What Is Peptidoglycan
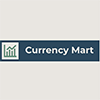
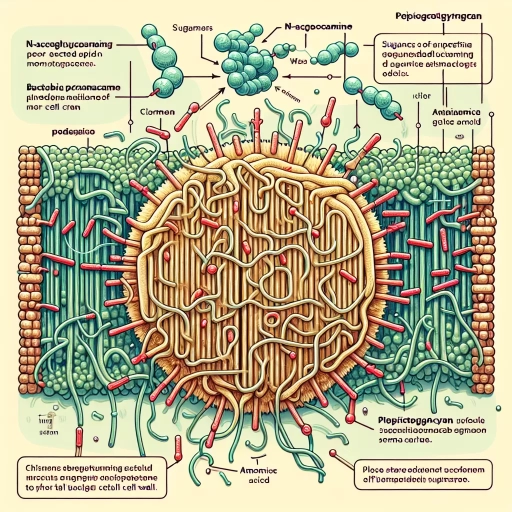
Peptidoglycan, also known as murein, is a critical component of bacterial cell walls that plays a pivotal role in the structural integrity and survival of these microorganisms. This complex polymer is not only essential for maintaining the shape and rigidity of bacterial cells but also serves as a protective barrier against external pressures and stresses. Understanding peptidoglycan involves delving into its composition, synthesis, and functional significance. In this article, we will explore what peptidoglycan is, including its molecular structure and the unique characteristics that distinguish it from other cell wall components. We will also examine how peptidoglycan is synthesized, highlighting the biochemical pathways and enzymes involved in its production. Finally, we will discuss the importance of peptidoglycan in bacterial biology, including its role in cell division, resistance to osmotic pressure, and its implications for antibiotic development. By grasping these aspects, we can appreciate the fundamental importance of peptidoglycan in bacterial life. Let us begin by exploring **What is Peptidoglycan?**
What is Peptidoglycan?
Peptidoglycan, also known as murein, is a crucial component of bacterial cell walls that plays a pivotal role in the structural integrity and survival of these microorganisms. This complex molecule is not just a passive structural element; it is dynamically involved in various biological processes that ensure the bacteria's viability. To understand the significance of peptidoglycan, it is essential to delve into its **chemical structure and composition**, which reveals the intricate arrangement of sugars and amino acids that form its backbone. Additionally, examining its **biological function in bacteria** highlights how peptidoglycan contributes to maintaining cellular shape and resisting osmotic pressure. Furthermore, exploring its **role in bacterial cell wall integrity** sheds light on how this molecule safeguards the cell from external stresses and maintains cellular homeostasis. By understanding these aspects, we can appreciate the multifaceted nature of peptidoglycan and its importance in bacterial biology. So, what is peptidoglycan?
Chemical Structure and Composition
Peptidoglycan, a critical component of bacterial cell walls, is characterized by its unique chemical structure and composition. At its core, peptidoglycan is a polymer composed of two main types of molecules: glycan chains and peptide cross-links. The glycan chains are made up of alternating units of N-acetylglucosamine (NAG) and N-acetylmuramic acid (NAM), which are linked together by β-1,4-glycosidic bonds. These chains form a robust and flexible framework that provides structural integrity to the bacterial cell wall. The peptide cross-links are attached to the NAM residues and consist of short chains of amino acids, typically including L-alanine, D-alanine, D-glutamic acid, and either L-lysine or meso-diaminopimelic acid (DAP). These peptides are covalently linked between adjacent glycan chains, creating a three-dimensional mesh that enhances the mechanical strength and stability of the peptidoglycan layer. The specific arrangement and composition of these peptide cross-links can vary among different bacterial species, influencing the overall architecture and function of the peptidoglycan. The synthesis of peptidoglycan involves a series of enzymatic reactions, including the formation of the glycan chains by glycosyltransferases and the assembly of the peptide cross-links by transpeptidases. This complex process is tightly regulated to ensure the proper assembly and maintenance of the bacterial cell wall, which is essential for maintaining cellular shape, resisting osmotic pressure, and protecting against environmental stresses. Understanding the chemical structure and composition of peptidoglycan is crucial for appreciating its role in bacterial physiology and pathogenesis. For instance, many antibiotics target the biosynthesis of peptidoglycan, such as penicillin, which inhibits transpeptidases, thereby disrupting the formation of peptide cross-links and leading to cell lysis. This highlights the significance of peptidoglycan not only as a structural component but also as a potential therapeutic target in combating bacterial infections. In summary, the intricate chemical structure and composition of peptidoglycan underpin its vital functions in bacterial biology, making it a fascinating subject for scientific study and a critical area for medical research.
Biological Function in Bacteria
In the intricate world of bacterial biology, peptidoglycan (also known as murein) plays a pivotal role, serving as the primary structural component of the bacterial cell wall. This complex polysaccharide is essential for maintaining the integrity and shape of bacterial cells, particularly in Gram-positive bacteria where it forms a thick layer, and in Gram-negative bacteria where it is present in a thinner layer. The biological function of peptidoglycan is multifaceted and critical for bacterial survival. Firstly, peptidoglycan acts as a mechanical barrier that protects the cell from osmotic pressure. Bacterial cells maintain a high internal osmotic pressure due to the concentration of solutes within the cytoplasm. Without peptidoglycan, the cell would burst under this pressure, leading to cell lysis. By forming a rigid yet flexible mesh-like structure, peptidoglycan provides the necessary strength to withstand these forces, ensuring the cell's structural integrity. Secondly, peptidoglycan is crucial for cell division and growth. During cell division, peptidoglycan synthesis is tightly regulated to ensure that the new cell wall is properly formed. This process involves the coordinated action of various enzymes, including transglycosylases and transpeptidases, which polymerize and cross-link the peptidoglycan layers. This precise regulation ensures that the cell wall is continuously remodeled as the cell grows and divides. Additionally, peptidoglycan plays a significant role in maintaining the cell's shape. Different bacteria have distinct shapes—such as rods, spheres, or spirals—which are largely determined by the organization and structure of their peptidoglycan layers. For example, in rod-shaped bacteria like *Escherichia coli*, peptidoglycan is synthesized in a way that maintains the rod shape by forming a cylindrical sacculus. Furthermore, peptidoglycan is involved in interactions with other cellular components and external factors. It can interact with other cell wall components such as lipopolysaccharides in Gram-negative bacteria and teichoic acids in Gram-positive bacteria. These interactions contribute to the overall stability and function of the cell wall. Moreover, peptidoglycan can be recognized by the host immune system, triggering an immune response that can be both protective and detrimental to the host. In summary, peptidoglycan is a vital component of bacterial cell walls, providing structural support, maintaining osmotic balance, facilitating cell division and growth, determining cell shape, and participating in interactions with other cellular and external components. Its importance underscores why peptidoglycan synthesis is a key target for antibiotics such as penicillin and ampicillin, which inhibit bacterial growth by disrupting peptidoglycan synthesis. Understanding the biological functions of peptidoglycan not only sheds light on bacterial biology but also informs strategies for combating bacterial infections.
Role in Bacterial Cell Wall Integrity
Peptidoglycan, also known as murein, plays a crucial role in maintaining bacterial cell wall integrity. This complex polysaccharide is essential for the structural stability and shape of bacterial cells, particularly in Gram-positive and Gram-negative bacteria. The peptidoglycan layer, often referred to as the bacterial cell wall, acts as a protective barrier against external pressures and osmotic forces that could otherwise cause the cell to burst. In Gram-positive bacteria, the peptidoglycan layer is thick and multilayered, accounting for up to 90% of the cell wall material. This robust structure provides significant mechanical strength, enabling these bacteria to withstand high internal turgor pressures. In contrast, Gram-negative bacteria have a thinner peptidoglycan layer but are surrounded by an outer membrane containing lipopolysaccharides, which also contributes to cell wall integrity. The peptidoglycan layer is composed of repeating units of N-acetylmuramic acid and N-acetylglucosamine linked by peptide cross-links. These cross-links are formed through a process involving enzymes such as transpeptidases and carboxypeptidases, which ensure the layer's rigidity and strength. The precise arrangement and cross-linking of these units are critical for maintaining the cell's shape and preventing lysis under osmotic stress. Moreover, peptidoglycan is not just a static component; it is dynamically remodeled throughout the bacterial life cycle. During cell division, peptidoglycan synthesis and degradation must be tightly regulated to allow for the formation of a new cell wall while preventing structural compromise. This dynamic remodeling involves a suite of enzymes including autolysins, which break down existing peptidoglycan, and synthases, which add new material. The importance of peptidoglycan in bacterial cell wall integrity is underscored by the fact that many antibiotics target its biosynthesis. Penicillin, for example, inhibits transpeptidase enzymes necessary for cross-linking peptidoglycan units, leading to weakened cell walls and eventual lysis. This highlights the critical role peptidoglycan plays in bacterial survival and the rationale behind targeting it in antimicrobial therapies. In summary, peptidoglycan is indispensable for maintaining bacterial cell wall integrity due to its structural role in providing mechanical strength and shape to the cell. Its dynamic remodeling during the bacterial life cycle ensures continuous functionality, and its importance is further emphasized by its role as a target for many antibiotics. Understanding peptidoglycan's function is crucial for appreciating bacterial physiology and developing effective antimicrobial strategies.
How is Peptidoglycan Synthesized?
Peptidoglycan, also known as murein, is a critical component of bacterial cell walls, providing structural integrity and protection against osmotic pressure. The synthesis of peptidoglycan is a complex process that involves multiple enzymes, precise biochemical steps, and stringent regulatory mechanisms. This article delves into the intricacies of peptidoglycan synthesis, exploring the enzymes involved in this process, the detailed steps of the biosynthetic pathway, and the regulatory mechanisms that ensure its accurate and efficient production. Understanding these aspects is crucial for appreciating the overall function of peptidoglycan in bacterial physiology. By examining the enzymes that catalyze each step, the sequential biochemical reactions that build the peptidoglycan layer, and the regulatory controls that govern this synthesis, we gain a comprehensive insight into how this essential polymer is constructed. This knowledge not only enhances our understanding of bacterial biology but also informs strategies for antibiotic development and bacterial pathogenesis. To fully grasp the significance of peptidoglycan synthesis, it is essential to first understand **What is Peptidoglycan?**.
Enzymes Involved in Synthesis
In the intricate process of peptidoglycan synthesis, a suite of enzymes plays a crucial role, orchestrating the assembly of this essential bacterial cell wall component. The journey begins in the cytoplasm where the first steps of peptidoglycan synthesis are catalyzed by a series of enzymes. Here, MurA and MurB enzymes are involved in the conversion of UDP-N-acetylglucosamine to UDP-N-acetylmuramic acid, a key precursor molecule. Subsequent steps involve the addition of amino acids and other moieties to this precursor, facilitated by MurC, MurD, MurE, and MurF enzymes. These enzymes ensure the correct assembly of the peptidoglycan monomer unit. Once the monomer is formed, it is transported across the cytoplasmic membrane by the enzyme MraY, which catalyzes the transfer of the monomer to a lipid carrier, bactoprenol (also known as undecaprenol). This lipid-linked intermediate is then modified by the enzyme MurG, which adds a second sugar moiety, forming the complete peptidoglycan monomer linked to the lipid carrier. The final stages of peptidoglycan synthesis occur outside the cytoplasmic membrane in the periplasm. Here, the enzyme transglycosylase (part of the bifunctional enzyme penicillin-binding proteins or PBPs) catalyzes the polymerization of the lipid-linked monomers into long glycan chains. Simultaneously, the enzyme transpeptidase (also part of PBPs) cross-links these glycan chains by forming peptide bonds between the amino acid side chains, thereby creating a robust and stable peptidoglycan layer. Additionally, other enzymes such as LytE and LytF in some bacteria, and PBP1a and PBP1b in others, contribute to the maturation and remodeling of the peptidoglycan layer. These enzymes ensure that the peptidoglycan is properly cross-linked and maintained, providing structural integrity and protection against osmotic pressure. The coordinated action of these enzymes underscores the complexity and precision required for peptidoglycan synthesis. Each enzyme's specific role highlights the biochemical sophistication involved in constructing this critical component of bacterial cell walls, which is essential for bacterial survival and integrity. Understanding these enzymatic processes not only sheds light on bacterial physiology but also informs strategies for antibiotic development, as many antibiotics target these very enzymes to inhibit bacterial growth.
Steps of the Biosynthetic Pathway
The biosynthetic pathway of peptidoglycan, a crucial component of bacterial cell walls, involves a series of intricate steps that ensure the structural integrity and survival of the bacterium. This complex process can be divided into three main stages: cytoplasmic, membrane-bound, and periplasmic. **Cytoplasmic Stage:** The journey begins in the cytoplasm where the building blocks of peptidoglycan are synthesized. Here, amino acids such as L-alanine, D-alanine, and D-glutamic acid are activated and linked to form a pentapeptide chain. This chain is then attached to a molecule called UDP-N-acetylmuramic acid (UDP-MurNAc), forming UDP-MurNAc-pentapeptide. Concurrently, UDP-N-acetylglucosamine (UDP-GlcNAc) is synthesized and will later be linked to UDP-MurNAc-pentapeptide to form the disaccharide-peptide monomer. **Membrane-Bound Stage:** The next phase occurs at the cytoplasmic membrane where the disaccharide-peptide monomer is transported across the membrane via a lipid carrier molecule called bactoprenol (or undecaprenol). This lipid carrier facilitates the translocation of the monomer from the cytoplasm to the periplasmic side of the membrane. Here, the monomer is transferred from bactoprenol to the growing peptidoglycan chain, a process catalyzed by enzymes known as transglycosylases. **Periplasmic Stage:** In the periplasm, the newly added monomers undergo cross-linking reactions mediated by transpeptidases (also known as penicillin-binding proteins). These enzymes form peptide bonds between adjacent pentapeptide chains, creating a robust and stable peptidoglycan layer. This cross-linking is critical for providing mechanical strength and maintaining the osmotic integrity of the bacterial cell wall. Throughout these stages, precise coordination and regulation are essential to ensure that peptidoglycan synthesis keeps pace with bacterial growth and division. Disruptions in this pathway, such as those caused by antibiotics like penicillin, can lead to weakened cell walls and ultimately bacterial lysis. Understanding these steps not only highlights the biochemical complexity of peptidoglycan synthesis but also underscores its importance in bacterial physiology and pathogenesis.
Regulation of Peptidoglycan Synthesis
The regulation of peptidoglycan synthesis is a complex and tightly controlled process essential for bacterial cell wall integrity and survival. Peptidoglycan, also known as murein, is a critical component of the bacterial cell wall, providing structural support and maintaining cellular shape. The synthesis of peptidoglycan involves multiple enzymatic steps, each of which must be precisely regulated to ensure proper cell wall formation and adaptation to changing environmental conditions. At the heart of peptidoglycan synthesis regulation are several key enzymes and regulatory mechanisms. The process begins with the cytoplasmic steps where amino acids are incorporated into the peptidoglycan precursor, UDP-N-acetylmuramoyl-pentapeptide. This precursor is then transported across the cytoplasmic membrane by the enzyme MraY, where it is linked to a lipid carrier, bactoprenol (also known as undecaprenol). The subsequent steps involve the assembly of the peptidoglycan layer by enzymes such as MurG, which adds N-acetylglucosamine to the precursor, and transpeptidases/transglycosylases like PBP1A and PBP1B, which polymerize and cross-link the peptidoglycan strands. Regulation of these enzymes is multifaceted. For instance, the activity of penicillin-binding proteins (PBPs), which include transpeptidases and transglycosylases, is tightly controlled. PBPs are targets of beta-lactam antibiotics like penicillin, which inhibit peptidoglycan synthesis by binding to these enzymes. Bacteria have evolved resistance mechanisms, such as producing beta-lactamases that degrade these antibiotics or modifying PBPs to reduce their affinity for the drugs. Additionally, regulatory proteins and signaling pathways play crucial roles in adjusting peptidoglycan synthesis rates according to cellular needs. For example, the two-component regulatory systems in bacteria, such as the WalKR system in Gram-positive bacteria and the PmrAB system in Gram-negative bacteria, sense changes in the cell wall and adjust gene expression accordingly. These systems can modulate the expression of genes involved in peptidoglycan synthesis and modification, ensuring that the cell wall remains robust yet flexible enough to accommodate growth and division. Furthermore, peptidoglycan synthesis is coordinated with other cellular processes such as DNA replication and cell division. The bacterial cell cycle involves checkpoints that ensure proper peptidoglycan layer formation before proceeding with cell division. This coordination is mediated by proteins like FtsZ, which forms a ring structure at the mid-cell position and recruits other proteins necessary for septum formation and peptidoglycan synthesis. In summary, the regulation of peptidoglycan synthesis is a highly orchestrated process involving enzymatic control, regulatory proteins, and signaling pathways. This intricate regulation ensures that the bacterial cell wall is dynamically maintained to support growth, division, and survival under various environmental conditions. Understanding these mechanisms not only sheds light on bacterial physiology but also provides insights into potential targets for antibacterial therapies.
Importance of Peptidoglycan in Bacterial Biology
Peptidoglycan, a crucial component of bacterial cell walls, plays a pivotal role in bacterial biology, influencing various aspects of their life cycle and interactions with their environment. This complex polysaccharide is essential for maintaining the structural integrity and shape of bacterial cells, thereby affecting their morphology and function. The importance of peptidoglycan extends beyond cell shape; it is also a key factor in the mechanisms of antibiotic resistance, as alterations in peptidoglycan synthesis can render bacteria less susceptible to certain antibiotics. Additionally, peptidoglycan is recognized by the host immune system, triggering specific responses that are critical in the pathogenesis of bacterial infections. Understanding the multifaceted roles of peptidoglycan is vital for advancing our knowledge of bacterial biology and developing effective therapeutic strategies. To delve deeper into these critical aspects, we will explore how peptidoglycan influences cell shape and morphology, contributes to antibiotic resistance mechanisms, and interacts with the immune system to facilitate pathogenesis. But first, it is essential to understand **What is Peptidoglycan?**
Cell Shape and Morphology
Cell shape and morphology are critical aspects of bacterial biology, significantly influenced by the presence and structure of peptidoglycan, a key component of the bacterial cell wall. Peptidoglycan, also known as murein, is a rigid layer that provides structural support and maintains the cell's shape against osmotic pressure. In bacteria, the shape can vary widely, ranging from spherical (cocci) to rod-shaped (bacilli) and spiral forms (spirochetes), each with specific functional implications. For instance, the rod shape of many bacteria allows for efficient movement and nutrient uptake, while the spherical shape of cocci may offer advantages in terms of surface-to-volume ratio and resistance to environmental stresses. The peptidoglycan layer is essential for maintaining these shapes. It is composed of glycan chains cross-linked by short peptides, forming a mesh-like structure that provides mechanical strength and rigidity. This layer is particularly crucial in Gram-positive bacteria, where it is thicker and more extensive, contributing to their characteristic Gram stain retention. In contrast, Gram-negative bacteria have a thinner peptidoglycan layer but are surrounded by an outer membrane containing lipopolysaccharides, which also play a role in maintaining cell integrity. The importance of peptidoglycan in cell shape is evident from its role in cell division and growth. During cell division, peptidoglycan synthesis is tightly regulated to ensure that the new cell wall is properly formed, maintaining the cell's shape and preventing lysis due to osmotic pressure. Mutations or inhibitors that disrupt peptidoglycan synthesis can lead to aberrant cell shapes and often result in cell death. Furthermore, peptidoglycan's influence on cell morphology extends to its interaction with other cellular components. For example, it interacts with membrane proteins and lipids to stabilize the cell envelope and facilitate the proper functioning of transport systems and sensory mechanisms. This intricate relationship underscores the central role of peptidoglycan in bacterial cell biology, making it a vital target for antibiotics such as penicillin and ampicillin, which inhibit peptidoglycan synthesis. In summary, the shape and morphology of bacterial cells are fundamentally determined by the presence and organization of peptidoglycan. This complex polysaccharide-peptide layer not only provides structural integrity but also plays a pivotal role in cell division, growth, and overall cellular function. Understanding the importance of peptidoglycan in maintaining cell shape and morphology is essential for appreciating its broader significance in bacterial biology and its implications for antibiotic development and microbial pathogenesis.
Antibiotic Resistance Mechanisms
Antibiotic resistance mechanisms are a critical concern in the realm of bacterial biology, particularly when considering the importance of peptidoglycan. Peptidoglycan, also known as murein, is a crucial component of the bacterial cell wall, providing structural integrity and maintaining cellular shape. However, its role extends beyond mere structural support; it is also a primary target for many antibiotics. The rise of antibiotic resistance has led to a deeper understanding of the complex mechanisms bacteria employ to evade these drugs. One key mechanism involves the alteration of peptidoglycan synthesis pathways. Bacteria can modify their peptidoglycan layers to reduce the affinity for antibiotics such as penicillins and cephalosporins, which inhibit cell wall synthesis by binding to penicillin-binding proteins (PBPs). Mutations in genes encoding PBPs can lead to the production of altered PBPs with reduced affinity for these antibiotics, allowing bacteria to continue synthesizing their cell walls despite the presence of these drugs. Another significant mechanism is the production of beta-lactamases, enzymes that degrade beta-lactam antibiotics like penicillins and cephalosporins. These enzymes can hydrolyze the beta-lactam ring of the antibiotic, rendering it ineffective against the bacterial cell wall. The widespread dissemination of beta-lactamase genes among bacterial populations has significantly compromised the efficacy of these antibiotics. In addition to these enzymatic strategies, bacteria can also employ efflux pumps to expel antibiotics from the cell. These pumps can recognize and transport a wide range of compounds, including antibiotics, out of the cell, thereby reducing their intracellular concentration to sub-lethal levels. This mechanism is particularly problematic because it can confer resistance to multiple classes of antibiotics simultaneously. Furthermore, some bacteria can alter their membrane permeability to prevent antibiotics from entering the cell. For instance, Gram-negative bacteria have an outer membrane that acts as a barrier to many antibiotics. Modifications in porin proteins or the addition of efflux pumps in this outer membrane can further restrict antibiotic entry. Lastly, horizontal gene transfer plays a pivotal role in the spread of antibiotic resistance genes among bacterial populations. Plasmids, transposons, and integrons facilitate the exchange of genetic material, allowing resistant strains to emerge rapidly and disseminate widely. Understanding these mechanisms highlights the importance of peptidoglycan in bacterial biology and underscores the need for novel therapeutic strategies that target different aspects of bacterial physiology. As antibiotic resistance continues to rise, it is imperative to develop new drugs and treatment approaches that can effectively combat resistant bacterial infections while preserving the integrity of peptidoglycan and other essential cellular components.
Immune Response and Pathogenesis
The immune response and pathogenesis are intricately linked processes that highlight the critical role of peptidoglycan in bacterial biology. When a pathogenic bacterium enters the host, it triggers a multifaceted immune response designed to eliminate the invader. Peptidoglycan, a key component of the bacterial cell wall, plays a pivotal role in this interaction. Upon recognition by pattern recognition receptors such as NOD1 and NOD2, peptidoglycan fragments activate innate immune pathways, initiating the production of pro-inflammatory cytokines and chemokines. These signaling molecules recruit immune cells like neutrophils and macrophages to the site of infection, where they attempt to phagocytose and destroy the bacteria. However, pathogenic bacteria have evolved various strategies to evade or manipulate this immune response. For instance, some bacteria can modify their peptidoglycan structure to reduce its immunogenicity or produce enzymes that degrade peptidoglycan fragments, thereby avoiding detection by the host's immune system. Additionally, certain pathogens can exploit the host's immune response for their benefit, using peptidoglycan to induce a dysregulated inflammatory response that facilitates their spread and survival within the host. The pathogenesis of bacterial infections is also influenced by the structural integrity and composition of peptidoglycan. In Gram-positive bacteria, peptidoglycan forms a thick layer that provides mechanical strength and maintains cellular shape, while in Gram-negative bacteria, it is thinner but still essential for cell wall stability. Alterations in peptidoglycan structure due to mutations or antibiotic exposure can compromise bacterial viability, making it a prime target for therapeutic interventions. Moreover, the importance of peptidoglycan extends beyond its role in immune recognition and bacterial survival. It is crucial for maintaining cellular homeostasis and regulating various cellular processes, including cell division and osmotic balance. The biosynthesis of peptidoglycan is tightly regulated and involves multiple enzymes, making it a complex but essential aspect of bacterial biology. In summary, the immune response to peptidoglycan is a critical determinant of bacterial pathogenesis. Understanding how peptidoglycan interacts with the host immune system and how bacteria manipulate this interaction can provide valuable insights into developing novel therapeutic strategies against bacterial infections. The intricate relationship between peptidoglycan, immune response, and pathogenesis underscores the significance of peptidoglycan in bacterial biology, making it a fascinating area of research with significant implications for public health.