In conclusion, understanding the historical context and evolution of NM is essential for appreciating its current state and future directions. From its humble beginnings with SNMP to the sophisticated AI-driven systems of today, NM has evolved significantly over the decades. This evolution reflects the growing complexity of networks and the need for more advanced, integrated, and intelligent management solutions to ensure optimal network performance and security. As networks continue to evolve with emerging technologies like 5G and edge computing, the role of NM will remain critical in maintaining the reliability, efficiency, and security of modern communication infrastructures.
Applications and Uses of NM
The applications and uses of nanomaterials (NM) are vast and transformative, impacting various sectors in profound ways. At the forefront of these applications are industrial and manufacturing uses, where nanomaterials enhance the performance, durability, and efficiency of products. In the medical and health arena, nanomaterials are revolutionizing diagnostics, drug delivery, and tissue engineering, offering unprecedented precision and effectiveness. Additionally, environmental and sustainability roles of nanomaterials are becoming increasingly significant, as they help in pollution remediation, energy storage, and the development of sustainable technologies. These diverse applications underscore the versatility and potential of nanomaterials to drive innovation across multiple fields. As we delve into the specifics of these uses, it becomes clear that the industrial and manufacturing sector is one of the earliest adopters and most significant beneficiaries of nanotechnology, leveraging its advantages to create cutting-edge materials and processes that are reshaping industries worldwide. Therefore, let us first explore the industrial and manufacturing uses of nanomaterials in greater detail.
Industrial and Manufacturing Uses
Industrial and manufacturing uses of nanomaterials (NM) are revolutionizing various sectors by enhancing performance, efficiency, and sustainability. In the realm of materials science, nanomaterials offer unique properties such as increased strength, conductivity, and thermal resistance due to their nanoscale dimensions. For instance, carbon nanotubes and graphene are being integrated into composite materials to create lightweight yet incredibly strong components for aerospace and automotive industries. These advanced materials reduce weight while maintaining structural integrity, leading to improved fuel efficiency and reduced emissions. In the manufacturing sector, nanomaterials are transforming traditional processes through nanocoatings and surface modifications. Nanocoatings can provide self-cleaning surfaces, corrosion resistance, and enhanced wear resistance, which are crucial for extending the lifespan of industrial equipment and reducing maintenance costs. For example, titanium dioxide (TiO2) nanoparticles are used in self-cleaning paints that can degrade pollutants and maintain surface cleanliness under UV light exposure. The electronics industry is also a significant beneficiary of nanomaterials. Nanoparticles like silver and gold are used in conductive inks for printed electronics, enabling the production of flexible circuits and wearable devices. Additionally, quantum dots made from semiconductor nanomaterials are improving display technology by offering higher color accuracy and energy efficiency in LEDs and OLEDs. Furthermore, nanomaterials play a critical role in energy storage and generation. Lithium-ion batteries, for instance, utilize nanoscale materials to enhance charge storage capacity and cycle life. Graphene-based electrodes are being explored for their potential to significantly increase battery performance and reduce charging times. In solar energy, nanostructured surfaces can improve light absorption in photovoltaic cells, leading to higher conversion efficiencies. In the field of biotechnology and pharmaceuticals, nanomaterials are facilitating targeted drug delivery systems. Nanoparticles can be engineered to carry therapeutic agents directly to diseased cells while minimizing side effects on healthy tissues. This precision medicine approach is particularly promising for treating cancers and other complex diseases. Moreover, environmental applications of nanomaterials include water purification systems where nanoparticles can adsorb or break down pollutants more effectively than conventional methods. For example, iron oxide nanoparticles can catalytically degrade organic contaminants in wastewater streams. In summary, the integration of nanomaterials across various industrial and manufacturing sectors is driving innovation by leveraging their unique properties to enhance product performance, reduce costs, and promote sustainability. As research continues to advance our understanding of these materials, their applications are likely to expand further into new areas such as energy harvesting, biomedical devices, and smart textiles. The future of industrial manufacturing looks promising with the potential for nanomaterials to solve some of the most pressing challenges facing modern society today.
Medical and Health Applications
Medical and health applications of nanomaterials (NM) have revolutionized the healthcare sector by offering innovative solutions to diagnose, treat, and prevent diseases. One of the most significant advancements is in drug delivery systems, where nanoparticles can be engineered to target specific cells or tissues, enhancing the efficacy of treatments while minimizing side effects. For instance, liposomes and polymeric nanoparticles are used to deliver chemotherapy directly to cancer cells, reducing the impact on healthy tissues. Additionally, nanocarriers can be designed to release drugs in a controlled manner, ensuring sustained therapeutic effects over time. In diagnostics, nanotechnology has led to the development of highly sensitive and specific biosensors. These devices can detect biomarkers for various diseases at very low concentrations, enabling early diagnosis and intervention. Quantum dots, for example, are being used in fluorescence imaging to visualize cancer cells and monitor disease progression. Similarly, gold nanoparticles are employed in lateral flow assays for rapid detection of infectious diseases like HIV and tuberculosis. Nanomaterials also play a crucial role in tissue engineering and regenerative medicine. Nanofibers and scaffolds provide a supportive environment for cell growth and differentiation, facilitating the repair of damaged tissues. For instance, nanocomposite scaffolds are being explored for bone tissue engineering, promoting bone regeneration by mimicking the natural extracellular matrix. Furthermore, nanotechnology is transforming the field of wound healing. Nanoparticles and nanofibers can be incorporated into wound dressings to enhance wound closure rates and reduce the risk of infection. Silver nanoparticles, known for their antimicrobial properties, are commonly used in wound care products to prevent bacterial growth. In the realm of imaging, nanomaterials have improved the resolution and sensitivity of various imaging modalities. Superparamagnetic iron oxide nanoparticles (SPIONs) are used as contrast agents in magnetic resonance imaging (MRI), allowing for better visualization of tumors and other lesions. Similarly, gold nanoparticles are being explored as contrast agents in computed tomography (CT) scans due to their high X-ray absorption. Lastly, nanotechnology is paving the way for personalized medicine by enabling the development of personalized diagnostic tools and therapeutic strategies. For example, nanoparticles can be tailored to respond to specific genetic or environmental triggers, allowing for customized treatment plans based on individual patient profiles. Overall, the integration of nanomaterials into medical and health applications has the potential to significantly improve patient outcomes by enhancing diagnostic accuracy, treatment efficacy, and overall quality of life. As research continues to advance in this field, we can expect even more innovative solutions to emerge, further revolutionizing healthcare practices.
Environmental and Sustainability Roles
In the context of Applications and Uses of Nanomaterials (NM), environmental and sustainability roles are increasingly pivotal. Nanomaterials, due to their unique properties such as high surface area, reactivity, and strength, offer significant potential in addressing various environmental challenges. One of the key applications is in water treatment, where nanomaterials like graphene, carbon nanotubes, and metal oxides can be used to remove contaminants such as heavy metals, pesticides, and industrial pollutants from water. These materials can enhance filtration efficiency and reduce the energy required for purification processes, making clean water more accessible and sustainable. Another critical area is air pollution mitigation. Nanocatalysts can be employed to reduce emissions of harmful gases like NOx and SOx from industrial processes and vehicles. For instance, nanostructured catalysts can improve the efficiency of catalytic converters in vehicles, leading to cleaner air and reduced greenhouse gas emissions. Additionally, nanomaterials are being explored for their role in carbon capture and storage technologies, which are essential for mitigating climate change by reducing CO2 levels in the atmosphere. Sustainable energy solutions also benefit from nanomaterials. Solar cells and fuel cells can be enhanced with nanostructured materials to improve their efficiency and durability. For example, quantum dots and nanoparticles can increase the light absorption in solar cells, leading to higher energy conversion rates. Similarly, nanomaterials can enhance the performance of fuel cells by improving catalyst activity and reducing material costs. In agriculture, nanomaterials are being used to develop more sustainable practices. Nano-fertilizers and nano-pesticides can be designed to release nutrients and active ingredients in a controlled manner, reducing waste and minimizing environmental impact. This targeted delivery system can improve crop yields while reducing the amount of chemicals used, thereby promoting sustainable agriculture. Furthermore, nanomaterials play a crucial role in waste management. They can be used to create advanced composites for packaging materials that are biodegradable and recyclable, reducing plastic waste. Additionally, nanocatalysts can facilitate the breakdown of organic waste into valuable chemicals and fuels, contributing to a circular economy. In the realm of construction, nanomaterials are enhancing the sustainability of buildings. Nano-modified concrete, for instance, can improve the strength and durability of structures while reducing the amount of cement required, which is a significant source of greenhouse gas emissions. Similarly, nanocoatings can provide self-cleaning surfaces that reduce maintenance needs and improve energy efficiency by minimizing the need for frequent cleaning. Overall, the integration of nanomaterials into various sectors is driving significant advancements in environmental sustainability. By leveraging their unique properties, we can develop more efficient, cost-effective, and environmentally friendly solutions that address some of the most pressing global challenges. As research continues to evolve, the potential for nanomaterials to contribute to a more sustainable future looks promising.
Future Prospects and Challenges of NM
As we delve into the future prospects and challenges of Nanotechnology (NM), it is crucial to consider several key aspects that will shape its trajectory. First, advancements in technology and research are pivotal in driving innovation and overcoming current limitations. These advancements will be instrumental in enhancing the efficiency, safety, and applicability of nanotechnology across various sectors. Second, the economic and social implications of NM cannot be overlooked, as widespread adoption could significantly impact job markets, healthcare systems, and environmental policies. Lastly, regulatory frameworks and ethical considerations will play a critical role in ensuring that the benefits of NM are realized while mitigating potential risks and ensuring public trust. By examining these interrelated factors, we can better understand the complex landscape of NM's future. Let us begin by exploring the latest developments in technology and research, which are laying the groundwork for the next generation of nanotechnological innovations.
Advancements in Technology and Research
Advancements in technology and research have been pivotal in shaping the future prospects and challenges of nanomaterials (NM). Over the past few decades, significant strides have been made in understanding the properties and applications of NM, driven by breakthroughs in fields such as materials science, nanotechnology, and biomedicine. One of the key advancements is the development of sophisticated synthesis techniques, allowing for the precise control over the size, shape, and composition of nanoparticles. This has enabled researchers to tailor NM for specific applications, such as drug delivery systems, where nanoparticles can be engineered to target specific cells or tissues, enhancing therapeutic efficacy while minimizing side effects. The integration of nanotechnology with other disciplines has also led to innovative solutions. For instance, the convergence of nanomaterials with biotechnology has given rise to bio-nanomaterials that can mimic natural biological processes, leading to advancements in tissue engineering and regenerative medicine. Additionally, advancements in computational modeling and simulation have facilitated the prediction of NM behavior at the atomic and molecular levels, accelerating the discovery of new materials with unique properties. Furthermore, advancements in characterization techniques such as high-resolution transmission electron microscopy (HRTEM) and atomic force microscopy (AFM) have provided unprecedented insights into the structure and properties of NM. These tools allow researchers to study the interactions between NM and biological systems at the nanoscale, which is crucial for understanding potential toxicity and environmental impact. However, these advancements also pose challenges. The increasing complexity of NM synthesis and characterization requires significant investment in infrastructure and human resources. Moreover, the scalability of NM production remains a major challenge, as current methods often struggle to translate from laboratory settings to industrial scales without compromising quality or consistency. Another critical challenge is the regulatory framework surrounding NM. As these materials become more ubiquitous, there is a growing need for standardized guidelines to ensure their safe use and disposal. This includes addressing concerns related to environmental sustainability and human health, where long-term exposure effects are still not fully understood. Despite these challenges, the future prospects of NM are promising. Continued research is expected to unlock new applications in fields such as energy storage, where nanomaterials are being explored for their potential to enhance battery performance and efficiency. Additionally, NM are likely to play a crucial role in addressing global health challenges through the development of novel diagnostic tools and therapeutic agents. In conclusion, the rapid advancements in technology and research have significantly expanded our understanding and utilization of NM. While these developments present numerous opportunities for innovation, they also underscore the need for careful consideration of the associated challenges. Addressing these challenges will be essential to fully realizing the potential of NM and ensuring their safe and beneficial integration into various aspects of society.
Economic and Social Implications
The economic and social implications of Nanotechnology (NM) are multifaceted and far-reaching, influencing various sectors and aspects of society. On the economic front, NM has the potential to revolutionize industries such as healthcare, energy, and manufacturing by introducing innovative materials and processes that enhance efficiency and reduce costs. For instance, nanomaterials can improve the performance of solar cells, leading to more affordable renewable energy solutions. In healthcare, nanotechnology can enable targeted drug delivery systems, reducing side effects and improving treatment outcomes. These advancements could lead to significant economic savings through reduced healthcare costs and increased productivity. However, the integration of NM also poses challenges. The high initial investment required for research and development can be a barrier for many companies, particularly small and medium-sized enterprises. Additionally, there are concerns about job displacement as automation and advanced technologies replace traditional roles. To mitigate these effects, governments and industries must invest in retraining programs and education initiatives that prepare workers for the changing job market. Socially, NM has profound implications for public health and safety. The use of nanoparticles in consumer products raises questions about their potential toxicity and environmental impact. Regulatory bodies must establish stringent safety standards to ensure that these materials do not harm human health or the environment. Public awareness campaigns are also crucial to educate consumers about the benefits and risks associated with nanotechnology. Moreover, NM has the potential to exacerbate existing social inequalities if access to its benefits is not equitable. For example, advanced medical treatments enabled by nanotechnology might initially be available only to those who can afford them, widening the health gap between rich and poor populations. Therefore, policymakers must work towards ensuring that the benefits of NM are distributed fairly across different socio-economic groups. In conclusion, while NM offers tremendous economic opportunities and social improvements, it also presents significant challenges that need to be addressed. Balancing innovation with responsible regulation, equitable access, and workforce adaptation will be key to harnessing the full potential of nanotechnology for the betterment of society as a whole. As we look towards the future prospects and challenges of NM, it is essential to consider these economic and social implications carefully to ensure that this technology benefits everyone equally.
Regulatory Frameworks and Ethical Considerations
As we delve into the future prospects and challenges of Nanotechnology (NM), it is imperative to address the regulatory frameworks and ethical considerations that will shape its trajectory. The rapid advancement of NM has necessitated the development of robust regulatory frameworks to ensure safety, efficacy, and ethical use. Governments and international bodies are increasingly focusing on creating standards and guidelines that balance innovation with public health and environmental protection. For instance, the European Union's REACH (Registration, Evaluation, Authorization, and Restriction of Chemicals) regulation sets stringent requirements for the registration and use of nanomaterials, while the U.S. Environmental Protection Agency (EPA) has established specific guidelines under the Toxic Substances Control Act (TSCA) to regulate nanoscale substances. These frameworks aim to mitigate potential risks associated with NM, such as toxicity and environmental impact, thereby fostering public trust and confidence in these technologies. Ethical considerations are equally crucial, as NM raises complex questions about privacy, consent, and societal equity. The use of nanosensors and nanodevices in healthcare, for example, raises concerns about data privacy and informed consent. Ethical guidelines must be developed to ensure that these technologies are used transparently and with respect for individual rights. Moreover, there is a need for equitable access to NM benefits, particularly in developing countries where resources may be limited. Ensuring that NM does not exacerbate existing health disparities or widen the technological gap between nations is a significant ethical challenge. Additionally, the potential for NM to be used in military applications or for surveillance purposes highlights the need for stringent ethical oversight to prevent misuse. In the future, as NM continues to evolve, these regulatory and ethical frameworks will need to be dynamic and adaptable. Continuous research into the long-term effects of nanomaterials on human health and the environment will be essential for refining regulations. Public engagement and education are also vital components; an informed public can better participate in discussions about the ethical implications of NM and support policies that align with societal values. Ultimately, a harmonized approach to regulation and ethics will be crucial for realizing the full potential of NM while safeguarding public welfare and promoting sustainable development. By addressing these challenges proactively, we can ensure that NM contributes positively to human well-being and global progress.