What Is An Autotroph
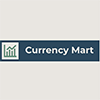
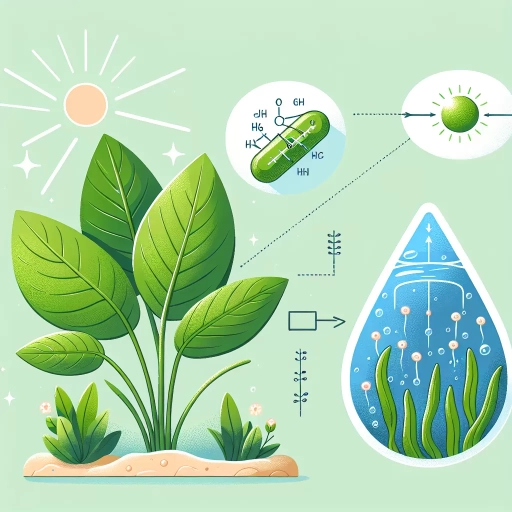
In the intricate web of life, autotrophs play a pivotal role as the primary producers of the biosphere. These organisms are capable of synthesizing their own food from inorganic substances, using energy from the sun or chemical reactions. This unique ability sets them apart from heterotrophs, which rely on consuming other organisms for sustenance. Understanding autotrophs is crucial for grasping the fundamental dynamics of ecosystems and the Earth's biosphere. This article delves into the world of autotrophs, beginning with a detailed **Definition and Types of Autotrophs**, where we explore the various forms these organisms take, including plants, algae, and certain bacteria. We will then examine the **Metabolic Processes in Autotrophs**, highlighting how these organisms convert inorganic materials into organic compounds through processes like photosynthesis and chemosynthesis. Finally, we will discuss the **Ecological Role and Importance of Autotrophs**, shedding light on their critical position in food chains and their impact on the environment. By understanding these aspects, we gain a deeper appreciation for the vital contributions autotrophs make to sustaining life on Earth. Let us start by defining what autotrophs are and the diverse types that exist.
Definition and Types of Autotrophs
Autotrophs, organisms that produce their own food using light, water, carbon dioxide, or chemicals, are the foundational pillars of life on Earth. These self-sustaining entities are crucial for the survival of nearly all living beings, as they form the base of food chains and ecosystems. Understanding autotrophs involves delving into their primary definition, which highlights their unique ability to synthesize organic compounds from inorganic materials. This understanding is further enriched by classifying them into two main types: photoautotrophs, which harness energy from sunlight, and chemoautotrophs, which derive energy from chemical reactions. By exploring these classifications and examining examples of different autotrophic organisms, we gain a comprehensive insight into the diverse ways these organisms thrive and contribute to the biosphere. In this article, we will delve into the **Definition and Types of Autotrophs**, exploring their primary definition, classification into photoautotrophs and chemoautotrophs, and providing examples of various autotrophic organisms.
Primary Definition of Autotrophs
**Primary Definition of Autotrophs** Autotrophs are organisms that have the unique ability to produce their own food through a process known as autotrophy. This fundamental characteristic distinguishes them from heterotrophs, which rely on consuming other organisms or organic matter for their nutritional needs. The term "autotroph" is derived from the Greek words "auto," meaning self, and "trophos," meaning nourishment. These self-sustaining organisms form the base of most food webs and ecosystems, playing a crucial role in the Earth's biosphere by converting inorganic substances into organic compounds. At the heart of autotrophy lies the process of photosynthesis, which is predominantly carried out by plants, algae, and certain types of bacteria. During photosynthesis, these organisms harness energy from sunlight to convert carbon dioxide and water into glucose and oxygen. This not only provides them with the necessary energy and organic compounds but also releases oxygen as a byproduct, which is essential for the survival of nearly all aerobic life forms. Besides photosynthesis, some autotrophs, such as chemolithoautotrophs, use chemical energy from inorganic compounds to synthesize their food. These microorganisms thrive in environments where sunlight is scarce, such as deep-sea vents and soil, and they play a vital role in nutrient cycling. The primary definition of autotrophs underscores their independence from external food sources, making them foundational to the structure and function of ecosystems. Without autotrophs, the complex web of life would collapse, as they are the primary producers that support the entire food chain. Herbivores feed on autotrophs, carnivores feed on herbivores, and decomposers break down dead autotrophs to recycle nutrients back into the environment. This interdependence highlights the critical role autotrophs play in maintaining ecological balance and ensuring the sustainability of life on Earth. In summary, autotrophs are self-nourishing organisms that produce their own food through processes like photosynthesis or chemosynthesis. Their ability to convert inorganic substances into organic matter makes them indispensable for the survival of all other life forms. Understanding the primary definition of autotrophs provides a foundational insight into the intricate dynamics of ecosystems and the vital role these organisms play in sustaining life on our planet.
Classification: Photoautotrophs and Chemoautotrophs
Autotrophs, organisms that produce their own food using light or chemical energy, are categorized into two primary types: photoautotrophs and chemoautotrophs. **Photoautotrophs** harness light energy to synthesize organic compounds from inorganic substances, a process known as photosynthesis. This group includes plants, algae, and certain bacteria like cyanobacteria. In photosynthesis, photoautotrophs use chlorophyll or other pigments to capture light energy, which is then converted into chemical energy stored in glucose. This glucose serves as a vital source of energy and building blocks for cellular components. The oxygen released during photosynthesis is a byproduct that supports aerobic respiration in other organisms, making photoautotrophs foundational to the Earth's food web. **Chemoautotrophs**, on the other hand, derive their energy from chemical reactions involving inorganic compounds. These organisms thrive in environments where light is scarce or absent, such as deep-sea vents and soil. Chemoautotrophs include certain bacteria and archaea that can oxidize ammonia, sulfur, or iron to produce ATP, the energy currency of cells. This process is known as chemosynthesis. For instance, nitrifying bacteria convert ammonia into nitrite and then nitrate, while sulfur-oxidizing bacteria convert sulfur into sulfate. These reactions not only provide energy for the chemoautotrophs but also play crucial roles in nutrient cycling within ecosystems. Chemoautotrophs are often found in symbiotic relationships with other organisms, such as giant tube worms, which lack digestive systems but thrive near hydrothermal vents where chemoautotrophic bacteria provide them with nutrients. Both photoautotrophs and chemoautotrophs are essential for maintaining the balance of ecosystems. They form the base of food chains by converting inorganic substances into organic matter that supports higher trophic levels. The diversity of autotrophic life forms ensures that energy and nutrients are cycled efficiently across various environments, from sunlit surfaces to deep-sea habitats. Understanding these autotrophic processes is vital for appreciating the intricate web of life and the complex interactions that sustain ecosystems on Earth. By recognizing the roles of photoautotrophs and chemoautotrophs, we gain insights into the fundamental mechanisms that underpin life on our planet.
Examples of Different Autotrophic Organisms
Autotrophic organisms, which produce their own food through various mechanisms, are fundamental to the Earth's ecosystem. These organisms can be broadly categorized into different types, each with unique characteristics and roles in their environments. **Photosynthetic Autotrophs** are perhaps the most well-known and include plants, algae, and cyanobacteria. These organisms use sunlight, carbon dioxide, and water to produce glucose and oxygen through the process of photosynthesis. For instance, plants such as trees and crops are primary producers in terrestrial ecosystems, while marine algae like phytoplankton form the base of aquatic food webs. Cyanobacteria, found in both water and soil, are ancient autotrophs that have played a crucial role in oxygenating the Earth's atmosphere. **Chemosynthetic Autotrophs**, on the other hand, derive energy from chemical reactions rather than sunlight. These organisms thrive in environments where light is scarce or absent, such as deep-sea vents and soil. Bacteria like Nitrosomonas and Thiobacillus are examples of chemosynthetic autotrophs that convert ammonia or sulfur compounds into energy-rich molecules. These bacteria are essential for nutrient cycling and support complex ecosystems in extreme environments. **Hydrothermal Vent Organisms** are another fascinating group of autotrophs. These microorganisms live near underwater volcanic vents where hot, chemical-rich fluids provide the necessary energy for chemosynthesis. Giant tube worms, for example, have symbiotic relationships with these bacteria, which live inside their tissues and provide them with nutrients. In addition to these well-studied groups, there are other less common types of autotrophs. **Radioautotrophs**, though still theoretical, would use radioactive decay as an energy source. While not yet discovered in nature, research into these potential organisms could reveal new insights into life's adaptability. Understanding the diversity of autotrophic organisms highlights their critical role in sustaining life on Earth. From the oxygen-producing plants that support aerobic respiration to the chemosynthetic bacteria that thrive in extreme conditions, autotrophs form the foundation of food webs and ecosystems across the globe. Their ability to convert inorganic substances into organic matter makes them indispensable for the survival of heterotrophic organisms, including humans. By exploring these different types of autotrophs, we gain a deeper appreciation for the intricate web of life and the remarkable adaptability of living organisms.
Metabolic Processes in Autotrophs
Autotrophs, organisms capable of producing their own food, play a pivotal role in the Earth's ecosystem by forming the base of the food chain. These self-sustaining entities thrive through various metabolic processes that enable them to convert inorganic substances into organic matter. This article delves into the intricate mechanisms behind these processes, focusing on three key areas: **Photosynthesis in Photoautotrophs**, where sunlight is harnessed to produce energy; **Chemolithoautotrophy in Chemoautotrophs**, where chemical energy is utilized; and **Energy Conversion Mechanisms**, which explore the biochemical pathways involved. By understanding these metabolic processes, we gain insight into how autotrophs sustain life on Earth. This comprehensive overview will transition seamlessly into a detailed exploration of **Definition and Types of Autotrophs**, providing a holistic understanding of these vital organisms and their diverse metabolic strategies.
Photosynthesis in Photoautotrophs
Photosynthesis in photoautotrophs is a complex yet fascinating metabolic process that underpins the very foundation of life on Earth. Photoautotrophs, such as plants, algae, and certain bacteria, have the unique ability to harness light energy from the sun to convert carbon dioxide and water into glucose and oxygen. This process occurs primarily in specialized organelles called chloroplasts, which contain pigments like chlorophyll that absorb light energy. The energy captured is then used to drive a series of chemical reactions known as the Calvin cycle, where CO₂ is fixed into organic compounds, and the light-dependent reactions, where water is split to produce ATP and NADPH. The light-dependent reactions take place in the thylakoid membranes of chloroplasts and involve the transfer of electrons from water to a special molecule called an electron acceptor. This electron transfer generates a proton gradient across the thylakoid membrane, which is used by ATP synthase to produce ATP. Simultaneously, NADPH is formed by reducing NADP+ with electrons. The Calvin cycle, also known as the light-independent reaction, utilizes the ATP and NADPH produced in the light-dependent reactions to convert CO₂ into glucose through a series of enzyme-catalyzed reactions. This intricate process not only provides energy and organic compounds for the autotrophs themselves but also supports nearly all other life forms by producing oxygen as a byproduct. Oxygen is released into the atmosphere, contributing significantly to the Earth's breathable air. Additionally, photosynthesis plays a crucial role in regulating Earth's climate by removing CO₂ from the atmosphere, thereby mitigating global warming. The efficiency and adaptability of photosynthesis are remarkable; it occurs in diverse environments, from aquatic ecosystems to arid deserts, and is essential for maintaining ecological balance. For instance, phytoplankton in oceans are responsible for a substantial portion of global photosynthesis, supporting marine food chains and influencing ocean chemistry. In summary, photosynthesis in photoautotrophs is an indispensable metabolic process that converts light energy into chemical energy, producing glucose and oxygen. This process is vital for sustaining life on Earth and underscores the critical role that autotrophs play in our ecosystem. Understanding photosynthesis not only deepens our appreciation for the intricate mechanisms of life but also highlights its importance in maintaining environmental health and supporting biodiversity.
Chemolithoautotrophy in Chemoautotrophs
Chemolithoautotrophy is a unique metabolic process exhibited by certain chemoautotrophs, which are a subset of autotrophs. These microorganisms thrive in environments where light is scarce or absent, such as deep-sea vents, soil, and freshwater sediments. Unlike photoautotrophs that rely on sunlight to drive their metabolic processes, chemoautotrophs harness chemical energy from inorganic compounds to synthesize organic molecules. This process is pivotal in ecosystems where photosynthesis cannot occur. Chemolithoautotrophs utilize various inorganic substrates like ammonia, nitrite, sulfur compounds, and hydrogen gas as energy sources. For instance, nitrifying bacteria convert ammonia into nitrite and then nitrate, releasing energy that is used to fix carbon dioxide into organic compounds via the Calvin-Benson-Bassham cycle or other carbon fixation pathways. Similarly, sulfur-oxidizing bacteria such as *Thiobacillus* species oxidize sulfur compounds to sulfate, generating ATP and reducing power necessary for biosynthesis. The metabolic pathways involved in chemolithoautotrophy are highly efficient and adaptable, allowing these organisms to thrive in diverse environments. For example, hydrogen-oxidizing bacteria like *Hydrogenobacter thermophilus* can live in hot springs where hydrogen gas is abundant, using it as an electron donor to produce ATP. This ability not only supports their own growth but also contributes significantly to the nutrient cycling within their ecosystems. Chemolithoautotrophy plays a crucial role in maintaining ecological balance. These organisms serve as primary producers in dark environments, providing organic matter that supports complex food webs. Additionally, they are key players in biogeochemical cycles, influencing the availability of essential nutrients such as nitrogen and sulfur for other organisms. In summary, chemolithoautotrophy in chemoautotrophs is an extraordinary metabolic strategy that enables these microorganisms to survive and flourish in environments devoid of light. By leveraging chemical energy from inorganic compounds, they produce organic matter and drive nutrient cycles, underscoring their importance in sustaining life on Earth. This unique capability highlights the remarkable diversity and resilience of autotrophic life forms and their indispensable role in maintaining the health and function of ecosystems.
Energy Conversion Mechanisms
Energy conversion mechanisms are pivotal in the metabolic processes of autotrophs, organisms that produce their own food using light, water, carbon dioxide, or chemicals. These mechanisms enable autotrophs to harness and transform energy from their environment into a usable form, primarily ATP (adenosine triphosphate), which is the energy currency of the cell. In photosynthetic autotrophs such as plants, algae, and certain bacteria, the primary energy conversion mechanism is photosynthesis. During photosynthesis, light energy from the sun is captured by chlorophyll and other pigments in the thylakoid membranes of chloroplasts. This light energy drives the conversion of carbon dioxide and water into glucose and oxygen through a series of complex biochemical reactions known as the light-dependent reactions and the Calvin cycle. The light-dependent reactions generate ATP and NADPH, which are then used in the Calvin cycle to synthesize glucose from CO₂. In chemosynthetic autotrophs, such as certain bacteria and archaea, energy is derived from chemical reactions involving inorganic compounds. These organisms use enzymes to catalyze reactions that release energy from substances like ammonia, sulfur compounds, or iron ions. This chemical energy is then converted into ATP through processes such as chemiosmosis, where protons are pumped across a membrane to create a proton gradient. The flow of protons back across this gradient drives the production of ATP via ATP synthase. Both photosynthetic and chemosynthetic pathways illustrate the fundamental principle of energy conversion in autotrophs: the transformation of environmental energy into biochemical energy that supports cellular functions and growth. These energy conversion mechanisms not only sustain the autotrophs themselves but also form the basis of life on Earth by providing the primary source of organic matter and oxygen for heterotrophic organisms. The efficiency and specificity of these mechanisms allow autotrophs to thrive in diverse environments, from the sunlit surfaces of oceans to deep-sea vents, highlighting their critical role in maintaining ecological balance and supporting the food chain. Understanding these energy conversion processes is essential for appreciating the metabolic versatility of autotrophs and their indispensable contribution to life on our planet.
Ecological Role and Importance of Autotrophs
Autotrophs, organisms capable of producing their own food through processes like photosynthesis or chemosynthesis, play a pivotal role in the ecological balance of our planet. These primary producers are the foundation of nearly all food chains, converting inorganic substances into organic matter that sustains life across various ecosystems. In this article, we will delve into the ecological significance of autotrophs, exploring their role in primary production, their crucial support of food chains and ecosystems, and their impact on environmental balance. By understanding these aspects, we can appreciate the multifaceted importance of autotrophs in maintaining the health and diversity of our natural world. This comprehensive overview will transition seamlessly into a detailed examination of the definition and types of autotrophs, providing a thorough understanding of these vital organisms.
Role in Primary Production
Autotrophs play a pivotal role in primary production, which is the foundation of nearly all ecosystems. These organisms, such as plants, algae, and certain bacteria, have the unique ability to convert inorganic substances into organic matter through processes like photosynthesis and chemosynthesis. This conversion of light energy or chemical energy into biological energy forms the basis of the food web, as autotrophs are the primary producers that support the entire trophic structure. In terrestrial ecosystems, plants are the dominant autotrophs responsible for primary production. They capture sunlight and use it to synthesize glucose from carbon dioxide and water, releasing oxygen as a byproduct. This process not only sustains plant growth but also supports herbivores that feed on plants, which in turn support carnivores that feed on herbivores. The energy and organic compounds produced by plants are essential for maintaining biodiversity and ecosystem health. In aquatic ecosystems, algae and cyanobacteria are key autotrophs. These microorganisms thrive in various water bodies, from freshwater lakes to marine environments, and contribute significantly to primary production. They form the base of aquatic food webs, providing sustenance for zooplankton, fish, and other aquatic organisms. The productivity of these autotrophs influences water quality, nutrient cycling, and the overall ecological balance of aquatic ecosystems. Beyond their role in food webs, autotrophs also influence their environments through various mechanisms. For instance, plants help stabilize soil through root systems, preventing erosion and maintaining soil quality. They also contribute to climate regulation by absorbing carbon dioxide and releasing oxygen, which helps mitigate the effects of global warming. In aquatic environments, algae can form blooms that affect water chemistry and support complex microbial communities. The importance of autotrophs in primary production extends to human societies as well. Agricultural crops, which are cultivated autotrophs, provide food for billions of people worldwide. Forests, dominated by autotrophic trees, offer timber, fuelwood, and numerous ecosystem services such as air and water purification. Additionally, autotrophs are crucial for biogeochemical cycles; they help recycle nutrients essential for their own growth and the growth of other organisms. In summary, autotrophs are indispensable for primary production, forming the energetic backbone of ecosystems. Their ability to convert inorganic resources into organic matter supports complex food webs and maintains ecological balance across diverse environments. Understanding the ecological role and importance of autotrophs is crucial for appreciating the intricate relationships within ecosystems and for managing natural resources sustainably.
Supporting Food Chains and Ecosystems
Supporting food chains and ecosystems is a critical ecological role played by autotrophs, which are organisms capable of producing their own food through processes such as photosynthesis or chemosynthesis. These primary producers form the foundational layer of nearly all food webs, converting inorganic substances into organic matter that sustains the entire food chain. Without autotrophs, the energy flow within ecosystems would be severely disrupted, leading to the collapse of complex food webs. Autotrophs, primarily plants, algae, and certain bacteria, capture sunlight or chemical energy to synthesize organic compounds like glucose. This process not only supports their own metabolic needs but also provides the necessary energy and nutrients for heterotrophs—organisms that cannot produce their own food. Herbivores feed on autotrophs, while carnivores and omnivores feed on herbivores or other carnivores, thereby transferring energy from one trophic level to the next. This hierarchical structure ensures that energy is efficiently distributed throughout the ecosystem. Moreover, autotrophs contribute significantly to maintaining ecosystem balance and biodiversity. They provide habitat and shelter for various species, support nutrient cycling by converting inorganic nutrients into forms that other organisms can use, and help regulate climate by absorbing carbon dioxide and releasing oxygen. For instance, forests composed of autotrophic trees are crucial for maintaining global oxygen levels and act as carbon sinks, mitigating the effects of climate change. In aquatic ecosystems, phytoplankton—a group of microscopic autotrophic algae—serve as the primary producers. They form the base of aquatic food webs, supporting zooplankton, fish, and other marine life. Similarly, in terrestrial ecosystems, grasslands and agricultural fields rely heavily on autotrophic plants to sustain grazing animals and human populations. The importance of autotrophs extends beyond their role in food chains; they also play a vital part in maintaining soil health through root systems that stabilize soil and facilitate nutrient uptake. Additionally, autotrophs influence local microclimates by regulating water cycles through transpiration and evaporation processes. In summary, autotrophs are indispensable for the functioning and resilience of ecosystems. By producing organic matter through photosynthesis or chemosynthesis, they support complex food webs, maintain ecosystem balance, and contribute to biodiversity. Their ecological importance underscores the need for conservation efforts aimed at protecting these primary producers and preserving the integrity of natural ecosystems.
Impact on Environmental Balance
The impact of autotrophs on environmental balance is profound and multifaceted, underscoring their ecological role as the foundational pillars of life on Earth. Autotrophs, primarily plants, algae, and certain bacteria, are organisms that produce their own food through photosynthesis or chemosynthesis. This ability to convert inorganic substances into organic matter not only sustains their own life processes but also supports the entire food web. By converting carbon dioxide into oxygen, autotrophs play a crucial role in maintaining atmospheric balance, ensuring that oxygen levels remain sufficient for aerobic respiration in other organisms. This oxygen production is essential for the survival of nearly all living beings, from simple microorganisms to complex multicellular organisms. Moreover, autotrophs are key players in the carbon cycle. They absorb carbon dioxide from the atmosphere and incorporate it into their biomass and soil through processes like root growth and decomposition. This sequestration of carbon helps mitigate climate change by reducing the concentration of greenhouse gases in the atmosphere. Additionally, autotrophs contribute to soil formation and fertility through root activity and leaf litter, which supports a diverse array of microbial and invertebrate life. These soil ecosystems are vital for nutrient cycling, water filtration, and erosion control, all of which are critical for maintaining healthy ecosystems. Autotrophs also provide habitat and shelter for countless species. Forests, grasslands, and aquatic ecosystems dominated by autotrophs serve as nurseries for juvenile fish, nesting sites for birds, and refuges for terrestrial animals. The structural complexity of these ecosystems supports biodiversity at multiple trophic levels, fostering intricate relationships between predators and prey that are essential for ecosystem resilience. In aquatic environments, autotrophic algae and phytoplankton form the base of aquatic food webs, supporting zooplankton, fish, and other marine life. These primary producers also influence water quality by absorbing nutrients such as nitrogen and phosphorus, thereby preventing eutrophication and maintaining water clarity. Furthermore, autotrophs have a significant impact on local climates through transpiration and evapotranspiration processes. By releasing water vapor into the atmosphere, they contribute to cloud formation and precipitation patterns, which in turn affect regional weather conditions and hydrological cycles. In summary, the ecological importance of autotrophs cannot be overstated. Their role in producing oxygen, sequestering carbon, forming soil, providing habitat, supporting aquatic life, and influencing climate underscores their critical position at the heart of environmental balance. Without autotrophs, life as we know it would be impossible; they are the unsung heroes that sustain ecosystems and ensure the health of our planet.