What Does Pk Mean
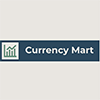
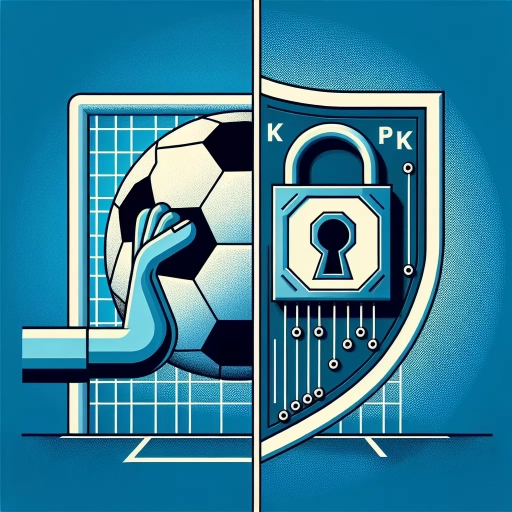
In the vast and intricate world of science, technology, and everyday life, the term "PK" holds significant importance across various domains. Whether you are delving into the scientific and technical aspects of PK, observing its applications in industry, or simply curious about its meaning, understanding this term is crucial for grasping its multifaceted implications. The acronym "PK" can refer to different concepts depending on the context, from pharmacokinetics in medical science to player killing in gaming communities. This article aims to demystify the term "PK" by exploring its core definition, delving into its scientific and technical aspects, and examining how it influences everyday life and industrial practices. By the end of this journey, you will have a comprehensive understanding of what "PK" means and how it impacts different fields. Let's begin by **Understanding the Term "PK"**, which will lay the foundation for our deeper exploration into its scientific, technical, and practical applications.
Understanding the Term "PK"
Understanding the term "PK" requires a multifaceted approach, as it encompasses various dimensions that have evolved over time. To fully grasp its significance, it is essential to delve into its historical context, explore its common uses and applications, and examine its cultural impact. Historically, the term "PK" has roots that can be traced back to specific events or movements that shaped its meaning and usage. By examining these origins, we can better understand how the term has been influenced by past events and how it has adapted over time. Additionally, "PK" is utilized in diverse contexts, from everyday conversations to specialized fields, each with its own set of applications and connotations. This versatility highlights the term's adaptability and widespread relevance. Furthermore, "PK" holds significant cultural value, reflecting societal norms, values, and beliefs that are integral to understanding its broader implications. By exploring these three aspects—historical context, common uses, and cultural significance—we can gain a comprehensive understanding of what "PK" truly represents. Let us begin by exploring the historical context of "PK," which lays the foundation for its contemporary significance.
Historical Context of PK
The term "PK" has its roots in various historical contexts, each contributing to its multifaceted meaning. In the realm of sports, particularly in soccer (or football), "PK" stands for "penalty kick," a crucial aspect of the game where a player is awarded a shot on goal from the penalty spot due to a foul committed by the opposing team within their own penalty area. This concept dates back to the late 19th century when the rules of soccer were formalized, with the penalty kick being introduced as a way to ensure fairness and deter fouls. In a different domain, "PK" is also an abbreviation for "Player Killer," a term that emerged in the early days of online gaming, particularly in massively multiplayer online role-playing games (MMORPGs). Here, it refers to a player who engages in player-versus-player combat, often targeting other players for sport or to gain in-game advantages. This usage reflects the evolving nature of gaming communities and the complexities of virtual interactions. Furthermore, "PK" can also stand for "Public Key," a fundamental concept in cryptography. Public-key cryptography, developed in the 1970s by Diffie, Hellman, and Merkle, revolutionized secure communication by allowing users to share public keys while keeping their private keys secret. This innovation has been pivotal in securing online transactions and communications, underpinning much of modern digital security. In addition, "PK" is used in various other contexts such as in business where it might refer to "Product Key," a unique code used to activate software products, or in science where it could denote "Protein Kinase," an enzyme that plays a crucial role in cellular signaling pathways. Understanding these historical contexts is essential for grasping the diverse meanings of "PK." Each usage reflects significant developments in their respective fields—sports, gaming, cryptography, and beyond—highlighting how a simple abbreviation can encapsulate complex ideas and practices that have evolved over time. By recognizing these origins, one can better navigate the nuanced landscape of modern terminology and appreciate the rich history behind such seemingly simple abbreviations.
Common Uses and Applications
Understanding the term "PK" (Player Kill) is crucial in various contexts, particularly in gaming and online communities. One of the most significant aspects of PK is its common uses and applications, which span across different domains. In the realm of online gaming, PK is a fundamental concept. It refers to the act of one player killing another player's character, often in multiplayer games such as MMORPGs (Massively Multiplayer Online Role-Playing Games) like World of Warcraft or Guild Wars. Here, PK can be a central mechanic, influencing gameplay dynamics and player interactions. For instance, in games with open-world PvP (Player versus Player) settings, PK can be a key strategy for gaining resources, territory control, or simply for the thrill of competition. Some games even have dedicated PK servers where players can engage in unrestricted combat against each other. Beyond gaming, the term PK has broader applications in social and cultural contexts. In online communities and forums, PK can metaphorically describe the act of intellectually or verbally "killing" someone's argument or point of view. This usage highlights the competitive nature of online discourse, where individuals may engage in heated debates or discussions that can be seen as a form of intellectual combat. In educational settings, particularly in fields like psychology and sociology, PK can be used as a case study to explore human behavior and social dynamics. For example, researchers might analyze how players interact in PK environments to understand aggression, cooperation, and conflict resolution strategies. This can provide valuable insights into how individuals behave in competitive environments and how these behaviors translate to real-world scenarios. From a technological standpoint, understanding PK can also inform the development of AI and machine learning algorithms. By studying how players make decisions in PK scenarios, developers can create more sophisticated AI models that mimic human behavior in competitive situations. This has implications for fields such as robotics, autonomous vehicles, and even cybersecurity, where adaptive decision-making is crucial. Moreover, the concept of PK has cultural significance. It reflects societal attitudes towards competition and conflict. In some cultures, PK is seen as a form of entertainment or a way to build community through shared experiences of competition. In others, it may be viewed more critically as promoting aggression or toxicity. Understanding these cultural nuances is essential for developing inclusive and respectful online environments. In conclusion, the term PK encompasses a wide range of applications and uses that extend far beyond its origins in gaming. From influencing gameplay mechanics to informing technological advancements and reflecting cultural attitudes, PK is a multifaceted concept that offers rich insights into human behavior, social dynamics, and technological innovation. By grasping the various dimensions of PK, we can better navigate the complexities of competitive environments both online and offline.
Cultural Significance
Understanding the term "PK" often requires a deeper dive into cultural significance, as it is a term that can vary widely in meaning depending on the context. In many cultures, particularly in South Asia, "PK" stands for "Peekay," which is a colloquial term derived from the Hindi word "peekay," meaning "vomit." However, this term gained global recognition through the 2014 Indian film "PK," directed by Rajkumar Hirani. The movie uses the character PK, played by Aamir Khan, to explore themes of religion, identity, and societal norms. Here, PK is an alien who lands on Earth and navigates human culture with a childlike curiosity, highlighting the absurdities and contradictions within religious practices. The cultural significance of "PK" extends beyond its literal meaning to symbolize a critique of societal norms and religious dogma. The film's narrative delves into issues such as blind faith, superstition, and the commercialization of religion. By portraying PK as an outsider looking in, the film challenges viewers to question their own beliefs and practices. This approach resonates deeply within Indian society, where religion plays a pivotal role in daily life. The movie's success not only in India but also internationally underscores its ability to transcend cultural boundaries and spark meaningful conversations about faith and identity. Moreover, "PK" has become a cultural phenomenon that reflects broader societal issues. It touches upon themes of tolerance, acceptance, and the importance of critical thinking. The character's innocence and naivety serve as a mirror to society, reflecting back our own biases and prejudices. This reflection encourages viewers to engage in introspection and consider the implications of their actions and beliefs. In this sense, "PK" is not just a film but a cultural artifact that prompts dialogue and fosters empathy. The term "PK" has also become synonymous with social commentary in contemporary Indian cinema. It represents a shift towards more nuanced storytelling that tackles complex issues head-on. This shift is significant because it indicates a growing appetite among audiences for films that challenge conventional wisdom and provoke thought. As such, "PK" serves as a benchmark for future films aiming to blend entertainment with social commentary. In conclusion, understanding the term "PK" involves recognizing its multifaceted nature—ranging from its literal meaning to its broader cultural implications. The film "PK" has embedded itself in popular culture as a symbol of critical inquiry and social critique. Its impact extends beyond the screen to influence public discourse on religion, identity, and societal norms. As a result, "PK" stands as a testament to the power of storytelling in shaping cultural narratives and fostering meaningful engagement with the world around us.
Scientific and Technical Aspects of PK
The scientific and technical aspects of pKa (acid dissociation constant) are fundamental to understanding various chemical and biological processes. pKa is a critical parameter that quantifies the strength of an acid in solution, influencing how it interacts with other molecules. This article delves into three key areas: the definition of pKa in chemistry and biology, the methods for measuring and calculating pKa, and its practical applications in scientific research. First, we will explore the definition of pKa in both chemical and biological contexts, highlighting its significance in acid-base reactions and its role in biological systems. This foundational understanding is crucial for grasping the subsequent sections. Next, we will discuss the various methods used to measure and calculate pKa, including experimental techniques and computational models. These methods are essential for accurately determining the pKa values of different substances. Finally, we will examine the practical applications of pKa in science, such as its use in drug design, environmental chemistry, and biochemical research. By understanding these aspects, researchers and scientists can better appreciate the importance of pKa in their work and apply this knowledge to advance their fields. Let us begin by examining the definition of pKa in chemistry and biology, which forms the basis for all subsequent discussions.
Definition in Chemistry and Biology
In the realms of chemistry and biology, the concept of "PK" is intricately linked with the fundamental principles governing the behavior of molecules and their interactions within biological systems. PK, or pharmacokinetics, refers to the study of how a drug is absorbed, distributed, metabolized, and excreted (ADME) in the body. This scientific discipline is crucial for understanding how drugs interact with biological systems at various levels. **Definition in Chemistry:** From a chemical perspective, PK involves the analysis of drug concentrations over time in different bodily compartments. It encompasses the chemical properties of the drug molecule, such as its solubility, lipophilicity, and molecular weight, which influence its absorption and distribution. For instance, a drug's ability to cross cell membranes depends on its lipophilic nature, while its solubility affects its absorption from the gastrointestinal tract. Chemical reactions such as hydrolysis, oxidation, and conjugation also play significant roles in drug metabolism, where enzymes like cytochrome P450 modify the drug molecule to make it more water-soluble for excretion. **Definition in Biology:** Biologically, PK is deeply intertwined with physiological processes. The absorption of a drug can be influenced by factors such as blood flow to the site of administration and the presence of transport proteins that facilitate or hinder drug uptake. Distribution involves the drug's interaction with plasma proteins and its ability to penetrate tissues, which is affected by factors like pH and the presence of specific receptors or transporters. Metabolism occurs primarily in the liver, where enzymes break down the drug into metabolites that may be active or inactive. Finally, excretion involves the elimination of the drug and its metabolites through renal filtration, hepatic biliary secretion, or other mechanisms. Understanding these biological processes is essential for predicting how a drug will behave in vivo and for optimizing its therapeutic efficacy and safety profile. **Scientific and Technical Aspects:** The scientific and technical aspects of PK are multifaceted. Advanced analytical techniques such as high-performance liquid chromatography (HPLC) and mass spectrometry are used to quantify drug concentrations in biological samples. Pharmacokinetic models, including compartmental models and population pharmacokinetics, help predict drug behavior based on parameters like clearance, volume of distribution, and half-life. These models are critical for dose optimization and for understanding interindividual variability in drug response. Additionally, in vitro studies using cell cultures and microsomes provide valuable insights into drug metabolism pathways, while in vivo studies in animal models and humans validate these findings. In summary, the definition of PK in chemistry and biology highlights the complex interplay between chemical properties of drugs and biological processes that govern their fate in the body. Understanding these principles is essential for developing effective therapeutic strategies and ensuring drug safety. By integrating chemical, biological, and technical aspects, pharmacokinetics provides a comprehensive framework for optimizing drug therapy and advancing our understanding of drug action at the molecular and systemic levels.
Measurement and Calculation Methods
**Measurement and Calculation Methods** In the realm of pharmacokinetics (PK), precise measurement and calculation methods are crucial for understanding how drugs are absorbed, distributed, metabolized, and excreted by the body. These processes collectively determine the drug's concentration in the bloodstream over time, which is essential for optimizing therapeutic efficacy and minimizing adverse effects. **Absorption** The absorption phase involves measuring the rate and extent to which a drug enters the systemic circulation. Techniques such as bioavailability studies and pharmacokinetic modeling help quantify this process. Bioavailability, often expressed as a percentage, indicates how much of the administered dose reaches the bloodstream in its active form. For instance, oral bioavailability can be calculated using the area under the concentration-time curve (AUC) in comparison to an intravenous dose. **Distribution** Once absorbed, drugs distribute throughout various body compartments. Volume of distribution (Vd) is a key parameter that describes this dispersion. Calculated using the formula \( Vd = \frac{Dose}{Concentration} \), it provides insight into whether a drug tends to accumulate in tissues or remain in the bloodstream. For example, lipophilic drugs often have high Vd values because they readily penetrate tissues. **Metabolism** Metabolic processes involve the breakdown of drugs by enzymes, primarily in the liver. The rate of metabolism can be quantified through parameters such as clearance (Cl) and half-life (t1/2). Clearance represents the volume of plasma cleared of drug per unit time and is calculated using the formula \( Cl = \frac{Dose}{AUC} \). Half-life, derived from clearance and volume of distribution (\( t_{1/2} = \frac{0.693 \times Vd}{Cl} \)), indicates how long it takes for the drug concentration to decrease by half. **Excretion** Excretion refers to the elimination of drugs from the body, primarily through renal excretion but also via other routes like biliary excretion. Renal clearance can be measured directly by collecting urine samples over a specified period and calculating the amount of drug excreted. This data helps in understanding how efficiently a drug is removed from the body. **Pharmacokinetic Modeling** Advanced pharmacokinetic models, such as compartmental models (e.g., one-compartment, two-compartment) and non-compartmental analysis, are employed to interpret these measurements. These models allow researchers to predict drug concentrations at various times post-administration, facilitating dose optimization and minimizing toxicity risks. **Bioanalytical Techniques** Accurate measurement of drug concentrations in biological samples is critical for PK studies. Bioanalytical techniques such as high-performance liquid chromatography (HPLC), mass spectrometry (MS), and immunoassays are commonly used. These methods ensure precise quantification of drug levels, enabling robust pharmacokinetic analysis. In summary, precise measurement and calculation methods form the backbone of pharmacokinetic studies. By accurately determining absorption rates, distribution volumes, metabolic clearances, excretion rates, and employing sophisticated modeling techniques, scientists can optimize drug dosing regimens to achieve therapeutic goals while ensuring patient safety. These methodologies are indispensable in the scientific and technical aspects of PK research.
Practical Applications in Science
**Practical Applications in Science** The term "PK" can refer to various concepts depending on the context, but in scientific and technical discourse, it often stands for "Pharmacokinetics" or "Psychokinesis." Here, we will explore practical applications in science with a focus on Pharmacokinetics. Pharmacokinetics (PK) is the study of how a drug is absorbed, distributed, metabolized, and excreted in the body. This field has numerous practical applications that are crucial for drug development and patient care. For instance, PK studies help pharmaceutical companies design drugs with optimal dosing regimens to ensure efficacy while minimizing side effects. By understanding how a drug behaves in the body, researchers can predict its therapeutic window—the range of drug concentrations that are safe and effective. This knowledge is essential for developing personalized medicine approaches, where treatments are tailored to individual patients based on their genetic profiles, age, weight, and other factors. In clinical settings, PK data guide healthcare providers in making informed decisions about drug dosing. For example, in patients with renal or hepatic impairment, PK studies provide insights into how these conditions affect drug metabolism and clearance, allowing for dose adjustments to prevent toxicity or ensure adequate therapeutic levels. Additionally, PK principles are applied in drug-drug interaction studies to predict potential adverse effects when multiple medications are administered concurrently. Beyond drug development and clinical practice, PK has significant implications for public health. Regulatory agencies such as the FDA rely heavily on PK data to approve new drugs and ensure their safety and efficacy. Moreover, PK research informs policies related to drug use in vulnerable populations like children and the elderly, where dosing requirements can be significantly different from those for adults. In the realm of toxicology, PK principles are used to assess the risk associated with environmental toxins and industrial chemicals. By understanding how these substances are absorbed, distributed, and metabolized in the body, scientists can better predict their potential harm and develop strategies for mitigation. Furthermore, advances in PK have led to the development of novel drug delivery systems aimed at improving bioavailability and reducing side effects. For example, targeted drug delivery systems use nanoparticles or other carriers to deliver drugs directly to the site of action, minimizing systemic exposure and enhancing therapeutic outcomes. In conclusion, the practical applications of Pharmacokinetics are vast and multifaceted, underpinning many aspects of modern medicine and public health. From optimizing drug dosing regimens to informing regulatory policies and developing innovative drug delivery systems, PK plays a critical role in ensuring that medications are safe, effective, and tailored to individual needs. As science continues to evolve, the importance of PK will only grow, driving advancements in healthcare and improving patient outcomes.
PK in Everyday Life and Industry
Phosphates (PK) play a multifaceted role in various aspects of everyday life and industry, impacting multiple sectors in profound ways. From enhancing the quality and shelf life of food products to serving as critical components in pharmaceuticals and medical treatments, phosphates are indispensable. In the food and beverage industry, phosphates act as preservatives, emulsifiers, and texture modifiers, ensuring that products remain fresh and appealing to consumers. Beyond this, phosphates are pivotal in the pharmaceutical and medical fields, where they are used in drug formulations and as vital nutrients in intravenous solutions. Additionally, their environmental implications cannot be overlooked, as they contribute to water pollution and affect aquatic ecosystems. Understanding these diverse applications and impacts is crucial for appreciating the significance of phosphates in modern society. This article will delve into these aspects, starting with the **Impact on Food and Beverage Industry**, where we will explore how phosphates transform the way we produce, consume, and enjoy our food.
Impact on Food and Beverage Industry
The impact of PK (Protein Kinase) on the food and beverage industry is multifaceted and significant, influencing various aspects from production to consumer health. Protein kinases, enzymes that modify proteins by phosphorylation, play crucial roles in cellular signaling pathways. In the context of food production, PKs are involved in the regulation of plant growth and development, which can be leveraged to enhance crop yields and resilience. For instance, genetic engineering techniques that modulate PK activity can help plants adapt better to environmental stresses such as drought or pests, leading to more sustainable agricultural practices. In food processing, understanding PKs can improve the quality and safety of products. Phosphorylation events are critical in the post-harvest handling of fruits and vegetables, affecting their ripening and spoilage rates. By manipulating these pathways, food manufacturers can extend shelf life and maintain nutritional value. Additionally, PKs are integral in the fermentation processes used in the production of beverages like beer and wine. Yeast strains with optimized PK activity can enhance fermentation efficiency and flavor profiles, contributing to better product consistency. From a nutritional perspective, PKs are essential for understanding how dietary components influence human health. For example, certain nutrients and phytochemicals found in foods can activate or inhibit specific PKs involved in metabolic pathways, thereby impacting conditions such as obesity, diabetes, and cardiovascular disease. This knowledge can guide the development of functional foods and dietary supplements that offer targeted health benefits. Moreover, the study of PKs has implications for food safety. Pathogenic bacteria often exploit host cell signaling pathways involving PKs to establish infections. Understanding these mechanisms can lead to the development of more effective antimicrobial strategies and safer food handling practices. Furthermore, PK inhibitors have potential applications as natural preservatives or additives that could replace synthetic chemicals currently used in food preservation. In summary, the impact of PKs on the food and beverage industry spans agricultural productivity, food processing quality, nutritional science, and safety protocols. By harnessing insights into PK function and regulation, the industry can drive innovation towards more sustainable, healthier, and safer food products for consumers worldwide. This underscores the broader significance of PKs in everyday life and industry beyond just their biological roles.
Role in Pharmaceutical and Medical Fields
In the pharmaceutical and medical fields, pharmacokinetics (PK) plays a pivotal role in ensuring the efficacy and safety of drugs. PK involves the study of how a drug is absorbed, distributed, metabolized, and excreted (ADME) within the body. This understanding is crucial for several reasons. Firstly, it helps in optimizing drug dosing regimens to achieve therapeutic levels while minimizing adverse effects. For instance, PK studies can determine the appropriate dosage and frequency of administration to maintain effective drug concentrations in the bloodstream. This is particularly important for drugs with narrow therapeutic windows, where the margin between efficacy and toxicity is small. Secondly, PK informs drug development by identifying potential issues early in the process. During preclinical and clinical trials, PK data help researchers understand how different populations (e.g., children, elderly, or those with specific health conditions) metabolize drugs differently. This information can lead to tailored dosing strategies that enhance patient outcomes and reduce side effects. For example, PK studies have shown that certain medications are metabolized more slowly in older adults due to decreased liver function and kidney clearance, necessitating lower doses to avoid toxicity. Thirdly, PK is essential for drug-drug interactions and polypharmacy management. When multiple drugs are administered together, their PK profiles can interact in complex ways, leading to either enhanced or diminished effects. Understanding these interactions allows healthcare providers to predict and mitigate potential risks. For instance, certain antibiotics can induce or inhibit liver enzymes that metabolize other drugs, thereby altering their PK profiles and necessitating dose adjustments. Furthermore, PK underpins personalized medicine by enabling the development of pharmacogenomic approaches. Genetic variations among individuals can significantly affect how they metabolize drugs. By integrating PK with genetic data, clinicians can prescribe medications that are more likely to be effective and safe for each patient. This personalized approach has been particularly beneficial in fields like oncology, where targeted therapies rely on precise PK profiles to maximize efficacy while minimizing side effects. In addition to these clinical applications, PK also supports regulatory decisions. Regulatory agencies such as the FDA rely heavily on PK data to approve new drugs and ensure their safety and efficacy. The submission of comprehensive PK studies is a critical component of the drug approval process, providing evidence that a drug is absorbed, distributed, metabolized, and excreted in a manner consistent with its intended use. Lastly, PK has implications beyond human health; it also influences veterinary medicine and environmental science. In veterinary medicine, understanding the PK of drugs in animals is vital for developing effective treatments while ensuring animal welfare. In environmental science, PK principles help in assessing the fate and transport of pharmaceuticals in ecosystems, which is crucial for mitigating their impact on aquatic life and human health through water contamination. In summary, pharmacokinetics is a cornerstone of pharmaceutical and medical research, enabling the development of safe and effective treatments. Its applications span from optimizing drug dosing regimens to informing personalized medicine and regulatory decisions. As our understanding of PK continues to evolve, it will remain an indispensable tool in improving patient outcomes and advancing healthcare practices.
Environmental Implications
The environmental implications of phosphoketenes (PK) in everyday life and industry are multifaceted and significant. Phosphoketenes, as versatile intermediates in organic synthesis, play a crucial role in the production of various chemicals, pharmaceuticals, and materials. However, their use and disposal can have profound effects on the environment. One of the primary concerns is the potential for PKs to contribute to air and water pollution. During their synthesis and processing, PKs can release volatile organic compounds (VOCs) that contribute to smog formation and ozone depletion. Additionally, if not properly managed, PKs can contaminate water bodies through industrial effluents, posing risks to aquatic life. The impact on soil quality is another critical aspect. PKs, when used in agricultural applications such as pesticides or fertilizers, can persist in soil for extended periods, altering microbial communities and affecting nutrient cycles. This can lead to long-term soil degradation and reduced fertility, compromising agricultural productivity and biodiversity. Furthermore, the production processes involving PKs often require energy-intensive steps, contributing to greenhouse gas emissions and exacerbating climate change. In terms of waste management, PKs present unique challenges. Due to their reactivity, they require specialized handling and disposal methods to prevent accidental releases or uncontrolled reactions. Improper disposal can result in hazardous waste that necessitates costly remediation efforts. On the other hand, responsible management practices such as recycling or safe incineration can mitigate these risks. Despite these challenges, there are also opportunities for PKs to contribute positively to environmental sustainability. For instance, PKs can be used in the development of more efficient and less toxic alternatives to traditional chemicals. In pharmaceutical applications, PKs can help synthesize drugs with reduced side effects and lower environmental impact during production. Moreover, research into green chemistry methods involving PKs aims to minimize waste and energy consumption while maximizing yield and selectivity. In conclusion, the environmental implications of PKs in everyday life and industry underscore the need for careful consideration and responsible management. By adopting sustainable practices, investing in green technologies, and ensuring stringent regulatory compliance, we can harness the benefits of PKs while minimizing their ecological footprint. This balanced approach not only protects our environment but also ensures the long-term viability of industries reliant on these versatile intermediates.