What Is Eit
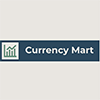
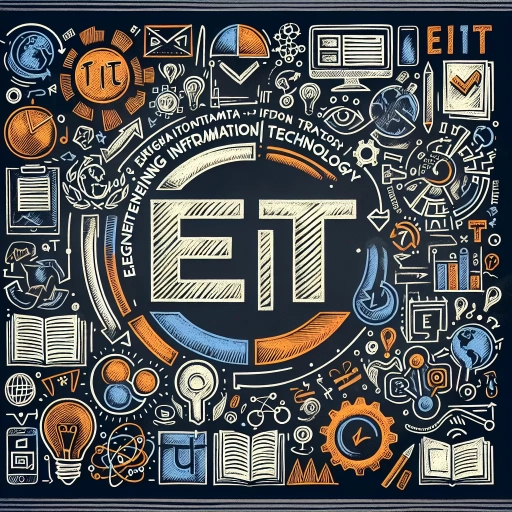
Electrical Impedance Tomography (EIT) is a non-invasive imaging technique that has garnered significant attention in various fields due to its unique ability to visualize the internal electrical properties of a body. This innovative method involves applying small electrical currents to the body and measuring the resulting voltages to create detailed images. In this article, we will delve into the multifaceted aspects of EIT, starting with **Understanding the Basics of EIT**, where we will explore the fundamental principles and mechanisms behind this technology. We will then discuss **Applications and Uses of EIT**, highlighting its diverse range of uses in medical diagnostics, industrial monitoring, and other sectors. Finally, we will examine **Technological Advancements and Future Directions**, shedding light on the latest developments and potential future applications of EIT. By understanding these core aspects, readers will gain a comprehensive insight into the capabilities and potential of this groundbreaking technology. Let us begin by understanding the basics of EIT.
Understanding the Basics of EIT
Understanding the Basics of Electrical Impedance Tomography (EIT) is crucial for grasping its applications and significance in various fields, particularly in medical imaging and industrial process monitoring. To delve into the fundamentals of EIT, it is essential to explore three key aspects: its definition and origins, the key principles and concepts that underpin the technology, and its historical development. Starting with the **Definition and Origins**, we will examine what EIT is, how it works, and where it originated. This foundational knowledge sets the stage for understanding the broader implications and advancements of the technology. Next, we will delve into the **Key Principles and Concepts**, which include the electrical properties of tissues, the reconstruction algorithms used to create images, and the various types of EIT systems. These principles are vital for comprehending how EIT translates electrical measurements into visual representations. Finally, the **Historical Development** of EIT will provide insight into how this technology has evolved over time, from its early beginnings to current applications and future prospects. By understanding these three components, readers will gain a comprehensive view of EIT's role and potential. Let's begin by defining what EIT is and tracing its origins to understand the roots of this innovative technology.
Definition and Origins
**Understanding the Basics of EIT: Definition and Origins** Electrical Impedance Tomography (EIT) is a non-invasive medical imaging technique that reconstructs the internal electrical conductivity of a body from surface measurements. The definition of EIT revolves around its ability to generate images of the internal distribution of electrical properties within the body, such as conductivity and permittivity, by applying small currents and measuring the resulting voltages at the surface. This method is particularly useful in monitoring dynamic processes like breathing or cardiac activity in real-time. The origins of EIT date back to the 1970s when it was first proposed by John G. Webster, a biomedical engineer. However, significant advancements were made in the 1980s by researchers such as David C. Barber and Brian H. Brown at the University of Sheffield. They developed the first practical EIT systems and algorithms, laying the groundwork for modern applications. The technique gained traction due to its potential in providing continuous, bedside monitoring without the need for ionizing radiation, making it an attractive alternative to traditional imaging modalities like X-rays or CT scans. EIT's development has been driven by advances in electronics, signal processing, and computational algorithms. Modern EIT systems typically consist of an array of electrodes placed around the body part of interest, an electrical current source, and sophisticated software for data acquisition and image reconstruction. The process involves applying a small alternating current through some of these electrodes while measuring the resulting voltages at others. These measurements are then used to reconstruct cross-sectional images of the internal conductivity distribution using inverse problem-solving techniques. The versatility of EIT has led to its application in various clinical settings, including pulmonary function monitoring, cardiac function assessment, and even in some cases of cancer detection. Its non-invasive nature and real-time capability make it particularly valuable in intensive care units (ICUs) where continuous monitoring is crucial. Despite its advantages, EIT faces challenges such as limited spatial resolution compared to other imaging modalities and the need for further research to fully exploit its potential. In summary, EIT is a dynamic imaging technique with roots in the pioneering work of the 1970s and 1980s. Its definition centers on reconstructing internal electrical properties from surface measurements, offering a unique tool for real-time monitoring without radiation exposure. As technology continues to evolve, EIT holds promise for expanding its clinical applications and improving patient care.
Key Principles and Concepts
Understanding the basics of Electrical Impedance Tomography (EIT) hinges on several key principles and concepts that underpin its functionality and application. At its core, EIT is a non-invasive imaging technique that measures the electrical impedance of tissues within the body. Here are the fundamental principles: 1. **Electrical Impedance**: This refers to the opposition that a circuit presents to a current when a voltage is applied. In biological tissues, impedance varies significantly depending on the type of tissue and its condition, making it a valuable diagnostic tool. 2. **Current Injection and Voltage Measurement**: In EIT, small currents are injected into the body through electrodes placed on the skin surface. The resulting voltage changes are then measured at other electrodes. This process is repeated multiple times with different electrode configurations to gather comprehensive data. 3. **Inverse Problem**: The raw data collected from voltage measurements needs to be processed to reconstruct images of internal impedance distributions. This involves solving an inverse problem, where the goal is to infer the internal conductivity distribution from boundary measurements. 4. **Algorithms and Reconstruction Techniques**: Various algorithms, such as the finite element method (FEM) and linear back-projection, are employed to reconstruct images from the measured data. These algorithms must account for the complex geometry of the body and the non-linear relationship between impedance and voltage. 5. **Electrode Placement and Configuration**: The placement and configuration of electrodes are crucial for obtaining accurate measurements. Common configurations include circular arrays around the body part being imaged, with each electrode serving as both a current injector and a voltage sensor at different times. 6. **Frequency Dependence**: Biological tissues exhibit frequency-dependent impedance characteristics, which can provide additional diagnostic information. For instance, different frequencies can highlight different tissue properties, such as cellular structure or fluid content. 7. **Safety and Limitations**: EIT is generally safe due to the low currents used, but it has limitations in terms of spatial resolution and depth penetration compared to other imaging modalities like MRI or CT scans. However, its non-invasive nature and real-time capabilities make it particularly useful for monitoring dynamic processes like breathing or cardiac function. 8. **Clinical Applications**: EIT has various clinical applications, including monitoring lung function in critically ill patients, detecting breast tumors, and assessing cardiac output. Its ability to provide continuous, real-time data makes it invaluable for bedside monitoring and research purposes. 9. **Technological Advancements**: Advances in hardware and software have significantly improved the performance of EIT systems. High-speed data acquisition systems and sophisticated reconstruction algorithms have enhanced image quality and reduced artifacts, making EIT more reliable and versatile. 10. **Research and Development**: Ongoing research aims to further improve EIT's spatial resolution, expand its range of applications, and integrate it with other imaging modalities for more comprehensive diagnostics. This includes exploring new electrode designs, advanced signal processing techniques, and combining EIT with other imaging methods like ultrasound or MRI. Understanding these principles and concepts is essential for appreciating the capabilities and limitations of EIT, as well as its potential to contribute significantly to medical diagnostics and research.
Historical Development
The historical development of Electrical Impedance Tomography (EIT) is a fascinating narrative that underscores the evolution of medical imaging technologies. EIT, which involves measuring the electrical impedance of tissues within the body, has its roots in the late 19th century when scientists first began exploring the electrical properties of biological tissues. However, it wasn't until the 1970s that the concept of EIT as we know it today started to take shape. In the early 1970s, researchers like David C. Barber and Brian H. Brown at the University of Sheffield pioneered the development of EIT. They recognized the potential for using electrical impedance measurements to create images of internal body structures, which could complement or even replace traditional imaging modalities like X-rays and ultrasound. The first practical EIT systems were developed in the 1980s, utilizing arrays of electrodes placed around the body to inject small currents and measure the resulting voltages. Throughout the 1990s and 2000s, advancements in computational algorithms and hardware significantly improved EIT's resolution and accuracy. The introduction of more sophisticated reconstruction techniques, such as the finite element method, allowed for better image quality. Additionally, advancements in data acquisition systems enabled faster and more reliable measurements, making EIT a viable tool for real-time monitoring in clinical settings. One of the key milestones in EIT's development was its application in critical care medicine. In the early 2000s, researchers began using EIT to monitor lung function in patients with acute respiratory distress syndrome (ARDS). This application highlighted EIT's ability to provide continuous, non-invasive monitoring of vital organ function, which is crucial for timely clinical interventions. Today, EIT continues to evolve with ongoing research focusing on improving image resolution, expanding its clinical applications, and integrating it with other imaging modalities. For instance, combining EIT with other techniques like MRI or CT scans can enhance diagnostic capabilities by providing both functional and anatomical information simultaneously. Moreover, advancements in wearable technology and miniaturization are paving the way for portable EIT devices that could revolutionize point-of-care diagnostics. Understanding these historical developments is essential for appreciating the current state and future potential of EIT. From its humble beginnings as a theoretical concept to its current status as a valuable clinical tool, EIT's journey reflects the collaborative efforts of scientists, engineers, and clinicians working towards better patient care through innovative medical imaging solutions.
Applications and Uses of EIT
Electrical Impedance Tomography (EIT) is a versatile and non-invasive imaging technique that has found diverse applications across various fields. This technology leverages the principle of measuring the electrical impedance of tissues or materials to create detailed images or monitor processes. In the realm of medical imaging and diagnostics, EIT offers a promising tool for real-time monitoring of internal organs and tissues, aiding in the diagnosis and treatment of conditions such as lung diseases and cardiac issues. Beyond healthcare, EIT is also employed in industrial process monitoring, where it helps in tracking the flow of materials and detecting anomalies in real-time, enhancing efficiency and safety. Additionally, environmental and geophysical surveys benefit from EIT as it allows for the mapping of subsurface structures and monitoring of soil moisture, crucial for geological research and environmental monitoring. Each of these applications underscores the flexibility and utility of EIT, making it an indispensable tool in modern science and technology. Transitioning to its medical applications, EIT's role in medical imaging and diagnostics is particularly noteworthy, as it provides healthcare professionals with a valuable diagnostic tool that is both safe and effective.
Medical Imaging and Diagnostics
Medical imaging and diagnostics play a crucial role in modern healthcare, enabling clinicians to visualize the internal structures of the body and diagnose a wide range of conditions with precision. This field encompasses various technologies, each with its unique strengths and applications. Magnetic Resonance Imaging (MRI) and Computed Tomography (CT) scans are among the most commonly used modalities, providing detailed images of soft tissues and internal organs. MRI is particularly useful for diagnosing neurological disorders, joint and musculoskeletal conditions, while CT scans are often employed for detecting cancers, vascular diseases, and injuries. Ultrasound imaging is another vital tool, especially in obstetrics and cardiology, offering real-time images without the use of ionizing radiation. Positron Emission Tomography (PET) scans combine with CT to provide metabolic information about tissues, making them invaluable in oncology for staging cancers and monitoring treatment response. X-ray imaging remains a cornerstone for diagnosing bone fractures and lung diseases. In addition to these traditional methods, emerging technologies like Electrical Impedance Tomography (EIT) are gaining traction. EIT involves measuring the electrical conductivity of tissues to create images, which can be particularly useful in monitoring dynamic processes such as lung function or cardiac activity in real-time. This non-invasive technique is especially beneficial in intensive care settings where continuous monitoring is critical. The applications of medical imaging extend beyond diagnosis to include treatment planning and follow-up care. For instance, imaging-guided interventions allow for precise targeting of tumors during radiation therapy or biopsies. Moreover, advanced imaging techniques like functional MRI (fMRI) and diffusion tensor imaging (DTI) provide insights into brain function and connectivity, aiding in the management of neurological conditions. In the context of EIT, its unique ability to monitor changes in tissue impedance makes it an attractive tool for various clinical applications. For example, EIT can be used to assess lung function in patients with respiratory diseases such as chronic obstructive pulmonary disease (COPD) or acute respiratory distress syndrome (ARDS). It can also monitor cardiac function post-surgery or during critical care scenarios where continuous monitoring is essential. Overall, medical imaging and diagnostics are indispensable components of modern healthcare, offering a range of tools that enhance diagnostic accuracy, guide treatment decisions, and improve patient outcomes. As technologies continue to evolve, including the integration of EIT into clinical practice, healthcare providers will have even more sophisticated means to diagnose and manage complex medical conditions effectively.
Industrial Process Monitoring
Industrial process monitoring is a critical aspect of modern manufacturing, ensuring the efficiency, safety, and quality of various industrial processes. Electrical Impedance Tomography (EIT) plays a significant role in this domain due to its non-invasive, real-time, and cost-effective nature. EIT involves measuring the electrical impedance of a medium to reconstruct images of its internal structure, which is particularly useful in monitoring processes involving multiphase flows, such as gas-liquid or solid-liquid mixtures. In chemical engineering, EIT helps monitor the mixing of reactants in reactors, ensuring uniform distribution and optimal reaction conditions. This is crucial for maintaining product quality and preventing unwanted side reactions. In oil and gas industries, EIT can be used to monitor the flow of different phases within pipelines, detecting blockages or phase separations that could lead to operational issues. Similarly, in food processing, EIT can monitor the mixing and blending of ingredients, ensuring consistent product quality. In power generation, particularly in coal-fired plants, EIT can monitor the combustion process within boilers, optimizing fuel efficiency and reducing emissions. It also helps in detecting anomalies such as slag buildup or uneven fuel distribution. In pharmaceutical manufacturing, EIT ensures that mixing processes adhere to strict regulatory standards by providing real-time data on the homogeneity of mixtures. Moreover, EIT's ability to operate in harsh environments makes it suitable for monitoring processes in extreme conditions, such as high temperatures or pressures. This versatility extends its application to various other industries including mining, where it can monitor the flow of slurries and detect potential blockages, and in wastewater treatment, where it helps in optimizing the mixing of chemicals for effective treatment. The real-time data provided by EIT allows for immediate adjustments to be made to the process, enhancing overall efficiency and reducing downtime. Additionally, the non-invasive nature of EIT means that it does not disrupt the ongoing process, making it an ideal tool for continuous monitoring without compromising production. Overall, the integration of EIT into industrial process monitoring enhances operational reliability, improves product quality, and contributes significantly to the optimization of industrial processes across diverse sectors.
Environmental and Geophysical Surveys
Environmental and geophysical surveys are crucial tools in understanding and managing the Earth's subsurface and surface environments. These surveys involve a range of techniques, including Electrical Resistivity Tomography (ERT), Ground-Penetrating Radar (GPR), and Electromagnetic (EM) surveys, which are often integrated with Electrical Impedance Tomography (EIT) to provide comprehensive data. In environmental applications, these surveys help in monitoring soil and groundwater contamination, identifying potential pollution sources, and assessing the effectiveness of remediation efforts. For instance, ERT can map the distribution of contaminants in the subsurface by measuring electrical resistivity variations, while GPR can detect buried tanks or other subsurface structures that may be sources of pollution. EM surveys are particularly useful for mapping large areas quickly to identify zones of high conductivity indicative of contamination. Geophysically, these surveys are essential for understanding geological structures and processes. They aid in the exploration of mineral and hydrocarbon resources by imaging subsurface rock formations and fluid distributions. Seismic surveys, often used in conjunction with EIT, help in detailed mapping of subsurface layers and identifying potential reservoirs. Additionally, geophysical surveys are vital in natural hazard assessment, such as detecting subsurface cavities or unstable rock formations that could lead to landslides or earthquakes. In the context of EIT, these surveys enhance the resolution and accuracy of subsurface imaging. By combining EIT with other geophysical methods, researchers and practitioners can achieve a more detailed understanding of complex subsurface environments. For example, in hydrogeological studies, integrating EIT with ERT can provide real-time monitoring of groundwater flow and contaminant transport, allowing for more effective management of aquifers. Moreover, environmental and geophysical surveys support urban planning and infrastructure development by identifying suitable sites for construction and assessing the stability of existing structures. They also play a critical role in archaeological investigations, helping to locate and map buried sites without invasive excavation. Overall, environmental and geophysical surveys are indispensable for a wide range of applications, from environmental monitoring and resource exploration to natural hazard mitigation and urban planning. When combined with EIT, these surveys offer unparalleled insights into the Earth's subsurface, enabling more informed decision-making across various disciplines.
Technological Advancements and Future Directions
Technological advancements are transforming various aspects of our lives, from how we gather data to how we analyze and apply it. This article delves into three critical areas that are driving these changes: advances in sensor technology, improvements in data analysis algorithms, and potential future applications and innovations. Advances in sensor technology have enabled the collection of more precise and diverse data, setting the stage for significant improvements in fields such as healthcare, environmental monitoring, and industrial automation. Improvements in data analysis algorithms have enhanced our ability to extract meaningful insights from this data, leading to better decision-making and more efficient processes. Looking ahead, potential future applications and innovations promise to revolutionize industries and daily life even further. By exploring these areas, we can gain a deeper understanding of the current state of technological progress and the exciting possibilities that lie ahead. Let us begin by examining the groundbreaking developments in sensor technology, which form the foundation for many of these advancements.
Advances in Sensor Technology
Advances in sensor technology have revolutionized various industries, from healthcare and manufacturing to transportation and environmental monitoring. One of the most significant developments is the miniaturization of sensors, enabling their integration into wearable devices, smartphones, and even implantable medical devices. These tiny sensors can monitor vital signs, track physical activity, and detect health anomalies with unprecedented precision. For instance, wearable heart rate monitors and blood glucose sensors provide real-time data that can be crucial for managing chronic conditions. Another critical advancement is the enhancement of sensor accuracy and reliability through advancements in materials science and nanotechnology. New materials like graphene and nanocrystals have improved sensor sensitivity, allowing for the detection of minute changes in temperature, pressure, and chemical composition. This has been particularly beneficial in industrial settings where precise monitoring of equipment health can prevent costly failures and enhance overall efficiency. The integration of artificial intelligence (AI) and machine learning (ML) algorithms with sensor data has also transformed the landscape. AI-driven sensors can analyze vast amounts of data in real-time, identifying patterns and anomalies that human operators might miss. In smart cities, for example, AI-powered sensors monitor traffic flow, air quality, and energy consumption to optimize urban planning and resource allocation. Furthermore, the advent of Internet of Things (IoT) technology has connected sensors across diverse platforms, facilitating seamless data exchange and collaborative decision-making. IoT sensors in agricultural fields can monitor soil moisture, temperature, and crop health, enabling farmers to adopt precision agriculture practices that maximize yield while minimizing resource usage. In addition, advancements in wireless communication technologies such as Bluetooth Low Energy (BLE) and 5G have significantly improved the connectivity and power efficiency of sensors. This has made it possible to deploy large-scale sensor networks without the need for extensive wiring or frequent battery replacements. Looking ahead, future directions in sensor technology include the development of more sophisticated biometric sensors capable of detecting subtle physiological changes indicative of early-stage diseases. Quantum sensors are also on the horizon, promising even greater precision in fields like navigation and geophysics. As these technologies continue to evolve, they will drive further innovation across multiple sectors, enhancing our ability to monitor, analyze, and respond to complex systems in real-time. Ultimately, these advancements will pave the way for smarter, more sustainable solutions that improve both individual lives and global well-being.
Improvements in Data Analysis Algorithms
The advancements in data analysis algorithms have revolutionized the way we interpret and utilize data, significantly enhancing the efficiency and accuracy of various technological applications. One of the most notable improvements is the development of machine learning algorithms, which have become increasingly sophisticated. These algorithms, such as deep learning models, can now handle complex datasets with unprecedented precision, enabling applications like image recognition, natural language processing, and predictive analytics to reach new heights. For instance, convolutional neural networks (CNNs) have transformed image recognition tasks by learning hierarchical representations of images, leading to breakthroughs in fields such as healthcare, where they are used for disease diagnosis from medical images. Another significant improvement is the integration of big data analytics with traditional statistical methods. This fusion allows for the analysis of vast datasets that were previously unmanageable, providing insights that were not possible before. Tools like Apache Spark and Hadoop have made it feasible to process large-scale data efficiently, enabling real-time analytics and decision-making. Additionally, advancements in data mining techniques have improved the ability to extract valuable information from raw data, facilitating better decision-making across industries. The rise of cloud computing has also played a crucial role in enhancing data analysis capabilities. Cloud platforms offer scalable infrastructure and on-demand resources, allowing organizations to handle massive datasets without the need for extensive hardware investments. This scalability has democratized access to advanced data analytics, making it possible for smaller organizations and startups to leverage sophisticated algorithms and tools. Furthermore, the integration of artificial intelligence (AI) with data analysis has opened up new avenues for automation and optimization. AI-driven tools can automatically detect anomalies, predict trends, and optimize processes, reducing the need for manual intervention and increasing overall efficiency. For example, in finance, AI-powered algorithms are used to detect fraudulent transactions in real-time, significantly reducing the risk of financial losses. In terms of future directions, the continued development of quantum computing holds immense potential for data analysis. Quantum algorithms promise to solve complex problems exponentially faster than classical computers, which could revolutionize fields like cryptography and optimization. Moreover, the increasing focus on ethical AI and explainable AI (XAI) is expected to ensure that data analysis algorithms are not only powerful but also transparent and fair. Overall, the improvements in data analysis algorithms have been transformative, enabling deeper insights and more accurate predictions. As technology continues to evolve, we can expect even more innovative applications of these algorithms, driving further advancements in various sectors and shaping the future of data-driven decision-making.
Potential Future Applications and Innovations
As technological advancements continue to propel us forward, the potential future applications and innovations are vast and exciting. In the realm of artificial intelligence, we can expect significant strides in autonomous systems, enabling self-driving cars and drones to become ubiquitous, revolutionizing transportation and logistics. AI will also play a crucial role in healthcare, with predictive analytics and personalized medicine becoming more prevalent, leading to better patient outcomes and more efficient healthcare systems. In the field of renewable energy, innovations such as advanced solar panels and more efficient wind turbines will help transition the world away from fossil fuels, mitigating climate change. Energy storage technologies like advanced batteries and supercapacitors will also see substantial improvements, making renewable energy sources more reliable and widespread. The Internet of Things (IoT) will continue to expand, integrating smart devices into every aspect of daily life, from smart homes to smart cities. This will enhance efficiency, safety, and convenience, while also providing valuable data for urban planning and resource management. Quantum computing is another area poised for significant breakthroughs. With its ability to process complex data sets exponentially faster than current computers, quantum computing will unlock new possibilities in fields such as cryptography, materials science, and pharmaceutical research. Biotechnology is set to transform industries like agriculture and medicine. Gene editing tools like CRISPR will continue to advance, allowing for precise modifications that could eradicate genetic diseases and improve crop yields. Synthetic biology will enable the creation of new biological pathways and organisms tailored for specific applications. Virtual and augmented reality technologies will become more sophisticated, changing the way we interact with information and each other. These technologies will revolutionize education, entertainment, and even therapy, providing immersive experiences that simulate real-world environments. Finally, advancements in nanotechnology will lead to the development of new materials with unique properties, such as superconductors and nanomaterials with enhanced strength and durability. These materials will find applications in everything from electronics to construction. Overall, these future applications and innovations promise to transform various sectors of society, driving economic growth, improving quality of life, and addressing some of the world's most pressing challenges. As we continue to push the boundaries of what is possible through technological advancements, we open up new avenues for progress and innovation that will shape the future in profound ways.