What Makes Red
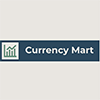
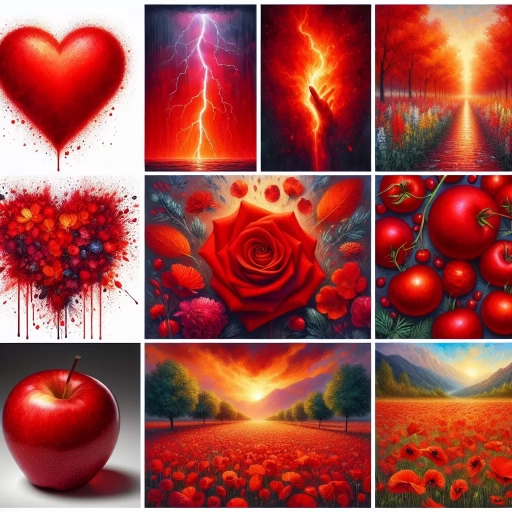
Chemical Composition and Structure
Chemistry, as an extensive branch of the Science tree, possesses deep roots across numerous fields, our focus in this article being Hemoglobin. We collaborate the aspects of chemistry with the human anatomy, diving headfirst into a microcosmic discussion on the formidable substance - Hemoglobin. This article lays out, simplistically, the chemical composition, structure, and importance of Hemoglobin in human bodies. Hemoglobin, a major component in red blood cells, plays a vital role in sustaining human life, significantly, making it a substance worthy of our attention. Furthermore, this article will focus on three major domains relating to Hemoglobin. Firstly, we shall delve into the presence of Hemoglobin, getting a prismatic outlook on the substance within us. Following that, we shall shift gears and venture into the role of Iron in Hemoglobin, understanding the binding force behind this life-maintaining compound. Lastly, the molecular structure of Hemoglobin, where we look into the nitty-gritty details, providing readers with a comprehensive understanding of this critical protein. Setting the stage for our exploration, let us commence with the ubiquitous presence of Hemoglobin in our bodies.
Presence of Hemoglobin
Hemoglobin, a complex, iron-containing compound, is the main component of red blood cells and gives them their characteristic color. Its primary role is transporting oxygen from the lungs to bodily tissues and carrying carbon dioxide for elimination through the lungs. This role finds its roots in its chemical composition and molecular structure. Hemoglobin comprises four protein subunits – two alpha and two beta. Each subunit is associated with a non-protein component, known as a heme group, which contains a centrally located, iron atom. The attraction of oxygen to the iron atom within the heme groups is what allows hemoglobin to carry oxygen. Interestingly, the chemical binding of oxygen changes the color of hemoglobin from a dark red to a bright red, explaining the range of red color we see in our blood. The iron atom can only bind one molecule of oxygen, allowing hemoglobin to carry up to four molecules of oxygen. When the iron atom loses its oxygen molecule, it binds to carbon dioxide for removal from the body. The presence of iron in hemoglobin not only is critical for oxygen transport but also influences the overall structure and function of the protein. Additionally, the hemoglobin molecule is a dynamic structure, capable of switching between a relaxed state (oxygen-rich) and a tense state (oxygen-poor), facilitating effective gas exchange throughout the body. This toggle between states showcases the importance of chemical composition and structure in hemoglobin's function. Hemoglobin's diverse roles and complex structure underline its importance to human life, and any deviations in its chemical composition can lead to significant health issues, such as anemia or sickle cell disease. Therefore, a comprehensive understanding of the chemical composition and structure of hemoglobin is essential in biomedical research and clinical practice.
Role of Iron in Hemoglobin
Iron plays a crucial role in the composition and structure of hemoglobin, a protein responsible for oxygen transport in the body. Forming the core of the heme group, which is a component of the globular hemoglobin protein, iron ensures the efficient transportation of oxygen from the lungs to the cells. Its key function lies in its capacity to transition between the reduced +2 (ferrous) and oxidized +3 (ferric) forms. In the reduced form, which exists in oxygen-rich environments such as the lungs, iron readily bonds with oxygen. As blood circulates to oxygen-deprived tissues, the environment triggers the iron to release the bonded oxygen. The iron then transitions from the ferrous to the ferric state, which has a lower affinity for oxygen. The iron atom's position within the heme pocket of the hemoglobin structure maintains this consistent cycle. Here, the iron atom is coordinated to a nitrogen atom from each of the four pyrrole rings, and then to an imidazole nitrogen from a histidine side chain of the protein, forming a pentagonal bipyramidal geometry. Centrally positioned, the iron atom is thus perfectly poised to accept and release oxygen, thereby maintaining the body's blood oxygen requirements. Despite occupying a small area within the massive hemoglobin molecule, the impact of iron is substantial. It plays a fundamental role in the cycle of oxygen transportation and release; a cycle that is vital for survival. The body is highly dependent on iron, highlighting the importance of maintaining the right levels of iron intake to sustain the hemoglobin's function. Furthermore, alterations or misalignments in the iron's placement can lead to conditions such as methemoglobinemia, where iron gets oxidized to the ferric state, impeding its ability to carry oxygen. This underlines the significance of iron's precise positioning within the hemoglobin structure. In conclusion, the role of iron in hemoglobin, albeit subtle, is incredibly critical. Beyond its role in the fundamental oxygen transportation cycle, science is still unveiling the many ways iron's exquisite positioning and chemical switching capabilities serve our bodies. Undeniably, iron's place within the hemoglobin structure is a testament to nature's intelligent and intricate design.
Molecular Structure of Hemoglobin
Hemoglobin, a vital protein present in the red blood cells of humans and many vertebrates, is responsible for the transportation of oxygen from the lungs to the tissues and also aids in the movement of carbon dioxide from the tissues to the lungs. Molecularly, hemoglobin is a tetramer composed of two alpha (α) and two beta (β) polypeptides, each embracing an intricate globin fold and an embedded heme group. These globin subunits, bearing a molecular weight of about 16,000 Da each, have multiple hydrophobic residues that stabilize the heme group. The heme group, a coordination complex comprising an iron ion (Fe2+) housed in a large heterocyclic organic ring called a porphyrin, is the active site of hemoglobin which binds to oxygen. The iron ion under normal physiological conditions can either exist in a reduced state (Fe2+) enabling it to bind reversibly with oxygen, or an oxidized state (Fe3+), which, albeit stable, inhibits the oxygen-carrying function due to its preference for binding water instead of oxygen. In the context of chemical composition, the alpha and beta subunits of hemoglobin are distinct in their amino acid sequences, but they are homologous in structure, signifying that they evolved from a common genetic ancestor. The alpha chain comprises 141 amino acid residues while the beta chain has 146. Each subunit has eight alpha-helices labeled A through H. The sequences of these helices, the connections among them and their orientations with respect to each other are preserved in all four subunits. Intriguingly, the hemoglobin molecule experiences a transition in structure when it binds and releases oxygen – shifting between the relaxed state, also known as the "R-state" where it has a high affinity for oxygen, and the tense state, or the "T-state", where it has a low affinity for oxygen. This structural transition, also referred to as the "allosteric transition", is facilitated by changes at the molecular level. At high oxygen concentrations, as in the lungs, hemoglobin molecules are more likely to be in the R-state, and thus readily binds to oxygen. Conversely, at low oxygen concentrations, as in the tissues, hemoglobin reverts to the T-state, promoting the release of oxygen. In conclusion, the chemical composition and structure of hemoglobin play a significant role in its functionality and efficiency in carrying out its primary task, which is the transportation of oxygen and carbon dioxide within the body. Its unique tetramer configuration, the intricately folded alpha and beta globins housing the heme groups and the conformational changes it undergoes in tandem with the fluctuating concentrations of oxygen, collectively contribute to its remarkable, life-sustaining properties.
Biological Functions and Importance
Residing at the heart of existence, the biological functions and their importance indisputably form the bedrock of life on Earth. Essential mechanisms such as the transport of oxygen and carbon dioxide, as well as the regulation of pH levels in blood, largely contribute to the seamless workings of this intricate life-machinery. Oxygen transport reigns supreme, acting as the cardinal lifesaver, fueling our cells and mitigating cellular death while ensuring that every breath we take counts. Meanwhile, the transport of carbon dioxide, a by-product of cellular respiration, is pivotal in maintaining the body's balance, preventing harmful build-ups and detoxifying our systems. Further, our bodies masterfully regulate pH levels in blood, a true testament to the inherent balance in nature. This vital function safeguards a stable environment for cells, ensuring the optimal performance of physiological processes. This symphony of biological processes illustrates the masterstroke of nature's design, where every cog plays a significant role in maintaining life. With that, let's delve deeper and examine the intricately designed process of Oxygen Transport Mechanism, essential for cellular energy and essentially, life itself.
Oxygen Transport Mechanism
Blood vital, Oxygen. Not just polychrome pigmentation red; red blood cells embody our very livelihood, an intricate ballet of oxygen transport that sustains connective tissue of life. This refrigerant tour de force unfolds in capillaries, the body’s minutest blood vessels where red blood cells -- the star acrobats of oxygen transport -- deftly pass along life-sustaining oxygen. A singular red blood cell has the daunting task of spiriting oxygen from lungs to disparate parts of the body, an operation accomplished with artful finesse and unparalleled precision. At the heart of this complex operation lies hemoglobin, a pigment replete with iron, contained within red cells. This biomolecule binds to inhaled oxygen in lung capillaries, crafting oxyhemoglobin, an oxygen-iron compound gifted at fueling a lean, mean machine of a body. Red blood cells act as an efficient transport system, carrying this much-needed oxygen to body tissues on a global dispatch. Once they reach oxygen-starved cells, a chemical reaction takes place; the oxygen molecule disassociates from the hemoglobin, transferring into cells, revving up cellular activity, and catalyzing metabolism – swap out for carbohydrates, and you have ATP, the body's powerhouse energy. Ensuring this oxygen transportation is key to maintaining optimal organ function, with a disruption potentially spelling disaster for the host body. Oxygen hunger, or hypoxia, can plunge the body into a predicament; meteoric heart rate, severe headaches, confusion, lack of coordination – the fallout of compromised oxygen delivery. Conversely, Oxygen excess, or hyperoxia, can boost free radicals, inducing damage to cell structures. But the red blood cells are tireless voyagers; once they offload oxygen to cells, they dutifully pick up carbon dioxide - the cell's waste product - hauling it back to lungs and off into the atmosphere at the exhale. A subtle albeit striking balance of offloading life-essential oxygen while clearing the carbon dioxide clutter - an intricate minuet sustaining our very existence. This fascinating transference of oxygen is a testament to nature’s ingenious adaptability. The enveloping phospholipid layer of these cells gifts them flexibility, allowing the red cells to contort and squeeze through the narrowest capillaries, conserving their precious oxygen cargo. Also, the disks' concave shape broadens the cell’s surface area, ensuring maximum oxygen pick-up. The nuances of oxygen transport mechanism are a bewildering marvel in and of themselves. Yet they represent just a fraction of the sheer symphony of activities that take place within our bodies every single moment, silent testament to the staggering complexity inherent in the very fabric of life itself. This function serves as an exquisite reminder of our intricate biology and the importance of maintaining this delicate biological balance for systematic health.
Carbon Dioxide Transport
Carbon dioxide transport is a crucial biological function that highlights the importance of red blood cells. This process is part of the respiratory cycle wherein the body eliminates waste material, primarily excess carbon dioxide. Essentially, during the metabolic processes within the cells, carbon dioxide is produced as a waste product. The transportation of this waste product from the body tissues to the lungs, where it is exhaled out, is carried out in the blood plasma or bound to hemoglobin in red blood cells. The metabolic process that generates carbon dioxide takes place in the cells' mitochondria, where glucose is broken down to provide energy to the body—leaving CO2 and water as byproducts. Carbon dioxide produced inside the cells diffuses passively across cell membranes into the interstitial fluid and the capillary blood stream, as a result of the existing concentration gradient. It can then reach the red blood cells present in the blood stream, binding with water to form carbonic acid. This reaction is facilitated by an enzyme known as carbonic anhydrase, further demonstrating the innate complexity and sophistication of cellular functions. Around 10% of carbon dioxide remains dissolved directly in the plasma, while the majority within the red blood cells is rapidly converted into bicarbonate (HCO3-) and hydrogen ions (H+) through the dissociation of carbonic acid (H2CO3). This transformation allows an efficient method of transport for the carbon dioxide, as it can now move easier through the bloodstream. Moreover, some quantity of carbon dioxide binds directly to hemoglobin forming carbaminohemoglobin, enabling hemoglobin to serve a dual role – transport of oxygen from lungs to tissues and carbon dioxide from tissues to lungs. Once the blood arrives in the lungs, the processes are reversed—bicarbonate and hydrogen ions recombine to form carbonic acid, and then into carbon dioxide and water, in a reaction catalyzed once again by carbonic anhydrase. This CO2 then diffuses out of the red blood cells, across the respiratory membrane, and into the alveoli, from where it is disposed of from our body via exhalation. The importance of this process—Carbon dioxide transport—lies in its role of removing from our bodies the carbon dioxide, a waste product of metabolism, which otherwise would create an imbalance in the pH levels and pose serious health risks. This biological function also demonstrates the interrelationship between different systems within the body – specifically, the circulatory and respiratory systems. Moreover, the specialized role of red blood cells and the protein hemoglobin in this process—besides their oxygen-transporting function—testify to the intricacy of our bodily functions and the key role of these cells offer beyond what is commonly known. Thus, illustrating the diverse roles cells play in maintaining homeostasis, further highlighting their valuable contribution to life sustainability.
Regulation of pH Levels in Blood
The regulation of pH levels in the blood is an essential biological function that helps to maintain a stable internal environment within the human body, thereby ensuring optimal health and wellbeing. The pH of the blood signifies the balance between the acidity and alkalinity of the body and is generally maintained at a slightly alkaline level, between 7.35 and 7.45. The regulation of these pH levels is primarily controlled by the respiratory and renal systems. The respiratory system achieves this by regulating the levels of carbon dioxide in the bloodstream, while the renal system does this by controlling the amount of bicarbonate ions in the blood. Together, these systems work to reduce the acidity in the blood, thus regulating its pH. In situations where there is an excess of acidity in the blood, a condition known as acidosis might occur. Conversely, when there is an excess of alkalinity in the blood, a condition called alkalosis might ensue. These conditions can negatively affect the functioning of proteins, enzymes, and other biochemical processes, leading to potential health trouble if not properly rectified. Therefore, it is imperative that these two systems work in harmony to sustain the ideal blood pH levels, as this is crucial for the effective functioning of the human body. The constant monitoring and intricate adjustments done by these systems daily underscore the importance of pH regulation in maintaining physiological homeostasis. Hence, the regulation of blood pH is not only essential for the efficient metabolic functioning of body systems but also as a defense mechanism against potential threats that might compromise the body's health. Regular monitoring of blood pH through blood tests is recommended to ensure balance and support overall health and wellbeing. Thus, pH regulation's integral role in human biology emphasizes its significance and gravity.
Clinical Significance and Health Implications
The inevitable intertwining between Clinical Significance and Health Implications is evident in various aspects of medicine. Highlighting this crucial relationship, this article delves into three core areas of concern. Firstly, it sheds light on the diagnosis of anemia and other related blood disorders, focusing on not only their clinical implication but also the broader impact on health. Secondly, it analyzes the vital process of oxygen delivery to tissues and its indicative role in diagnosing and monitoring health conditions. Lastly, it explores the significant influence of these medically salient issues on athletic performance and endurance, illustrating how comprehensive clinical information can enhance understanding of athletic capacity and inform training strategies. Positioned at this intersection of clinical knowledge and health outcomes, this article strives to present a holistic, coherent, and medical fact-backed understanding of these topics. Without further ado, let us delve into the first topic of discussion: diagnosis of anemia and other affiliated blood disorders. Here, we explore not only the clinical approach towards these conditions but also their broader implications for healthcare systems around the world.
Diagnosis of Anemia and Other Blood Disorders
Diagnosing anemia and other blood disorders entails a detailed process comprising adequate assessment and various testing methodologies. Becoming informed and engaged in understanding anemia or any other blood disorder's diagnosis is crucial, as it is the stepping stone to appropriate, efficient, and successful treatment. The initial diagnosis phase often comprises of medical and lifestyle histories, emphasizing any pathological conditions like renal disease, dietary habits, family medical history, and any prevalent anemic symptoms. This is followed by thorough physical examinations focussed on visible anemic signs such as pallor, jaundice, and tachycardia. However, a definitive diagnosis heavily relies on lab tests. Typically, Complete Blood Count (CBC), an essential diagnostic tool in hematological disorders, is utilized for initial screening. CBC's different constituents such as Hemoglobin (Hb) level, Mean Corpuscular Volume (MCV), Mean Corpuscular Hemoglobin (MCH), and Mean Corpuscular Hemoglobin Concentration (MCHC) offer critical insights which assist in distinguishing between various anemia types. For instance, Microcytic anemia (lower MCV) could suggest Iron Deficiency or Thalassemia, whereas Macrocytic anemia (increased MCV) could indicate Vitamin B12 or Folate Deficiency. Additionally, to further refine the diagnosis, other tests like peripheral blood smear, serum iron studies, reticulocyte count, serum vitamin B12 level, haptoglobin, and others can be used based on initial findings. For instance, serum ferritin test aids in diagnosing iron deficiency anemia, while bone marrow biopsy could be beneficial in diagnosing aplastic anemia. It is also crucial to remember that diagnosing blood disorders can be tricky since several conditions often share similar traits and symptoms. In such cases, a collective approach involving differential diagnosis, which consists of eliminating potential disorders or diseases in view of the laboratory findings and clinical signs, is employed. Undoubtedly, timely diagnosis and treatment are critical to prevent potential complications and mitigate the health implications of anemia and other blood disorders. They have significant clinical relevance as they affect millions of individuals globally and can lead to severe health complications if undiagnosed or untreated. It can lead to reduced quality of life, impaired cognitive and physical development in children, increased surgical mortality, and reduced work productivity in adults. Additionally, they can also be indicative of underlying chronic diseases sometimes. Thus, robust and accurate diagnostic process forms the backbone of efficacious clinical management of anemia and other blood disorders. Further research and advancements in diagnostic technologies and methodologies are essential to ensure improved patient outcomes.
Impact on Oxygen Delivery to Tissues
The complex circulatory systems in humans play a vital role in the distribution of oxygen throughout the body, which is critical for maintaining life. Red blood cells, the primary carriers of oxygen, accomplish this task through a protein they contain called hemoglobin. It has been observed that a decrease in oxygen delivery, either through a reduction in the number of red blood cells or problems with the hemoglobin itself, can profoundly affect the overall health of an individual. Oxygen delivery is not only dependent on its successful loading onto red blood cells in the lungs, but also its efficient offloading to the tissues where it is needed. Factors such as age, disease, and environmental conditions can significantly impact the affinity of hemoglobin for oxygen, resulting in inadequate oxygen delivery to the tissues. This affinity is finely balanced; high affinity can lead to oxygen being retained by the hemoglobin, preventing its delivery to the tissues, while low oxygen affinity may result in premature offloading before the red blood cells reach tissue in need. Anemia, for instance, reduces red blood cells' capability to deliver oxygen to various tissues in the body, leading to fatigue, paleness, and shortness of breath. Similarly, conditions such as sickle cell disease, where the shape of the red blood cells is distorted, can impair oxygen delivery and lead to severe pain crises and organ damage. In contrast, high altitudes, with low oxygen levels, stimulate the body to produce more red blood cells, enhancing the body’s oxygen-carrying capacity and partially compensating for the lower oxygen levels available in the environment. Furthermore, the efficiency of oxygen delivery to tissues can be influenced by the metabolic activity of the cells themselves. Highly active cells, such as those in skeletal muscle during exercise, secrete metabolites that lower the affinity of hemoglobin for oxygen, enhancing oxygen offloading where it is most needed. In summary, oxygen delivery to tissues is an essential physiological process that can be influenced by numerous factors. Understanding the mechanisms involved in oxygen delivery can lead to better diagnoses and medical interventions for conditions that impact this critical bodily function. Therefore, the health implications are significant, emphasizing the importance of ongoing research in this field to advance the understanding and treatment of blood and circulatory disorders.
Role in Athletic Performance and Endurance
The role of red blood cells in athletic performance and endurance is pivotal, given their function in the body's oxygen transport system. In the realm of physical exertion, oxygen efficiency is key to sustaining prolonged periods of exercise, a concept widely understood amongst athletes and trainers. Red blood cells are responsible for carrying oxygen from the lungs to the muscles which, coupled with glucose, generates the energy necessary for muscle contraction that enables physical activity. In essence, the greater the red blood cell count, the more oxygen is available to the muscles, thereby improving athletic performance and endurance. Athletes professionally involved in endurance sports, such as long-distance running or cycling, often undergo altitude training. A stay in high-altitude locations induces the body to produce more red blood cells to compensate for the lower oxygen saturation in the environment, leading to an increased oxygen-carrying capacity upon returning to lower elevations. However, this strategy should be undertaken with care, as excessive red blood cells can lead to a condition called polycythemia, potentially causing blood clots due to thickened blood. Iron, a vital component of red blood cells, is necessary for their production and function. Iron deficiencies can impair athletic performance, manifesting in symptoms such as fatigue, weakness, and decreased endurance. Furthermore, physically active individuals, notably women and vegetarians, are at a higher risk of iron deficiency. This highlights the importance of maintaining an iron-rich diet or considering supplementation under professional guidance. Understanding the role and significance of red blood cells in athletics not only optimizes performance but also helps in maintaining good health. Fluctuating red blood cell levels could indicate a medical condition such as anemia or dehydration, both of which can severely impact performance and overall health. Therefore, regular blood tests are crucial for athletes to monitor their red blood cell count and iron levels, ensuring both their competitive edge and wellbeing. Moreover, further research into genetic factors influencing red blood cell production could open new avenues for personalized training and nutrition plans, paving the way for a new era of tailored sports science. With the obvious clinical significance and health implications of red blood cell count and function, it is paramount for anyone involved in sport to understand the relationship between athletic performance, endurance, and their blood health. In a broader context, this underscores the intricate interplay between physical activity, nutrition, and human physiology, adding depth to our comprehension of the body's capabilities and limitations.