What Do Astrophysicists Do
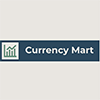
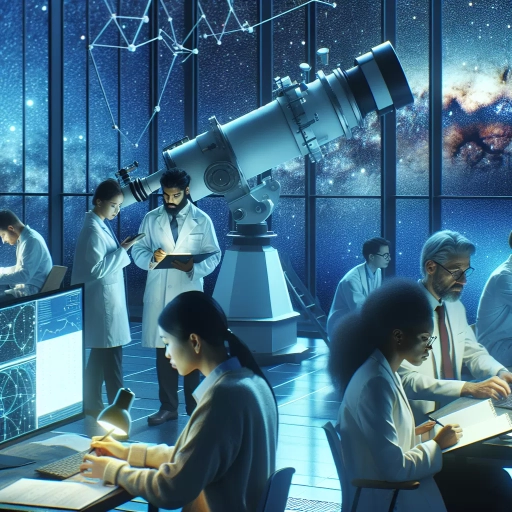
Astrophysicists are at the forefront of unraveling the mysteries of the universe, delving into the intricacies of celestial bodies, space, and time. Their work is multifaceted, involving a deep understanding of the role they play in advancing our knowledge of the cosmos. To comprehend what astrophysicists do, it is essential to explore their role in detail. This includes examining the key areas of study in astrophysics, such as stellar evolution, black holes, and cosmology. Additionally, understanding the tools and techniques they employ, from advanced telescopes to sophisticated computational models, provides insight into their methodologies. By diving into these aspects, we can gain a comprehensive view of how astrophysicists contribute to our understanding of the universe. Let's begin by understanding the role of astrophysicists and how they shape our knowledge of the cosmos.
Understanding the Role of Astrophysicists
Astrophysicists play a crucial role in advancing our understanding of the universe, leveraging a combination of observational research, theoretical modeling, and data analysis from space missions. By conducting observational research, astrophysicists gather empirical data that form the foundation of their studies. This involves using telescopes and other observational tools to collect information about celestial objects and phenomena. Additionally, developing theoretical models allows astrophysicists to interpret and predict the behavior of these objects, providing a framework for understanding complex astronomical processes. Analyzing data from space missions further enriches this knowledge by providing detailed insights into the cosmos, often revealing new phenomena and challenging existing theories. These interconnected approaches ensure that astrophysicists can draw comprehensive conclusions about the universe. To delve deeper into these processes, let's first explore the critical role of conducting observational research.
Conducting Observational Research
Conducting observational research is a cornerstone of astrophysicists' work, allowing them to gather data and insights about celestial objects and phenomena. This method involves systematically observing and recording the behavior of stars, galaxies, black holes, and other cosmic entities using a variety of tools and techniques. Astrophysicists employ advanced telescopes, both ground-based and space-based, such as the Hubble Space Telescope, to collect data across various wavelengths of the electromagnetic spectrum, including visible light, infrared, ultraviolet, X-rays, and gamma rays. These observations help scientists understand the physical properties of celestial bodies, such as their temperature, luminosity, composition, and motion. Observational research often begins with careful planning and preparation. Astrophysicists must select appropriate targets for study, design observation strategies, and allocate telescope time efficiently. Once data are collected, they undergo rigorous analysis using sophisticated software and algorithms to extract meaningful information. This process may involve calibrating instruments, correcting for atmospheric interference or other sources of noise, and applying statistical methods to ensure the reliability of the findings. The data obtained through observational research are crucial for testing theoretical models and hypotheses in astrophysics. For instance, observations of supernovae explosions have provided key evidence for the accelerating expansion of the universe, supporting the concept of dark energy. Similarly, detailed observations of exoplanet transits have allowed scientists to determine the sizes and atmospheres of planets outside our solar system. In addition to these specific examples, observational research contributes broadly to our understanding of the universe's evolution, structure, and dynamics. By studying the light curves of variable stars or the spectra of distant galaxies, astrophysicists can infer the age, composition, and evolutionary history of these objects. This cumulative knowledge helps build a comprehensive picture of the cosmos, from the formation of the first stars and galaxies to the present day. Moreover, observational research is often interdisciplinary, involving collaboration with engineers, computer scientists, and other specialists to develop new technologies and analytical techniques. The development of next-generation telescopes like the James Webb Space Telescope or the Square Kilometre Array (SKA) relies on such collaborations, enabling more precise and detailed observations than ever before. In summary, conducting observational research is essential for astrophysicists as it provides the empirical foundation upon which theoretical models are built and tested. Through meticulous observation and analysis, scientists can uncover new insights into the nature of the universe, driving forward our understanding of its mysteries and complexities. This approach underscores the critical role that astrophysicists play in advancing our knowledge of the cosmos.
Developing Theoretical Models
Developing theoretical models is a cornerstone of astrophysicists' work, enabling them to interpret and predict celestial phenomena. These models are sophisticated mathematical constructs that simulate the behavior of stars, galaxies, black holes, and other cosmic entities. Astrophysicists use a combination of physical laws, such as gravity, electromagnetism, and quantum mechanics, to build these models. For instance, they might develop stellar evolution models to understand how stars form, live, and die, or create cosmological models to explain the expansion and evolution of the universe. These theoretical frameworks are tested against observational data from telescopes and spacecraft, allowing astrophysicists to refine their models and gain deeper insights into the workings of the universe. The process involves iterative cycles of hypothesis formulation, model development, simulation, and validation against empirical evidence. By developing and refining these theoretical models, astrophysicists can make predictions about future observations and guide the design of new experiments and missions, thereby advancing our understanding of the cosmos. This interplay between theory and observation is crucial for advancing astrophysical knowledge and driving breakthroughs in our understanding of the universe.
Analyzing Data from Space Missions
Analyzing data from space missions is a critical component of the work undertaken by astrophysicists, who play a pivotal role in deciphering the vast amounts of information gathered from celestial bodies and phenomena. This process involves several key steps, each designed to extract meaningful insights from the raw data. First, astrophysicists must ensure the quality and integrity of the data, which often involves calibrating instruments and correcting for any errors or biases introduced during the collection process. Once the data is validated, they employ sophisticated algorithms and statistical methods to process and analyze it. For instance, in missions like those conducted by the Hubble Space Telescope or the Kepler Space Telescope, astrophysicists use advanced software to identify patterns, such as the light curves of distant stars or the spectral signatures of exoplanet atmospheres. The analysis phase is highly specialized and may involve collaboration with other scientists, engineers, and technicians. Astrophysicists use a variety of tools, including computational models and machine learning techniques, to interpret the data in the context of existing theories and hypotheses. For example, analyzing the cosmic microwave background radiation from missions like Planck or WMAP helps astrophysicists understand the origins and evolution of the universe. Similarly, data from missions such as Voyager 1 and 2 provide valuable insights into the outer reaches of our solar system and beyond. Interpreting this data requires a deep understanding of astrophysical principles, including gravity, electromagnetism, and nuclear physics. Astrophysicists must also consider the limitations and uncertainties of their measurements, often using Bayesian inference and other probabilistic methods to quantify these uncertainties. The findings from these analyses are then communicated through peer-reviewed publications, presentations at scientific conferences, and sometimes even public outreach programs to share the discoveries with a broader audience. Ultimately, the analysis of space mission data drives our understanding of the universe forward by providing empirical evidence that can either support or challenge existing theories. It also informs future mission design and scientific objectives, ensuring that subsequent missions are optimized to address the most pressing questions in astrophysics. By meticulously analyzing data from space missions, astrophysicists contribute significantly to our collective knowledge of the cosmos, advancing our understanding of everything from the formation of stars and galaxies to the potential for life beyond Earth. This work underscores the essential role that astrophysicists play in unraveling the mysteries of the universe.
Key Areas of Study in Astrophysics
Astrophysics, the study of celestial objects and phenomena, encompasses a broad range of key areas that collectively contribute to our understanding of the universe. At its core, astrophysics delves into three primary domains: Stellar Evolution and Formation, Galactic Structure and Dynamics, and Cosmology and the Universe's Origins. Each of these areas provides a unique lens through which we can observe and interpret the cosmos. Stellar Evolution and Formation explores the life cycles of stars, from their birth in molecular clouds to their eventual demise in supernovae or white dwarfs. Galactic Structure and Dynamics examines the organization and movement of galaxies, including our own Milky Way, shedding light on gravitational interactions and the distribution of matter. Cosmology and the Universe's Origins investigates the universe's beginnings, expansion, and evolution, often relying on theories such as the Big Bang. By understanding these interconnected fields, scientists can piece together a comprehensive narrative of the universe's history and future. This article will delve into these critical areas, starting with the intricate processes of Stellar Evolution and Formation.
Stellar Evolution and Formation
Stellar evolution and formation are cornerstone areas of study in astrophysics, providing insights into the life cycles of stars from their birth to their eventual demise. The process begins with the collapse of a molecular cloud, where gravity overcomes internal pressure, leading to the formation of a protostar. As the protostar contracts, its core heats up, eventually igniting nuclear fusion and marking the birth of a main-sequence star. Astrophysicists study this phase to understand how stars like our Sun sustain life through stable energy output. As stars age, they exhaust their hydrogen fuel, leading to expansion into red giants or more dramatic transformations for more massive stars. The red giant phase is characterized by helium fusion in the core, while the outer layers expand significantly. For stars with masses several times that of the Sun, the end stages involve explosive supernovae, leaving behind either neutron stars or black holes. These events are crucial for dispersing heavy elements throughout the galaxy, enriching subsequent star-forming regions. The study of stellar evolution also involves understanding the final stages of a star's life, including white dwarfs, neutron stars, and black holes. White dwarfs are remnants of low-mass stars that have shed their outer layers, leaving behind a hot, dense core. Neutron stars and black holes result from the collapse of more massive stars, with neutron stars being incredibly dense objects and black holes representing regions of spacetime where gravity is so strong that nothing, not even light, can escape. Astrophysicists employ a variety of methods to study stellar evolution, including spectroscopy to analyze the chemical composition and temperature of stars, photometry to measure their brightness, and asteroseismology to probe their internal structures. Computational models and simulations are also essential tools for predicting the evolutionary paths of stars under different conditions. Understanding stellar evolution is not only fundamental to astrophysics but also has broader implications for cosmology and the search for life beyond Earth. By studying how stars form and evolve, scientists can better comprehend the history of our galaxy and the potential for life-supporting planets around other stars. This knowledge is pivotal for guiding future astronomical research and missions aimed at exploring the universe in greater detail. Thus, stellar evolution and formation remain key areas of study in astrophysics, driving our understanding of the cosmos and its intricate processes.
Galactic Structure and Dynamics
Galactic structure and dynamics are pivotal areas of study in astrophysics, offering insights into the formation, evolution, and behavior of galaxies. Astrophysicists delve into the intricate details of galactic morphology, examining the distribution of stars, gas, and dark matter within these vast cosmic entities. They investigate various types of galaxies, including spiral, elliptical, and irregular galaxies, each with unique characteristics that reveal clues about their histories and interactions. The study of galactic dynamics involves understanding the gravitational interactions that govern the motion of stars, gas clouds, and other components within a galaxy. This includes analyzing rotation curves to infer the presence of dark matter, which is crucial for explaining the observed velocities of stars in the outer regions of galaxies. Astrophysicists also explore the role of supermassive black holes at the centers of many galaxies, which significantly influence the surrounding stellar and gaseous environments. Galactic structure is further elucidated through observations of galaxy clusters and superclusters, which are the largest known structures in the universe. These studies help astrophysicists understand how galaxies interact with each other and their environment on a cosmic scale. The dynamics of galaxy mergers and collisions are also a key focus, as these events can trigger starbursts, reshape galactic morphology, and redistribute matter within and between galaxies. Advanced observational techniques, such as spectroscopy and imaging with telescopes like the Hubble Space Telescope and the Atacama Large Millimeter/submillimeter Array (ALMA), provide detailed data on galactic composition and motion. Computational simulations play a complementary role, allowing astrophysicists to model complex processes like galaxy formation and evolution over billions of years. Understanding galactic structure and dynamics not only enriches our knowledge of the universe's current state but also provides a window into its past and future. By studying these phenomena, astrophysicists can reconstruct the history of galaxy formation and evolution, shedding light on fundamental questions about the cosmos, such as how galaxies came to be as we observe them today and how they will change over time. This research is integral to the broader field of astrophysics, contributing to a comprehensive understanding of the universe's structure, evolution, and ultimate fate.
Cosmology and the Universe's Origins
Cosmology, a fundamental area of study in astrophysics, delves into the origins, evolution, and fate of the universe. This field seeks to understand the universe on its largest scales, from the Big Bang to the present day. Astrophysicists studying cosmology rely on a combination of theoretical frameworks, observational data, and computational simulations to unravel the mysteries of cosmic history. The Big Bang theory, supported by evidence such as cosmic microwave background radiation and the abundance of light elements, posits that the universe began as an infinitely hot and dense point approximately 13.8 billion years ago. Since then, it has expanded and cooled, leading to the formation of subatomic particles, atoms, and eventually galaxies and stars. Key areas within cosmology include dark matter and dark energy, which are thought to make up about 95% of the universe's mass-energy budget but remain invisible to direct observation. Understanding these components is crucial for explaining the observed large-scale structure of the universe and its accelerating expansion. Additionally, cosmologists investigate the early universe through studies of primordial nucleosynthesis and the cosmic microwave background, which provide snapshots of conditions in the first fraction of a second after the Big Bang. The search for gravitational waves and the study of distant supernovae also contribute to our understanding of cosmic evolution. By integrating data from these diverse areas, astrophysicists aim to construct a comprehensive picture of how our universe came to be and what its future might hold, making cosmology an essential and captivating field within the broader discipline of astrophysics.
Tools and Techniques Used by Astrophysicists
Astrophysicists employ a diverse array of tools and techniques to unravel the mysteries of the universe. At the forefront of their research are advanced telescopes and observatories, which serve as the primary windows to the cosmos. These instruments allow scientists to observe celestial objects in various wavelengths, from visible light to gamma rays, providing invaluable data on the composition, behavior, and evolution of stars, galaxies, and other celestial entities. Additionally, computational simulations play a crucial role in astrophysics, enabling researchers to model complex phenomena such as supernovae explosions and black hole mergers. These simulations help predict outcomes and interpret observational data more accurately. Furthermore, interpreting spectroscopic data is essential for understanding the chemical and physical properties of celestial objects. By analyzing the light spectra emitted or absorbed by these objects, astrophysicists can determine their chemical composition, temperature, and motion. This multifaceted approach ensures that astrophysicists can gather comprehensive insights into the universe. To delve deeper into these methods, let's first explore how utilizing telescopes and observatories forms the foundation of modern astrophysical research.
Utilizing Telescopes and Observatories
Astrophysicists rely heavily on telescopes and observatories as fundamental tools for their research. These instruments enable scientists to study celestial objects and phenomena in unprecedented detail. Telescopes, whether optical, radio, infrared, or gamma-ray, are designed to collect and focus electromagnetic radiation from distant sources, allowing astrophysicists to gather data on the composition, temperature, and motion of stars, galaxies, and other cosmic entities. Observatories, which house these telescopes, provide controlled environments that minimize interference and optimize viewing conditions. Ground-based observatories like the Mauna Kea Observatory in Hawaii offer clear skies and stable atmospheric conditions ideal for optical and infrared observations. Space-based observatories such as the Hubble Space Telescope and the James Webb Space Telescope operate above Earth's atmosphere, providing unobstructed views of the universe in various wavelengths. By utilizing these tools, astrophysicists can conduct a wide range of studies, from monitoring the life cycles of stars to exploring the expansion of the universe itself. Advanced technologies within these observatories include adaptive optics systems that correct for atmospheric distortion and sophisticated detectors that capture faint signals from distant objects. The data collected through these instruments are then analyzed using sophisticated software and computational models to derive insights into the nature of the cosmos. Overall, telescopes and observatories are indispensable in the pursuit of understanding the universe, allowing astrophysicists to probe deeper into space and time than ever before.
Employing Computational Simulations
Employing computational simulations is a cornerstone in the toolkit of astrophysicists, enabling them to explore and understand complex celestial phenomena that are often inaccessible through direct observation. These simulations leverage advanced computational algorithms and powerful supercomputers to model various astrophysical processes, such as the formation and evolution of galaxies, the dynamics of black holes, and the behavior of stellar systems. By inputting initial conditions and physical laws into sophisticated software frameworks, astrophysicists can predict outcomes and analyze data in a controlled virtual environment. This approach allows for the testing of hypotheses, the exploration of parameter spaces, and the validation of theoretical models against observational data. For instance, simulations can recreate the conditions under which stars form and die, providing insights into the chemical enrichment of galaxies. Similarly, they can simulate the merger of neutron stars or black holes, helping to interpret the signals detected by gravitational wave observatories like LIGO and VIRGO. Computational simulations also facilitate the study of cosmic structures on large scales, such as galaxy clusters and the cosmic web, by mimicking the gravitational interactions over billions of years. Furthermore, these simulations are crucial for preparing and optimizing observational campaigns, ensuring that limited telescope time is used efficiently. By bridging the gap between theory and observation, computational simulations have become an indispensable tool for advancing our understanding of the universe, allowing astrophysicists to delve deeper into the mysteries of space and time with unprecedented precision and accuracy.
Interpreting Spectroscopic Data
Interpreting spectroscopic data is a crucial tool in the arsenal of astrophysicists, enabling them to unravel the mysteries of celestial objects and phenomena. Spectroscopy involves analyzing the interaction between matter and electromagnetic radiation, typically by measuring the spectrum of light emitted, absorbed, or scattered by an object. This technique allows astrophysicists to determine various properties of stars, galaxies, and other cosmic entities, such as their chemical composition, temperature, velocity, and distance. When interpreting spectroscopic data, astrophysicists look for specific spectral lines—distinct peaks or troughs in the spectrum—that correspond to particular wavelengths of light. These lines are characteristic of different elements and can indicate the presence of hydrogen, helium, oxygen, or other elements within a star or galaxy. By analyzing the strength and width of these lines, scientists can infer the abundance of elements, the surface temperature of stars, and even the presence of magnetic fields. The Doppler shift is another key aspect of spectroscopic analysis. When light from a celestial object is shifted towards the blue end of the spectrum (blue shift), it indicates that the object is moving towards Earth. Conversely, a shift towards the red end (red shift) suggests that the object is moving away. This principle is fundamental in understanding cosmic expansion and the dynamics of galaxies and stars. Spectroscopic data also provide insights into the physical conditions within celestial objects. For instance, the broadening of spectral lines can indicate turbulence or rotation within a star's atmosphere. Additionally, the presence of certain spectral features can reveal information about the ionization state of gases, which in turn can help scientists understand the energy sources and processes at play. Advanced spectroscopic techniques, such as high-resolution spectroscopy and multi-messenger astronomy, have further enhanced the precision and scope of astrophysical research. High-resolution spectrographs can resolve very narrow spectral lines, allowing for detailed studies of stellar atmospheres and interstellar media. Multi-messenger astronomy combines spectroscopic observations with data from other wavelengths (like gamma rays or gravitational waves) to provide a more comprehensive understanding of transient events like supernovae or black hole mergers. In summary, interpreting spectroscopic data is a powerful method that allows astrophysicists to extract rich information about the composition, motion, and physical states of celestial objects. By leveraging this technique, scientists can gain deep insights into the universe's structure, evolution, and fundamental processes, thereby advancing our understanding of the cosmos.