What Is H3o
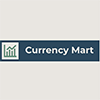
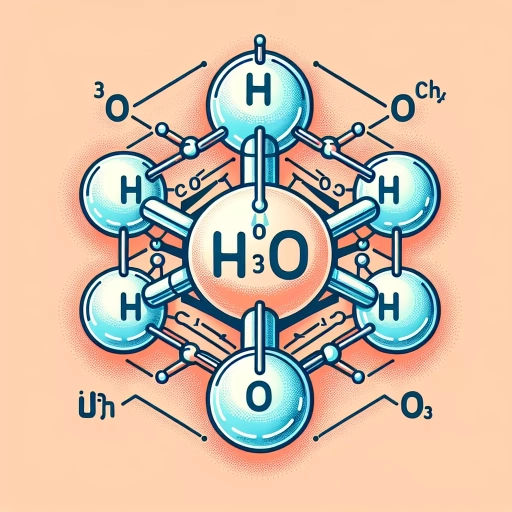
H3O, commonly known as the hydronium ion, is a fundamental concept in chemistry that plays a crucial role in various chemical reactions and biological processes. This ion is formed when a hydrogen ion (H+) combines with a water molecule (H2O), resulting in a positively charged species. Understanding the concept of H3O is essential for grasping its properties and behavior, which are pivotal in acidic environments and biochemical reactions. The properties and behavior of H3O, such as its structure, stability, and reactivity, are critical for comprehending its role in chemical systems. Furthermore, the applications and importance of H3O extend beyond the laboratory, influencing fields like environmental science, biochemistry, and industrial processes. In this article, we will delve into the concept of H3O, exploring its properties and behavior, as well as its significant applications and importance. Let's begin by understanding the concept of H3O.
Understanding the Concept of H3O
Understanding the concept of H3O, commonly known as the hydronium ion, is crucial for grasping various fundamental principles in chemistry. This ion plays a pivotal role in several chemical reactions and processes, making it a cornerstone in both theoretical and practical chemistry. To delve into the significance of H3O, it is essential to explore three key aspects: its definition and chemical structure, its historical context and discovery, and its scientific significance in chemistry. Firstly, understanding the definition and chemical structure of H3O is vital. This involves recognizing how a proton (H+) combines with a water molecule (H2O) to form the hydronium ion. Secondly, examining the historical context and discovery of H3O provides insight into how our understanding of this ion has evolved over time. Lastly, appreciating its scientific significance in chemistry highlights its importance in acid-base reactions, aqueous solutions, and biological systems. By exploring these facets, we can gain a comprehensive understanding of H3O. Let us begin by examining the definition and chemical structure of this critical ion, which forms the foundation of its role in chemistry.
Definition and Chemical Structure
**Definition and Chemical Structure** H₃O, commonly known as the hydronium ion, is a fundamental concept in chemistry that plays a crucial role in understanding various chemical reactions, particularly those involving acids and bases. **Definition:** The hydronium ion is a positively charged ion formed when a hydrogen ion (H⁺) combines with a water molecule (H₂O). This process occurs naturally in aqueous solutions where hydrogen ions are present, such as in acidic environments. The hydronium ion acts as a proton donor, making it an essential component in acid-base chemistry. **Chemical Structure:** The chemical structure of H₃O consists of one oxygen atom bonded to three hydrogen atoms. In this structure, the oxygen atom is bonded to two hydrogen atoms through covalent bonds, while the third hydrogen atom is bonded to the oxygen via a hydrogen bond. This arrangement results in a trigonal pyramidal shape due to the lone pair of electrons on the oxygen atom, which repels the bonded hydrogen atoms. The molecular formula for hydronium is H₃O⁺, indicating its positive charge. Understanding the hydronium ion's structure is vital because it influences its reactivity and stability. For instance, the positive charge on the hydronium ion makes it highly reactive and capable of participating in various chemical reactions, such as neutralization reactions with bases. Additionally, the presence of hydronium ions in a solution can significantly affect pH levels, making it a key indicator of acidity. In summary, the hydronium ion (H₃O⁺) is defined as the combination of a hydrogen ion with a water molecule, resulting in a positively charged ion with a specific trigonal pyramidal structure. This ion's unique chemical properties make it central to understanding acid-base chemistry and its applications across various scientific disciplines.
Historical Context and Discovery
The concept of H3O, or heavy water, is deeply rooted in historical context and scientific discovery. The journey to understanding H3O began in the early 20th century when scientists were exploring the properties of hydrogen isotopes. In 1931, American chemist Harold Urey discovered deuterium, a heavy isotope of hydrogen with a neutron in its nucleus, which is twice as heavy as the more common protium isotope. This breakthrough led to the synthesis of heavy water (D2O), where deuterium replaces the hydrogen atoms in water. Urey's discovery was pivotal because it opened up new avenues for research in nuclear physics and chemistry. The unique properties of heavy water, such as its higher boiling point and different chemical reactivity compared to regular water, made it an interesting subject for study. During World War II, heavy water gained significant attention due to its potential use in nuclear reactors and the production of atomic bombs. The Allies and Axis powers both sought to control supplies of heavy water, particularly from Norway's Vemork plant, which was one of the few facilities capable of producing it on an industrial scale. The historical context surrounding heavy water is also marked by espionage and sabotage. The Norwegian resistance, aided by British Special Operations Executive agents, conducted a series of daring operations known as the "Heavy Water Sabotage" to disrupt German production efforts. These actions significantly delayed the German nuclear program and are often cited as crucial in hindering their ability to develop an atomic bomb during the war. Post-war research continued to uncover the diverse applications of heavy water. In nuclear reactors, it serves as an excellent neutron moderator due to its ability to slow down neutrons without absorbing them, making it essential for certain types of reactors like the CANDU (CANada Deuterium Uranium) reactor used in Canada. Additionally, heavy water has been used in various scientific experiments, including those related to quantum mechanics and the study of biological systems. Understanding the historical context and discovery of H3O provides a rich backdrop for appreciating its significance in modern science and technology. From its initial discovery by Harold Urey to its critical role in wartime efforts and ongoing scientific research, heavy water remains an intriguing substance with unique properties that continue to inspire innovation and exploration. This historical narrative underscores the importance of fundamental scientific research and its potential impact on broader societal and technological advancements.
Scientific Significance in Chemistry
The scientific significance of chemistry lies in its ability to unravel the fundamental principles governing the behavior of matter, which is crucial for understanding complex phenomena such as the concept of H3O. Chemistry, as a discipline, delves into the atomic and molecular structures that underpin all physical and biological processes. By studying chemical reactions, properties, and interactions, chemists can elucidate how substances transform and interact at various scales. This understanding is pivotal for advancing fields like materials science, pharmacology, and environmental science. For instance, comprehending the chemistry behind water (H2O) and its variants, such as H3O (hydronium ion), is essential for grasping acid-base chemistry and its implications in biological systems and industrial processes. The hydronium ion, formed when a proton (H+) associates with a water molecule, plays a central role in acid-base reactions and pH regulation in aqueous solutions. This knowledge is vital for understanding biochemical pathways, drug design, and environmental monitoring. Furthermore, the study of chemical kinetics and thermodynamics provides insights into reaction mechanisms and energy transformations, which are critical for optimizing industrial processes and developing sustainable technologies. In summary, the scientific significance of chemistry stems from its capacity to explain and predict the behavior of matter at all levels, thereby enabling advancements across diverse scientific and technological domains, including the nuanced understanding of H3O and its role in chemical and biological systems.
Properties and Behavior of H3O
The properties and behavior of H3O, commonly known as the hydronium ion, are multifaceted and crucial for understanding various chemical and biological processes. This article delves into three key aspects: Physical Properties and Characteristics, Chemical Reactions and Interactions, and Stability and Existence Conditions. By examining the physical properties of H3O, we gain insight into its structure, charge distribution, and how it interacts with other molecules. This understanding is foundational for grasping its chemical reactions and interactions, which are pivotal in acid-base chemistry and biological systems. Furthermore, exploring the stability and existence conditions of H3O reveals the environments in which it can form and persist, shedding light on its role in aqueous solutions. To begin, let's focus on the physical properties and characteristics of H3O, which set the stage for a comprehensive analysis of its behavior and significance.
Physical Properties and Characteristics
Physical properties and characteristics are fundamental aspects that define the behavior and interactions of any substance, including H3O, which is commonly known as hydronium ion. In the context of H3O, these properties are crucial for understanding its role in various chemical and biological processes. **Molecular Structure**: The hydronium ion consists of one oxygen atom bonded to three hydrogen atoms, forming a trigonal pyramidal shape. This structure is stabilized by the delocalization of electrons, making it a relatively stable ion. **Charge and Polarity**: H3O+ carries a positive charge due to the protonation of water (H2O). This positive charge influences its interactions with other molecules, particularly in aqueous solutions where it acts as a strong acid. **Solubility**: Hydronium ions are highly soluble in water, which is why they are often found in aqueous environments. Their solubility is a result of their ability to form hydrogen bonds with water molecules. **Conductivity**: Solutions containing H3O+ ions are good conductors of electricity because the ions can move freely, carrying charge through the solution. This property is significant in electrochemical reactions and biological systems. **Reactivity**: As a strong acid, H3O+ is highly reactive. It readily donates its proton (H+), making it a key player in acid-base chemistry and enzymatic reactions within living organisms. **Thermal Stability**: The thermal stability of H3O+ is moderate; it can exist stably at room temperature but decomposes into water and a proton at higher temperatures or in the presence of strong bases. **Biological Significance**: In biological systems, H3O+ plays a critical role in maintaining pH balance and facilitating enzymatic reactions. It is involved in the functioning of various cellular processes, including protein synthesis and metabolic pathways. Understanding these physical properties and characteristics of H3O+ is essential for grasping its behavior and importance in both chemical and biological contexts. These attributes underpin its role as a fundamental component in many natural and synthetic systems.
Chemical Reactions and Interactions
Chemical reactions and interactions are fundamental processes that underpin the behavior and properties of H₃O⁺, commonly known as the hydronium ion. In aqueous solutions, H₃O⁺ is formed when a hydrogen ion (H⁺) associates with a water molecule (H₂O). This association is a prime example of a chemical interaction where the proton (H⁺) acts as a Lewis acid, accepting a pair of electrons from the oxygen atom in water, thus forming a stable complex. This interaction is crucial because it influences the pH of the solution, with higher concentrations of H₃O⁺ indicating more acidic conditions. The formation of H₃O⁺ involves several key chemical reactions. For instance, when an acid like hydrochloric acid (HCl) dissolves in water, it dissociates into H⁺ and Cl⁻ ions. The H⁺ ions then react with water molecules to form H₃O⁺ and hydroxide ions (OH⁻) in a process known as autoionization. This equilibrium reaction is represented by the equation: 2H₂O ⇌ H₃O⁺ + OH⁻. The concentration of H₃O⁺ in solution is directly related to the strength of the acid; stronger acids produce more H₃O⁺ ions, leading to higher acidity. Chemical interactions involving H₃O⁺ also play a significant role in various biochemical processes. For example, in enzymatic reactions, H₃O⁺ can act as a catalyst or reactant, facilitating the breakdown or synthesis of molecules. In biological systems, maintaining the balance of H₃O⁺ and OH⁻ ions is critical for cellular functions and overall health. The body's buffering systems, such as the bicarbonate buffering system, help regulate pH levels by controlling the concentration of H₃O⁺. Furthermore, the properties of H₃O⁺ are influenced by its chemical structure and interactions. The hydronium ion has a trigonal pyramidal shape due to the sp³ hybridization of the oxygen atom, which allows it to form hydrogen bonds with other water molecules. These hydrogen bonds are essential for the solvation of ions and the structure of aqueous solutions. Additionally, the high mobility of H₃O⁺ in water contributes to its role in electrical conductivity and transport processes within biological and chemical systems. In summary, the chemical reactions and interactions involving H₃O⁺ are pivotal in understanding its properties and behavior. These interactions govern the acidity of solutions, influence biochemical processes, and are integral to maintaining physiological balance. The unique structure and reactivity of H₃O⁺ make it a central component in various chemical and biological contexts, underscoring its importance in both natural and synthetic environments.
Stability and Existence Conditions
Stability and existence conditions are crucial factors in understanding the properties and behavior of H₃O⁺, commonly known as the hydronium ion. This ion is formed when a water molecule (H₂O) accepts a proton (H⁺), a process that occurs naturally in aqueous solutions. The stability of H₃O⁺ is influenced by several key factors: 1. **Thermodynamic Stability**: The formation of H₃O⁺ is thermodynamically favorable due to the high electronegativity of oxygen, which allows it to form strong hydrogen bonds with surrounding water molecules. This network of hydrogen bonds enhances the ion's stability in aqueous environments. 2. **pH Conditions**: The existence of H₃O⁺ is directly related to the pH of the solution. At acidic pH levels (pH < 7), the concentration of protons (H⁺) is higher, leading to an increased formation of hydronium ions. Conversely, at basic pH levels (pH > 7), the concentration of hydroxide ions (OH⁻) is higher, which tends to neutralize H₃O⁺. 3. **Solvent Effects**: The solvent in which H₃O⁺ exists significantly affects its stability. Water is an ideal solvent because it can form multiple hydrogen bonds with the hydronium ion, stabilizing it through solvation. In non-aqueous solvents, the stability of H₃O⁺ can be compromised due to weaker interactions. 4. **Temperature**: Temperature also plays a role in the stability and existence of H₃O⁺. At higher temperatures, the kinetic energy of the molecules increases, potentially disrupting the hydrogen bond network that stabilizes the hydronium ion. However, within typical biological and environmental conditions, this effect is minimal. 5. **Chemical Environment**: The chemical environment surrounding H₃O⁺ can influence its stability. For instance, in the presence of strong bases or certain organic compounds, the hydronium ion may be neutralized or complexed, affecting its existence. Understanding these stability and existence conditions is essential for comprehending the behavior of H₃O⁺ in various chemical and biological contexts. For example, in biological systems, the regulation of pH and the presence of buffering agents can significantly impact the concentration and activity of hydronium ions, which in turn affect enzymatic reactions and cellular processes. Similarly, in industrial applications such as acid-base chemistry and electrochemistry, knowing how to manipulate the conditions for H₃O⁺ formation and stability is critical for optimizing processes and outcomes. Thus, the interplay between these factors underscores the importance of considering stability and existence conditions when studying the properties and behavior of H₃O⁺.
Applications and Importance of H3O
Water (H2O) is a fundamental component of life and technology, playing a crucial role in various biological, industrial, and environmental contexts. In biological systems, water is essential for maintaining cellular functions, regulating body temperature, and facilitating metabolic processes. Its importance extends to industrial and technological uses, where it serves as a solvent, coolant, and reactant in numerous manufacturing processes. Additionally, water's environmental impact is significant, influencing ecosystems, climate regulation, and human health. Understanding these multifaceted roles is vital for appreciating the full scope of water's applications and importance. This article will delve into these aspects, beginning with the critical role of water in biological systems.
Role in Biological Systems
In biological systems, water (H₂O) plays a pivotal role that is both multifaceted and indispensable. It serves as the medium in which many of the chemical reactions of life occur, facilitating metabolic processes and maintaining cellular homeostasis. Water's high specific heat capacity helps regulate body temperature, ensuring that organisms can function optimally across a range of environmental conditions. Its solvent properties allow it to dissolve a wide variety of substances, making it an essential component for transporting nutrients, oxygen, and waste products throughout the body via the circulatory system. Additionally, water is crucial for maintaining cellular structure; it fills the spaces between cells and helps maintain tissue shape and integrity. In plants, water is vital for photosynthesis and transpiration, processes that are fundamental to their survival and growth. Furthermore, water's role in digestion and absorption of nutrients cannot be overstated; it helps break down food in the digestive tract and aids in the absorption of nutrients into the bloodstream. The lubricating properties of water also protect joints and other moving parts of the body, reducing friction and wear. In summary, water's unique chemical and physical properties make it an irreplaceable component of all living organisms, underpinning virtually every biological process and ensuring the proper functioning of cells, tissues, and entire organisms.
Industrial and Technological Uses
Industrial and technological uses of H3O, or heavy water, are diverse and critical in various sectors. Heavy water, which contains deuterium instead of hydrogen, has unique properties that make it invaluable in nuclear applications. One of the most significant uses is in nuclear reactors, where it serves as a neutron moderator and coolant. This is particularly important in pressurized heavy water reactors (PHWRs) and other types of reactors that rely on natural uranium or other non-enriched fuels. The ability of heavy water to slow down neutrons without absorbing them allows for more efficient fission reactions, making it a crucial component in the generation of nuclear power. In addition to its role in nuclear energy, heavy water is used in scientific research. It is a key component in the production of deuterated compounds, which are essential in various fields such as chemistry, biology, and materials science. Deuterated compounds are used as tracers in chemical reactions and biological processes, allowing scientists to study complex mechanisms with greater precision. Heavy water itself is also used as a solvent in NMR (Nuclear Magnetic Resonance) spectroscopy, enabling detailed structural analysis of molecules. Heavy water's isotopic properties make it useful in the field of medicine as well. It is used in the production of certain medical isotopes, such as deuterium-labeled compounds, which are employed in diagnostic imaging and therapeutic treatments. For instance, deuterated water can be used to study metabolic processes in the body by tracing the movement of deuterium through biological systems. Furthermore, heavy water has applications in the field of materials science. It is used to study the properties of materials under different conditions. For example, deuterium can be substituted for hydrogen in materials to study their mechanical and thermal properties without altering their chemical structure significantly. In industrial processes, heavy water is used as a heat transfer medium due to its high specific heat capacity and thermal conductivity. This makes it suitable for cooling systems in various industrial applications where efficient heat management is critical. Overall, the unique properties of H3O make it an indispensable resource across multiple industrial and technological domains, from energy production and scientific research to medical applications and materials science. Its versatility and critical role underscore its importance in advancing various fields and ensuring the efficiency and safety of numerous industrial processes.
Environmental Impact and Considerations
The environmental impact and considerations of H3O, or heavy water, are multifaceted and significant. Heavy water, which contains deuterium instead of hydrogen, is primarily used in nuclear reactors as a coolant and neutron moderator. One of the key environmental concerns is the potential for radioactive contamination. If not handled properly, heavy water can become contaminated with radioactive isotopes, posing risks to both human health and the environment. This necessitates stringent safety protocols and rigorous waste management practices to prevent accidental releases. Another consideration is the energy consumption and greenhouse gas emissions associated with the production of heavy water. The process of isolating deuterium from regular water is energy-intensive, contributing to carbon emissions. Additionally, the transportation and storage of heavy water require specialized facilities, which can have further environmental impacts due to the need for secure and controlled environments. However, heavy water also has some positive environmental attributes. For instance, it can be used in advanced nuclear reactors that are designed to be safer and more efficient than traditional reactors. These reactors can potentially reduce the overall nuclear waste generated and minimize the risk of nuclear accidents. Furthermore, research into heavy water applications is driving innovation in nuclear technology, which could lead to cleaner and more sustainable energy solutions in the future. In terms of broader ecological impact, the extraction of heavy water does not directly harm ecosystems since it is derived from natural water sources. However, the indirect effects of increased energy consumption and potential radioactive contamination must be carefully managed to ensure minimal harm to aquatic life and terrestrial ecosystems. Overall, while the use of heavy water presents several environmental challenges, it also offers opportunities for advancing nuclear technology in a way that could contribute to a more sustainable energy mix. Balancing these considerations through responsible production, handling, and disposal practices is crucial for mitigating adverse environmental impacts while harnessing the benefits of heavy water in various applications.