What Is Mach 10
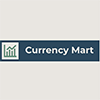
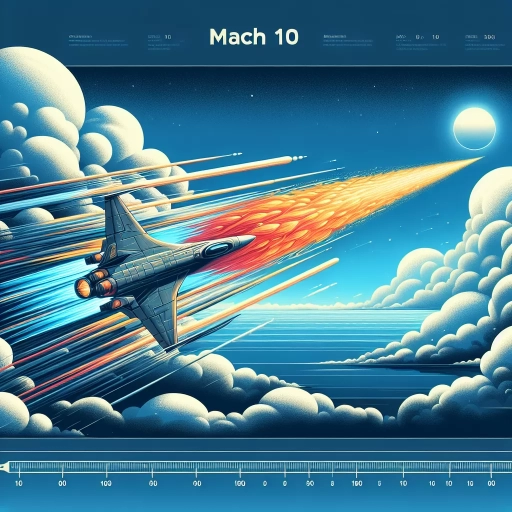
In the realm of aerospace engineering, the term "Mach 10" represents a significant milestone in speed, denoting an object traveling at ten times the speed of sound. This extraordinary velocity holds immense potential for revolutionizing various fields, from military operations to space exploration. However, achieving such speeds is fraught with technological challenges that push the boundaries of current engineering capabilities. This article delves into the intricacies of Mach 10, beginning with **Understanding Mach 10: The Basics**, where we explore the fundamental principles behind this speed and its implications. We then examine **Technological Challenges of Achieving Mach 10**, highlighting the obstacles that scientists and engineers face in designing vehicles capable of sustaining such high velocities. Finally, we discuss **Applications and Future Prospects of Mach 10 Technology**, outlining the potential uses and future developments that could transform industries and our understanding of flight. By understanding these aspects, we can better appreciate the complexity and significance of reaching Mach 10. Let's start by grasping the basics of this extraordinary speed.
Understanding Mach 10: The Basics
Understanding Mach 10, a speed that surpasses ten times the speed of sound, is a complex and fascinating topic that delves into the heart of aerodynamics and aerospace engineering. To grasp this concept fully, it is essential to explore three key areas: the definition of Mach number, the historical context and development of supersonic flight, and the importance of Mach 10 in aerospace engineering. Firstly, understanding the definition of Mach number is crucial as it provides the foundational knowledge necessary to comprehend how speeds are measured relative to the speed of sound. This metric is vital for engineers and scientists working on high-speed aircraft and spacecraft. Secondly, delving into the historical context and development of supersonic flight reveals the challenges and breakthroughs that have led to our current understanding of Mach 10. This includes the pioneering work of scientists and engineers who pushed the boundaries of speed. Lastly, recognizing the importance of Mach 10 in aerospace engineering highlights its significance in designing and operating vehicles that can withstand and achieve such extreme velocities. This knowledge is pivotal for advancing technology in fields such as military aviation, space exploration, and commercial air travel. By starting with a clear definition of Mach number, we can build a robust understanding of how this concept has evolved historically and its critical role in modern aerospace engineering. Therefore, let us begin by defining what Mach number is and how it serves as the cornerstone for understanding speeds at Mach 10.
Definition of Mach Number
The Mach number is a fundamental concept in aerodynamics and fluid dynamics, serving as a dimensionless quantity that characterizes the speed of an object relative to the speed of sound in the surrounding medium. Named after Austrian physicist Ernst Mach, this number is crucial for understanding various phenomena in aviation, aerospace engineering, and other fields where fluid flow plays a significant role. Mathematically, the Mach number (M) is defined as the ratio of the velocity of an object to the local speed of sound in the fluid it is moving through. This relationship can be expressed as \( M = \frac{v}{a} \), where \( v \) is the velocity of the object and \( a \) is the speed of sound in the medium. Understanding Mach numbers is essential because they help predict and analyze the behavior of fluids under different conditions. For instance, when an object moves at a Mach number less than 1 (subsonic), it travels slower than the speed of sound, and the flow around it is generally smooth and continuous. As the Mach number approaches 1 (transonic), the flow becomes more complex due to the formation of shock waves. At Mach 1 (sonic), the object is moving at the speed of sound, and beyond this point (supersonic), the flow becomes even more turbulent with significant shock wave formation. In the context of "Understanding Mach 10: The Basics," the definition of Mach number becomes particularly relevant. Mach 10 represents an object moving at ten times the speed of sound, which is an extremely high velocity that poses significant engineering challenges. At such speeds, air behaves more like a solid than a fluid due to the intense heat generated by friction and the severe shock waves produced. This regime is critical for hypersonic flight, where vehicles must withstand extreme temperatures and stresses while maintaining stability and control. The practical implications of Mach numbers extend beyond theoretical understanding; they are vital for designing aircraft, missiles, and spacecraft. For example, understanding how different Mach regimes affect aerodynamic forces allows engineers to optimize vehicle shapes for efficiency and stability. Additionally, knowing the Mach number helps in predicting sonic booms, which are loud noises produced when an object breaks the sound barrier. In summary, the Mach number is a critical parameter that helps scientists and engineers understand and predict fluid behavior across various speed regimes. Its definition provides a clear framework for analyzing complex aerodynamic phenomena, making it indispensable for advancing technologies in aviation and space exploration. As we delve into "Understanding Mach 10: The Basics," grasping this fundamental concept is essential for appreciating the challenges and opportunities associated with achieving such extraordinary speeds.
Historical Context and Development
The concept of Mach 10, representing a speed ten times the speed of sound, is deeply rooted in the historical context of aerodynamics and supersonic flight. The journey to understanding and achieving such high speeds began in the early 20th century, when scientists like Ernst Mach laid the groundwork for modern aerodynamics. Mach, an Austrian physicist, introduced the concept of the Mach number in the late 19th century, which quantifies the speed of an object relative to the speed of sound in the surrounding medium. This foundational work set the stage for subsequent generations of engineers and researchers who sought to push the boundaries of flight. During World War II, the need for faster aircraft drove significant advancements in aerodynamics. The development of jet engines by pioneers like Frank Whittle and Hans von Ohain enabled aircraft to break the sound barrier for the first time. Chuck Yeager's historic flight in the Bell X-1 on October 14, 1947, marked a pivotal moment when he became the first person to fly at Mach 1. This achievement opened up new avenues for research into supersonic flight. The Cold War era saw an intense focus on military aviation, leading to further innovations. The development of missiles and space exploration necessitated understanding flight at higher Mach numbers. Projects like the X-15, a rocket-powered aircraft that reached speeds up to Mach 6.72, were instrumental in expanding our knowledge of hypersonic flight. These experiments not only tested materials and designs but also provided critical data on aerodynamic behavior at extreme speeds. In the latter half of the 20th century, advancements in materials science and computational fluid dynamics allowed for more precise modeling and simulation of high-speed flight. This period also saw the introduction of scramjets (supersonic combustion ramjets), which are designed to operate efficiently at speeds above Mach 5. The NASA X-43A, for example, demonstrated the feasibility of scramjet technology by reaching speeds of approximately Mach 9.6 in 2004. Today, research into Mach 10 and beyond continues with a focus on both military applications and space exploration. Hypersonic vehicles capable of reaching such speeds are being developed for potential use in missile defense systems and as part of future space launch systems. Private companies like SpaceX and Blue Origin are also pushing the envelope with their reusable rockets, which often operate at hypersonic speeds during re-entry. Understanding Mach 10 involves not just grasping the technical aspects but also appreciating the historical context that has led us to this point. From Ernst Mach's initial theories to the current cutting-edge research, each milestone has built upon previous discoveries, driving human innovation forward in pursuit of faster and more efficient flight. As we continue to explore the frontiers of speed, we honor the legacy of those who have contributed to our understanding of aerodynamics while paving the way for future breakthroughs in this field.
Importance in Aerospace Engineering
Aerospace engineering plays a pivotal role in the advancement of modern aviation and space exploration, and its importance cannot be overstated. At the heart of this discipline lies the pursuit of pushing the boundaries of speed, efficiency, and safety. Understanding Mach 10, a speed that is ten times the speed of sound, is a prime example of this endeavor. Aerospace engineers are tasked with designing aircraft and spacecraft that can withstand the extreme conditions associated with such high velocities. This involves meticulous research into materials science to develop materials that can endure the intense heat generated by friction at Mach 10, as well as sophisticated aerodynamics to manage the complex airflow patterns that arise at these speeds. Moreover, aerospace engineering is crucial for national security, economic growth, and scientific discovery. The development of supersonic and hypersonic vehicles has significant implications for military operations, allowing for faster response times and enhanced strategic capabilities. Economically, advancements in aerospace engineering drive innovation in related industries such as manufacturing, electronics, and software development. For instance, the technological spin-offs from aerospace research have led to improvements in medical imaging, telecommunications, and even consumer electronics. From a scientific perspective, aerospace engineering is essential for expanding our understanding of the universe. Space missions rely on precise engineering to ensure that spacecraft can survive the harsh conditions of space and perform their intended functions. The exploration of Mars, for example, requires engineers to design systems that can withstand extreme temperatures, radiation, and the lack of atmosphere. These challenges drive innovation in areas such as life support systems, propulsion technologies, and communication networks. Furthermore, aerospace engineering has a profound impact on environmental sustainability. As the world grapples with climate change, engineers are working on more efficient aircraft designs that reduce fuel consumption and emissions. Electric and hybrid-electric propulsion systems are being developed to minimize the carbon footprint of air travel. Additionally, satellite technology enabled by aerospace engineering helps monitor climate changes, track weather patterns, and manage natural resources more effectively. In conclusion, the importance of aerospace engineering extends far beyond the technical aspects of building faster aircraft or reaching new altitudes. It is a field that intersects with national security, economic development, scientific discovery, and environmental sustainability. As we continue to push the boundaries of what is possible with speeds like Mach 10, aerospace engineers remain at the forefront of innovation, driving progress that benefits society as a whole. Their work not only advances our technological capabilities but also inspires future generations to pursue careers in science, technology, engineering, and mathematics (STEM).
Technological Challenges of Achieving Mach 10
Achieving Mach 10, a speed of approximately 7,673 miles per hour, is a monumental technological challenge that pushes the boundaries of current engineering capabilities. This endeavor is fraught with several critical hurdles, each requiring innovative solutions to overcome. First, aerodynamic heating and materials pose significant issues as the friction generated at such high speeds can cause severe thermal stress, necessitating the development of advanced materials that can withstand these extreme conditions. Second, propulsion systems and fuel efficiency must be optimized to generate the immense power required while maintaining a sustainable fuel consumption rate. Lastly, control and stability at high speeds are crucial, as even minor deviations can lead to catastrophic consequences due to the immense forces involved. Understanding these challenges is essential for advancing our technological prowess in hypersonic flight. By addressing these key areas, researchers and engineers can pave the way for breakthroughs in materials science, propulsion technology, and flight control systems. Let us delve into the first of these critical challenges: **Aerodynamic Heating and Materials**.
Aerodynamic Heating and Materials
Achieving Mach 10, a speed of approximately 7,600 miles per hour, poses significant technological challenges, one of the most critical being aerodynamic heating. As an object travels at such high velocities, it encounters immense friction with the atmosphere, leading to a substantial increase in surface temperature. This phenomenon, known as aerodynamic heating, can cause materials to degrade or even melt, compromising the structural integrity and performance of the vehicle. To mitigate this issue, advanced materials with high thermal resistance and durability are essential. Traditional materials like aluminum and steel are inadequate for such extreme conditions; instead, researchers turn to exotic materials such as ceramic matrix composites (CMCs) and advanced polymers. CMCs, for instance, offer exceptional thermal stability and resistance to oxidation, making them ideal for hypersonic applications. These materials are engineered to withstand temperatures exceeding 2,000 degrees Celsius, ensuring that the vehicle's structure remains intact despite the intense heat generated during flight. Another critical aspect is the development of thermal protection systems (TPS). TPS involves applying specialized coatings or tiles that can absorb or dissipate heat efficiently. For example, NASA's Space Shuttle used a combination of ceramic tiles and reinforced carbon-carbon (RCC) panels to protect its surface during re-entry into Earth's atmosphere. Similarly, for Mach 10 vehicles, innovative TPS designs are being explored, including ablative materials that gradually erode to absorb heat and maintain a stable surface temperature. In addition to material selection and TPS, aerodynamic design plays a crucial role in managing heat loads. Engineers employ computational fluid dynamics (CFD) and wind tunnel testing to optimize the vehicle's shape, minimizing areas where heat tends to concentrate. This includes designing smooth surfaces, reducing drag-inducing features, and incorporating cooling systems such as transpiration cooling or film cooling. The integration of these technologies is further complicated by the need for lightweight yet robust structures. Hypersonic vehicles must balance the requirement for high strength-to-weight ratios with the necessity for thermal protection. This has led to the development of hybrid structures that combine different materials to achieve optimal performance. For instance, sandwich structures consisting of a lightweight core material sandwiched between high-strength face sheets can provide both thermal insulation and mechanical strength. In conclusion, overcoming the challenges of aerodynamic heating is pivotal to achieving Mach 10 speeds. The development and application of advanced materials, sophisticated thermal protection systems, optimized aerodynamic designs, and innovative structural solutions are all critical components in this pursuit. As research continues to push the boundaries of what is possible in hypersonic flight, these technological advancements will be essential for ensuring the safety and efficiency of future high-speed vehicles.
Propulsion Systems and Fuel Efficiency
Achieving Mach 10, a speed of approximately 7,600 miles per hour, poses significant technological challenges, particularly in the realm of propulsion systems and fuel efficiency. Propulsion systems are the heart of any high-speed vehicle, and at such extreme velocities, conventional engines are woefully inadequate. For instance, traditional jet engines rely on air-breathing combustion, which becomes inefficient at high altitudes and speeds due to the scarcity of oxygen and the intense heat generated. To overcome this, advanced propulsion systems such as scramjets (supersonic combustion ramjets) are being developed. Scramjets use the atmosphere as the oxidizer, eliminating the need for onboard oxygen storage and significantly reducing weight. However, they require the vehicle to reach supersonic speeds before they can ignite, necessitating a booster stage. Fuel efficiency is another critical factor. At Mach 10, the energy required to accelerate and maintain such high speeds is enormous. Traditional fossil fuels lack the necessary energy density to achieve these speeds efficiently. Alternative fuels like hydrogen or advanced synthetic fuels are being explored for their higher energy density and cleaner combustion characteristics. Additionally, innovative materials and designs are crucial for managing the extreme temperatures and stresses associated with high-speed flight. Lightweight yet robust materials such as advanced composites and ceramics are essential for constructing vehicles that can withstand these conditions without compromising performance. Moreover, achieving optimal fuel efficiency involves sophisticated aerodynamic designs that minimize drag while maximizing thrust. Computational fluid dynamics (CFD) and wind tunnel testing play pivotal roles in optimizing the aerodynamic profiles of these vehicles. The integration of advanced sensors and real-time data analytics also helps in optimizing performance by adjusting parameters such as angle of attack and thrust vectoring in real-time. In summary, the technological challenges of achieving Mach 10 are multifaceted and demanding. Advanced propulsion systems like scramjets, high-energy-density fuels, cutting-edge materials, and sophisticated aerodynamic designs are all essential components in this pursuit. Overcoming these challenges will not only push the boundaries of aerospace engineering but also pave the way for future breakthroughs in high-speed flight technology.
Control and Stability at High Speeds
Achieving control and stability at high speeds, particularly at Mach 10, is a monumental technological challenge that underscores the complexities of hypersonic flight. At such extreme velocities, the dynamics of airflow and the structural integrity of the vehicle are pushed to their limits. The primary issue is managing the intense heat generated by friction with the atmosphere, which can cause materials to degrade or even vaporize. This necessitates the development of advanced materials and thermal protection systems (TPS) that can withstand temperatures exceeding 3,000 degrees Fahrenheit. Additionally, the aerodynamic forces at Mach 10 are so severe that even minor deviations in flight path can lead to catastrophic instability. To mitigate this, sophisticated control systems must be integrated, including advanced sensors, real-time data processing, and precise actuation mechanisms. These systems rely on cutting-edge algorithms and machine learning techniques to predict and correct for aerodynamic disturbances in real-time. Furthermore, the shock waves produced by hypersonic flight create unique challenges for maintaining stable airflow around the vehicle. The formation of these shock waves can lead to significant drag increases and unpredictable aerodynamic behavior, making it crucial to design vehicles with optimized shapes that minimize these effects. Computational fluid dynamics (CFD) and wind tunnel testing play critical roles in simulating and validating these designs, but even these tools have limitations when dealing with the extreme conditions of Mach 10 flight. Another critical aspect is the control of air-breathing engines, which are essential for sustained hypersonic flight. These engines must be capable of operating efficiently across a wide range of speeds and altitudes, which demands innovative solutions in combustion management and air intake design. The integration of such engines with the vehicle's control systems adds another layer of complexity, requiring seamless communication between propulsion and control subsystems to maintain stable operation. In summary, achieving control and stability at Mach 10 involves overcoming a myriad of technological hurdles related to material science, aerodynamics, control systems, and propulsion engineering. Each component must be meticulously designed and tested to ensure that the vehicle can operate safely and efficiently under conditions that are far beyond those encountered in conventional flight regimes. The pursuit of these advancements not only pushes the boundaries of current technology but also opens up new possibilities for future high-speed flight applications.
Applications and Future Prospects of Mach 10 Technology
The advent of Mach 10 technology represents a significant leap forward in the realm of high-speed flight, promising transformative impacts across various sectors. This cutting-edge innovation has the potential to revolutionize military and defense operations, enhance civilian aviation and space exploration, and pave the way for hypersonic transportation. In the military and defense arena, Mach 10 technology could enable the development of advanced missiles and aircraft capable of evading current defense systems, thereby enhancing national security. For civilian aviation and space exploration, this technology could facilitate faster travel times and more efficient access to space, opening up new frontiers for scientific research and commercial ventures. Additionally, the potential for hypersonic transportation could redefine global travel by reducing travel times between continents to mere hours. As we delve into the applications and future prospects of Mach 10 technology, it becomes clear that its military and defense applications are among the most immediate and critical, setting the stage for a new era in strategic capabilities. Therefore, we will first explore the military and defense applications of this groundbreaking technology.
Military and Defense Applications
The integration of Mach 10 technology into military and defense applications represents a significant leap forward in terms of speed, efficiency, and strategic advantage. At Mach 10, or approximately 12,000 km/h (7,500 mph), vehicles and projectiles can traverse vast distances in a fraction of the time required by conventional systems. This speed enables rapid response capabilities, allowing military forces to react swiftly to emerging threats and deploy assets with unprecedented agility. In the realm of missile defense, Mach 10 technology can revolutionize intercept systems. Hypersonic missiles, which operate at such speeds, are highly difficult to detect and intercept due to their rapid trajectory changes and minimal radar cross-sections. However, if harnessed for defensive purposes, these missiles could potentially neutralize incoming threats before they reach their targets. This capability would significantly enhance national security by providing a robust layer of protection against ballistic missiles and other high-speed threats. Moreover, Mach 10 aircraft could transform air superiority and reconnaissance missions. Hypersonic aircraft would be able to penetrate contested airspace with minimal risk of detection or interception, allowing for real-time intelligence gathering and precision strikes without exposing pilots to danger. The speed and maneuverability of these aircraft would also enable them to evade enemy defenses more effectively than current-generation fighter jets. Logistics and supply chain management within military operations could also benefit from Mach 10 technology. Rapid transportation of critical supplies over long distances could be achieved with hypersonic vehicles, ensuring that troops receive essential resources in a timely manner even in remote or hostile environments. This would enhance operational readiness and sustainment capabilities, thereby improving overall mission effectiveness. Additionally, the development of Mach 10 technology has profound implications for space exploration and satellite deployment. Hypersonic vehicles could serve as reusable launch platforms for satellites, reducing the cost and increasing the frequency of space missions. This would enable more frequent updates to satellite constellations, enhancing global communication networks, navigation systems, and Earth observation capabilities. However, the challenges associated with achieving and sustaining Mach 10 speeds are substantial. Materials must withstand extreme temperatures generated by atmospheric friction, and sophisticated guidance systems are required to maintain stability and control at such high velocities. Despite these hurdles, ongoing research and development efforts are driving innovation in materials science, aerodynamics, and propulsion systems. In conclusion, the application of Mach 10 technology in military and defense contexts offers transformative potential across various domains—from enhanced missile defense and air superiority to improved logistics and space exploration. As this technology continues to evolve, it is likely to play a pivotal role in shaping future military strategies and capabilities, providing nations with unparalleled advantages in terms of speed, agility, and operational effectiveness.
Civilian Aviation and Space Exploration
Civilian aviation and space exploration are poised to benefit significantly from the advancements in Mach 10 technology, which promises to revolutionize the speed and efficiency of flight. At Mach 10, or approximately 7,600 miles per hour, aircraft can traverse vast distances in a fraction of the time currently possible. For civilian aviation, this means that long-haul flights could be reduced to mere hours, transforming global travel and commerce. Imagine being able to fly from New York to Tokyo in under two hours, or from London to Sydney in just over three hours. Such speeds would not only enhance passenger experience but also open up new economic opportunities by facilitating faster and more efficient transportation of goods and people. In the realm of space exploration, Mach 10 technology could serve as a critical stepping stone for achieving hypersonic flight capabilities necessary for re-entry into Earth's atmosphere and for intercontinental travel. Hypersonic vehicles, capable of operating at speeds above Mach 5, are being developed to reduce the cost and complexity of accessing space. By leveraging Mach 10 technology, these vehicles could potentially achieve even higher speeds, enabling more frequent and cost-effective launches. This would be particularly beneficial for satellite deployment, space tourism, and even manned missions to the Moon and Mars. The ability to reach such high speeds would also allow for more precise control during re-entry phases, enhancing safety and reducing the risk of damage to spacecraft. Moreover, the technological innovations driven by the pursuit of Mach 10 speeds will have cascading benefits across various sectors. Advanced materials and cooling systems developed for hypersonic flight could be adapted for use in conventional aircraft, improving their efficiency and reducing fuel consumption. Additionally, the sophisticated navigation and control systems required for hypersonic flight will drive advancements in avionics and software engineering, which can then be applied to other areas of aviation and aerospace. The future prospects of Mach 10 technology are not limited to just speed; they also encompass significant environmental and societal impacts. With the potential to reduce travel times dramatically, there could be a shift away from traditional modes of transportation like ships and conventional aircraft, leading to lower carbon emissions and less environmental impact. Furthermore, the economic benefits of faster travel times could stimulate global economic growth by facilitating quicker exchange of goods, services, and ideas. However, achieving Mach 10 speeds is not without its challenges. The intense heat generated during hypersonic flight poses significant engineering hurdles that must be overcome through the development of advanced heat shields and cooling systems. Additionally, there are substantial regulatory and safety considerations that need to be addressed before such technology can be implemented commercially. Despite these challenges, the potential rewards of Mach 10 technology make it an exciting frontier in both civilian aviation and space exploration. As researchers continue to push the boundaries of what is possible with hypersonic flight, we are on the cusp of a new era in transportation that promises unprecedented speed, efficiency, and innovation. The future of travel—whether it's crossing continents or exploring the cosmos—looks brighter than ever with Mach 10 technology leading the way.
Potential for Hypersonic Transportation
The potential for hypersonic transportation, particularly at Mach 10 speeds, represents a revolutionary leap in travel technology that could transform global connectivity and commerce. Hypersonic vehicles, capable of flying at speeds over 7,600 mph (approximately 12,200 km/h), promise to significantly reduce travel times between continents. For instance, a trip from New York to London could be cut down from around 7 hours to just under 30 minutes, and journeys from Los Angeles to Tokyo could be completed in less than an hour. This drastic reduction in travel time would not only enhance personal convenience but also boost economic efficiency by facilitating faster business interactions and logistics. From a technological standpoint, achieving Mach 10 requires overcoming substantial challenges related to materials science, aerodynamics, and propulsion systems. Advanced materials must be developed to withstand the extreme heat generated during hypersonic flight, while sophisticated aerodynamic designs are needed to manage the intense friction and air resistance. Additionally, powerful propulsion systems such as scramjets (supersonic combustion ramjets) or hybrid rocket engines are essential for sustaining such high speeds. Despite these technical hurdles, several countries and private companies are actively investing in hypersonic research and development. For example, NASA and the U.S. military have been conducting extensive research on hypersonic vehicles, while private ventures like SpaceX and Blue Origin are exploring the commercial viability of such technology. These efforts are driven by the potential for hypersonic transportation to revolutionize various sectors including defense, space exploration, and civil aviation. In terms of future prospects, hypersonic transportation could open up new avenues for space tourism and satellite deployment. With the ability to reach the edge of space quickly and efficiently, launching satellites or even humans into orbit could become more accessible and cost-effective. Furthermore, the development of hypersonic technology could also lead to breakthroughs in other areas such as advanced materials and energy storage, benefiting a wide range of industries beyond transportation. However, it is crucial to address the environmental and regulatory implications of hypersonic travel. The sonic booms generated by these vehicles could pose significant noise pollution concerns, while the high energy consumption required for such speeds raises questions about sustainability. Regulatory frameworks will need to be established to ensure safe operation and mitigate potential environmental impacts. In conclusion, the potential for hypersonic transportation at Mach 10 speeds is vast and transformative. While significant technological challenges must be overcome, ongoing research and development efforts suggest that this vision is not only feasible but also nearing reality. As we move forward, it will be important to balance technological advancements with environmental responsibility and regulatory oversight to fully realize the benefits of this revolutionary technology.