What Is Dehydration Synthesis
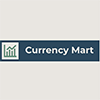
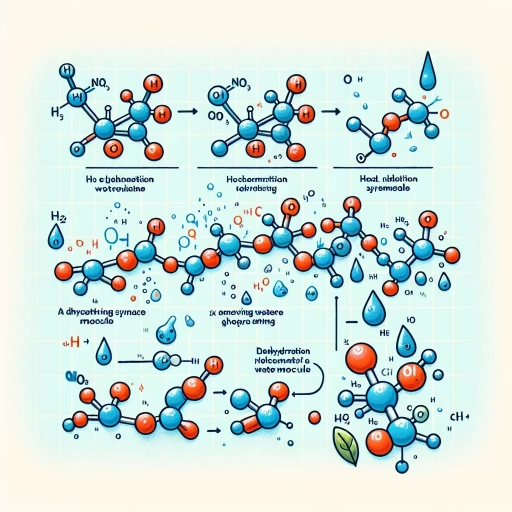
Dehydration synthesis is a fundamental chemical reaction that plays a crucial role in the formation of various biological molecules, such as proteins, carbohydrates, and nucleic acids. This process involves the removal of a water molecule from two reactants, resulting in the formation of a new covalent bond. Understanding dehydration synthesis is essential for grasping how these vital molecules are constructed and how they function within living organisms. In this article, we will delve into the intricacies of dehydration synthesis, starting with a detailed explanation of the process itself. We will then explore examples and applications of dehydration synthesis, highlighting its significance in biological systems and industrial processes. Finally, we will examine the mechanisms and factors that influence dehydration synthesis, providing insights into the conditions and catalysts that facilitate this reaction. By understanding these aspects, we can better appreciate the importance of dehydration synthesis in both natural and synthetic contexts. Let us begin by understanding dehydration synthesis in depth.
Understanding Dehydration Synthesis
Dehydration synthesis is a fundamental biochemical process that underpins the formation of various biomolecules essential for life. This article delves into the intricacies of dehydration synthesis, providing a comprehensive understanding through three key aspects: **Definition and Basic Concept**, **Chemical Reaction Process**, and **Importance in Biological Systems**. By defining what dehydration synthesis is and exploring its basic principles, we establish a solid foundation for understanding how this reaction occurs. The chemical reaction process section will detail the step-by-step mechanisms involved, highlighting the roles of reactants, products, and catalysts. Finally, the importance of dehydration synthesis in biological systems will be elucidated, revealing its critical role in the synthesis of proteins, polysaccharides, and other vital molecules. To begin, let's start with the **Definition and Basic Concept** of dehydration synthesis, which sets the stage for a deeper exploration of this vital biochemical reaction.
Definition and Basic Concept
Dehydration synthesis, a fundamental biochemical process, is defined as the chemical reaction where two molecules combine to form a new compound, releasing a water molecule (H₂O) in the process. This concept is crucial in understanding various biological processes, particularly in the formation of macromolecules such as proteins, polysaccharides, and nucleic acids. At its core, dehydration synthesis involves the condensation of two molecules, typically involving the loss of a hydroxyl group (-OH) from one molecule and a hydrogen atom (H-) from another. This reaction is often catalyzed by enzymes and is essential for building complex biomolecules from simpler precursors. For instance, in protein synthesis, amino acids are linked together through peptide bonds via dehydration synthesis. Here, the carboxyl group of one amino acid reacts with the amino group of another, resulting in the formation of a peptide bond and the release of water. Similarly, in the synthesis of polysaccharides like glycogen or starch, glucose molecules are joined together through glycosidic bonds, also involving dehydration synthesis. This process allows cells to construct large, complex molecules necessary for various cellular functions, such as structural support, energy storage, and genetic information storage. Understanding dehydration synthesis is vital because it highlights how cells efficiently use resources to build essential biomolecules while minimizing waste. The release of water as a byproduct is a key aspect, as it ensures that the reaction is thermodynamically favorable and can proceed under physiological conditions. Additionally, dehydration synthesis is reversible; the process can be undone through hydrolysis, where water is added back to break the bond, illustrating the dynamic nature of cellular metabolism. In summary, dehydration synthesis is a fundamental biochemical reaction that underpins the formation of many critical biomolecules. By grasping this basic concept, one can better appreciate the intricate mechanisms by which cells construct and maintain their complex structures and functions. This understanding is essential for fields such as biochemistry, molecular biology, and medicine, where knowledge of these processes can lead to insights into disease mechanisms and therapeutic strategies.
Chemical Reaction Process
Dehydration synthesis is a fundamental chemical reaction process that involves the removal of a water molecule from two reactants to form a new compound. This process is crucial in various biological and industrial contexts, particularly in the formation of macromolecules such as proteins, polysaccharides, and nucleic acids. During dehydration synthesis, two molecules with hydroxyl (-OH) groups react to eliminate a water molecule (H₂O), resulting in the formation of a covalent bond between the reactants. For instance, in the synthesis of proteins, amino acids combine through dehydration reactions to form peptide bonds, linking the amino acids into a polypeptide chain. Similarly, in carbohydrate synthesis, monosaccharides undergo dehydration reactions to form glycosidic bonds, creating polysaccharides like starch and cellulose. This reaction is typically catalyzed by enzymes that facilitate the removal of the water molecule and stabilize the transition state. The energy required for these reactions often comes from ATP (adenosine triphosphate), which is hydrolyzed to release energy that drives the synthesis forward. Understanding dehydration synthesis is essential for grasping how cells construct complex molecules from simpler precursors, highlighting its pivotal role in cellular metabolism and biosynthesis. By recognizing the mechanisms and significance of dehydration synthesis, scientists can better appreciate the intricate biochemical processes that underpin life at the molecular level.
Importance in Biological Systems
Dehydration synthesis is a fundamental process in biological systems, playing a crucial role in the formation of various biomolecules essential for life. This type of synthesis involves the removal of a water molecule from two reactants, resulting in the formation of a new covalent bond. The importance of dehydration synthesis can be seen across multiple biological contexts. 1. **Protein Synthesis**: During protein synthesis, dehydration reactions occur between amino acids to form peptide bonds, which are the backbone of proteins. These proteins are vital for structural support, catalyzing biochemical reactions, and regulating cellular processes. 2. **Carbohydrate Formation**: In the biosynthesis of polysaccharides like glycogen and starch, dehydration reactions link glucose molecules together, forming glycosidic bonds. These polysaccharides serve as energy storage molecules in cells. 3. **Lipid Synthesis**: The formation of triglycerides from glycerol and fatty acids involves dehydration reactions, creating ester bonds that are essential for energy storage and cell membrane structure. 4. **DNA and RNA Synthesis**: Phosphodiester bonds, which link nucleotides together in DNA and RNA, are formed through dehydration reactions. These bonds are critical for the integrity and function of genetic material. 5. **Cell Wall Formation**: In plants and some bacteria, dehydration synthesis is involved in the formation of cellulose and peptidoglycan, respectively, which are key components of cell walls providing structural support and protection. 6. **Energy Storage and Metabolism**: Dehydration synthesis is integral to the formation of ATP (adenosine triphosphate), the primary energy currency of cells. The phosphate groups in ATP are linked via phosphoanhydride bonds formed through dehydration reactions. 7. **Cellular Signaling**: Some signaling molecules, such as phospholipids involved in cell signaling pathways, are synthesized through dehydration reactions. These molecules play critical roles in regulating various cellular activities. In summary, dehydration synthesis is a ubiquitous process in biological systems that underpins the creation of diverse biomolecules necessary for cellular structure, function, and metabolism. Its importance extends across various biochemical pathways, highlighting its central role in sustaining life at the molecular level. Understanding dehydration synthesis is essential for grasping how cells construct and maintain their complex structures and functions.
Examples and Applications of Dehydration Synthesis
Dehydration synthesis is a fundamental chemical reaction that plays a crucial role in various biological and industrial processes. This reaction involves the removal of a water molecule from two reactants, resulting in the formation of a new bond. The significance of dehydration synthesis can be seen in several key areas. Firstly, it is essential for the **Formation of Polymers**, where it enables the creation of long-chain molecules such as proteins, carbohydrates, and nucleic acids. Secondly, dehydration synthesis is integral to **Role in Metabolic Pathways**, facilitating key biochemical reactions that sustain life. Lastly, its **Industrial and Medical Uses** are diverse, ranging from the production of synthetic fibers to the development of pharmaceuticals. Understanding these applications highlights the versatility and importance of dehydration synthesis in both natural and synthetic contexts. By examining these aspects, we can appreciate the critical role this reaction plays in forming the building blocks of life and driving technological advancements. Let us begin by exploring how dehydration synthesis contributes to the **Formation of Polymers**.
Formation of Polymers
The formation of polymers is a fundamental process in chemistry, particularly through dehydration synthesis. Dehydration synthesis, also known as condensation reaction, involves the removal of a water molecule from two monomers to form a larger molecule, or polymer. This process is crucial in the creation of various types of polymers, including proteins, carbohydrates, and synthetic materials like nylon and polyester. In the context of proteins, dehydration synthesis occurs during peptide bond formation. Here, amino acids react with each other, releasing a water molecule as they link together to form a polypeptide chain. This chain can then fold into its native conformation to create a functional protein. For instance, the synthesis of collagen—a protein essential for connective tissue—occurs through this mechanism, where amino acids like glycine and proline are linked together through peptide bonds. Carbohydrates also undergo dehydration synthesis to form polysaccharides. For example, starch and cellulose are formed when glucose molecules are linked together through glycosidic bonds, releasing water in the process. These polysaccharides serve vital roles in energy storage and structural support in plants and animals. Synthetic polymers are another significant application of dehydration synthesis. Nylon, for instance, is produced through the condensation reaction between adipic acid and hexamethylene diamine. This reaction results in the formation of amide bonds and the release of water molecules, leading to the creation of nylon fibers used in textiles and industrial applications. Similarly, polyester is synthesized from ethylene glycol and terephthalic acid through a condensation reaction that produces ester linkages and releases water. The versatility of dehydration synthesis in polymer formation extends to various industrial and biological contexts. In biotechnology, this process is harnessed to produce biodegradable polymers such as polylactic acid (PLA) from lactic acid monomers. PLA is used in medical implants, packaging materials, and 3D printing due to its biocompatibility and environmental sustainability. In summary, dehydration synthesis is a pivotal mechanism for the formation of polymers across diverse fields. From the biological synthesis of proteins and carbohydrates to the industrial production of synthetic materials like nylon and polyester, this process underpins many essential applications in modern science and technology. Understanding dehydration synthesis is crucial for appreciating how complex molecules are built from simpler monomers, highlighting its significance in both natural and synthetic contexts.
Role in Metabolic Pathways
Dehydration synthesis plays a pivotal role in various metabolic pathways, which are essential for the proper functioning of living organisms. One of the most significant roles is in the synthesis of macromolecules such as proteins, polysaccharides, and nucleic acids. For instance, in protein synthesis, dehydration reactions occur during peptide bond formation between amino acids. This process involves the removal of a water molecule as two amino acids join together, forming a polypeptide chain. Similarly, in the synthesis of polysaccharides like glycogen and starch, dehydration reactions link glucose molecules together, releasing water in the process. These reactions are crucial for energy storage and structural integrity in cells. In nucleic acid synthesis, dehydration reactions are fundamental in the formation of phosphodiester bonds between nucleotides, leading to the creation of DNA and RNA. This process is vital for genetic information storage and transmission. Additionally, dehydration synthesis is involved in lipid metabolism, particularly in the formation of triglycerides from glycerol and fatty acids, which are essential for energy storage and cell membrane structure. Furthermore, dehydration synthesis is integral to the citric acid cycle (Krebs cycle), where it occurs during the conversion of citrate to isocitrate. This step involves the dehydration of citrate to form cis-aconitate, which is then rehydrated to isocitrate. This cycle is a key component of cellular respiration, contributing to the production of ATP, NADH, and FADH2. In glycolysis, another critical metabolic pathway, dehydration synthesis occurs during the conversion of fructose-1,6-bisphosphate to glyceraldehyde-3-phosphate and dihydroxyacetone phosphate. This step is catalyzed by the enzyme aldolase and involves the removal of a water molecule. Moreover, dehydration synthesis is involved in the pentose phosphate pathway, where it helps in generating NADPH and pentoses from glucose-6-phosphate. This pathway is essential for biosynthetic reactions and protecting cells from oxidative damage. In summary, dehydration synthesis is a ubiquitous reaction mechanism that underpins many fundamental metabolic processes. Its role in forming peptide bonds, phosphodiester bonds, and glycosidic bonds is indispensable for the synthesis of proteins, nucleic acids, and polysaccharides. Additionally, its involvement in lipid metabolism and key energy-producing pathways like the citric acid cycle and glycolysis underscores its critical importance in maintaining cellular homeostasis and overall organismal health.
Industrial and Medical Uses
Dehydration synthesis, a fundamental chemical reaction where two molecules combine to form a new compound with the loss of a water molecule, has extensive applications across various industries, particularly in industrial and medical fields. In the industrial sector, dehydration synthesis is crucial for the production of polymers such as nylon and polyethylene terephthalate (PET). For instance, nylon is synthesized through the condensation reaction between adipic acid and hexamethylene diamine, resulting in a strong and versatile polymer used in textiles, automotive parts, and industrial components. Similarly, PET is produced through the dehydration synthesis of ethylene glycol and terephthalic acid, yielding a material widely used in packaging, fibers, and films. In the medical field, dehydration synthesis plays a pivotal role in the synthesis of drugs and biopolymers. For example, certain antibiotics like penicillin are synthesized using dehydration reactions to form amide bonds between amino acids. Additionally, biodegradable polymers such as polylactic acid (PLA) and polyglycolic acid (PGA) are produced via dehydration synthesis for use in medical implants, sutures, and drug delivery systems. These biopolymers are particularly valuable because they can degrade naturally in the body without causing adverse reactions. Furthermore, dehydration synthesis is essential in the production of pharmaceuticals. Many drugs are synthesized through condensation reactions that involve the formation of peptide bonds between amino acids or other organic molecules. For instance, the synthesis of insulin involves multiple dehydration steps to form the peptide bonds necessary for its structure and function. This process ensures that the final product has the correct molecular configuration to effectively regulate blood sugar levels. Moreover, in tissue engineering and regenerative medicine, dehydration synthesis is used to create scaffolds from biopolymers that support cell growth and tissue regeneration. These scaffolds are designed to degrade at a rate that allows new tissue to form, making them invaluable for repairing damaged tissues and organs. In summary, dehydration synthesis is a cornerstone of both industrial and medical applications due to its ability to form complex molecules with specific properties. Its role in polymer production, drug synthesis, and biopolymer creation underscores its importance in advancing technology and healthcare.
Mechanisms and Factors Influencing Dehydration Synthesis
Dehydration synthesis, a fundamental process in biochemistry, involves the formation of a new chemical bond between two molecules with the simultaneous loss of a water molecule. This intricate mechanism is influenced by several key factors that ensure its efficiency and specificity. At the heart of dehydration synthesis are catalysts and enzymes, which play a crucial role in facilitating these reactions by lowering the activation energy required. Additionally, environmental conditions such as temperature, pH, and solvent composition significantly impact the rate and yield of these reactions. Lastly, understanding the energy requirements and efficiency of dehydration synthesis is essential for optimizing these processes in both biological systems and industrial applications. By examining these three critical aspects—catalysts and enzymes involved, environmental conditions, and energy requirements and efficiency—we can gain a comprehensive understanding of how dehydration synthesis operates. This article will delve into these factors, starting with the pivotal role of catalysts and enzymes involved in dehydration synthesis.
Catalysts and Enzymes Involved
In the context of dehydration synthesis, catalysts and enzymes play crucial roles in facilitating and regulating the reaction mechanisms. Dehydration synthesis, a fundamental process in biochemistry, involves the formation of a new chemical bond between two molecules with the simultaneous loss of a water molecule. Catalysts, in general, are substances that speed up chemical reactions without being consumed in the process. Enzymes, which are biological catalysts, are particularly important in dehydration synthesis due to their high specificity and efficiency. Enzymes such as DNA polymerase and RNA polymerase are essential in nucleic acid synthesis, where they catalyze the formation of phosphodiester bonds between nucleotides. These enzymes ensure that the correct nucleotides are incorporated into the growing DNA or RNA chain, thereby maintaining genetic fidelity. Similarly, in protein synthesis, enzymes like aminoacyl-tRNA synthetases and peptidyl transferase facilitate the dehydration reactions that link amino acids together to form polypeptide chains. The mechanism by which enzymes influence dehydration synthesis involves several key steps. First, the enzyme binds to the substrates, positioning them in a way that optimizes the reaction. This binding often involves specific active sites on the enzyme that recognize and interact with the substrates. Once bound, the enzyme stabilizes the transition state of the reaction, lowering the activation energy required for bond formation. This stabilization can occur through various means, including hydrogen bonding, ionic interactions, or conformational changes that bring reactive groups closer together. Factors influencing the activity of these enzymes include pH, temperature, substrate concentration, and the presence of inhibitors or activators. Optimal pH and temperature conditions are critical for enzyme function; deviations from these conditions can denature the enzyme or reduce its activity. Substrate concentration affects the rate of reaction according to Michaelis-Menten kinetics, where increasing substrate concentration can increase the reaction rate until it reaches a maximum velocity (Vmax). Inhibitors can bind to the enzyme, either competitively or non-competitively, reducing its ability to catalyze the reaction. Conversely, activators can enhance enzyme activity by stabilizing the active conformation or increasing substrate affinity. In addition to these biochemical factors, molecular structure and evolutionary adaptations also influence enzyme function in dehydration synthesis. Enzymes have evolved to recognize specific substrates and catalyze reactions with high precision, minimizing errors and ensuring metabolic efficiency. For instance, the active site of an enzyme may be shaped to fit a particular substrate, allowing for precise positioning and orientation that facilitates the dehydration reaction. In summary, catalysts and enzymes are indispensable in dehydration synthesis, enabling these reactions to occur efficiently and accurately. Understanding the mechanisms by which these biological catalysts operate and the factors that influence their activity provides valuable insights into the intricate processes underlying cellular metabolism and biosynthesis. This knowledge is crucial for advancing fields such as biotechnology, medicine, and synthetic biology, where controlled dehydration reactions are essential for producing biomolecules with specific functions.
Environmental Conditions
Environmental conditions play a crucial role in the process of dehydration synthesis, influencing both the rate and efficiency of the reaction. Temperature is a key factor; optimal temperatures typically range between 20°C to 40°C, as higher temperatures can denature enzymes involved in the synthesis, while lower temperatures slow down the reaction rate. pH levels also significantly impact dehydration synthesis, with most reactions occurring optimally at a slightly acidic to neutral pH range (pH 6-7). This is because enzymes, which catalyze these reactions, have specific pH optima. Additionally, the presence of water is critical; although dehydration synthesis involves the removal of water molecules, an initial aqueous environment is necessary for the reactants to come into contact and for enzymes to function properly. However, excessive water can hinder the reaction by favoring hydrolysis over synthesis. Pressure and ionic strength can also affect enzyme activity and substrate availability, thereby influencing the overall yield of the reaction. Furthermore, the presence of cofactors and coenzymes can enhance enzyme activity under specific environmental conditions. Understanding these environmental factors is essential for optimizing dehydration synthesis in various biological and industrial contexts, ensuring that the conditions are tailored to maximize the efficiency and yield of the desired products. By controlling these variables, scientists and engineers can better harness the potential of dehydration synthesis in fields such as biochemistry, pharmaceuticals, and biotechnology.
Energy Requirements and Efficiency
Dehydration synthesis, a fundamental process in biochemistry, involves the formation of a new chemical bond between two molecules with the simultaneous loss of a water molecule. This reaction is crucial in various biological contexts, such as the synthesis of proteins, polysaccharides, and nucleic acids. However, the efficiency and feasibility of dehydration synthesis are significantly influenced by energy requirements. Energy requirements play a pivotal role in dehydration synthesis because these reactions often require an input of energy to proceed. This energy can come from various sources, including ATP (adenosine triphosphate), which is the primary energy currency of the cell. In many cases, dehydration synthesis reactions are coupled with ATP hydrolysis, where the energy released from breaking down ATP into ADP (adenosine diphosphate) and inorganic phosphate drives the formation of the new bond. For instance, during protein synthesis, amino acids are linked together through peptide bonds in a process that consumes ATP. Similarly, in the synthesis of glycogen from glucose, ATP is used to activate glucose molecules before they are added to the growing glycogen chain. The efficiency of dehydration synthesis is also a critical factor. Efficiency here refers to how effectively the reaction proceeds under given conditions, including temperature, pH, and the presence of catalysts such as enzymes. Enzymes, in particular, can significantly enhance the efficiency of dehydration synthesis by lowering the activation energy required for the reaction to occur. This allows the reaction to proceed faster and with greater specificity. For example, in the synthesis of DNA and RNA, enzymes like DNA polymerase and RNA polymerase facilitate the addition of nucleotides to the growing nucleic acid chain, ensuring high accuracy and speed. Additionally, environmental factors such as temperature and pH can influence the efficiency of dehydration synthesis. Optimal conditions for these reactions are typically those that are close to physiological conditions found within cells. Deviations from these optimal conditions can lead to reduced efficiency or even inhibition of the reaction. For instance, high temperatures can denature enzymes, rendering them inactive, while extreme pH levels can disrupt the stability of reactants and products. In summary, the energy requirements and efficiency of dehydration synthesis are interlinked and crucial for the successful formation of new chemical bonds. Understanding these aspects is essential for appreciating how cells manage to synthesize complex biomolecules efficiently and accurately. By leveraging ATP as an energy source and optimizing reaction conditions through enzymes and environmental control, cells ensure that dehydration synthesis reactions proceed with high efficiency, enabling the construction of vital biological structures.