What Is The Fastest Thing In The World
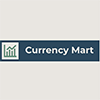
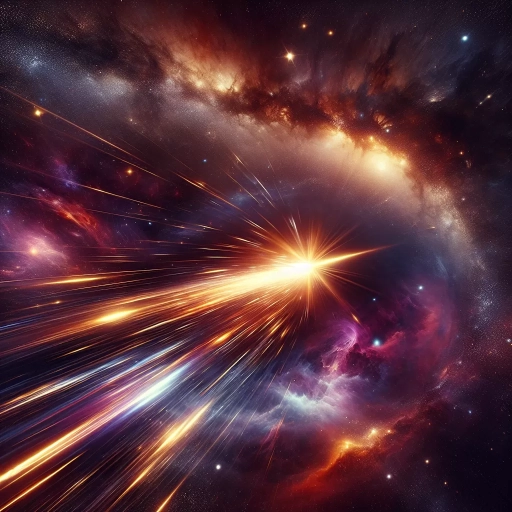
In the vast expanse of our universe, speed is a fundamental concept that fascinates scientists and laymen alike. The quest to identify the fastest thing in the world has led to intriguing discoveries across various fields. This article delves into three key areas: the unparalleled speed of light, the high-speed particles that govern the realm of physics, and the fastest man-made objects that human ingenuity has created. We begin by exploring the ultimate benchmark of speed, **The Speed of Light**, which stands as the fastest phenomenon in the universe, setting the stage for understanding other high-speed entities. This foundational concept will be followed by an examination of **High-Speed Particles in Physics**, which reveal the intricate dynamics at the subatomic level. Finally, we will look at **Fastest Man-Made Objects**, showcasing human achievements in engineering and technology. By understanding these different facets of speed, we gain a deeper appreciation for the complexities and wonders of our universe. Let us start with the most fundamental and awe-inspiring of these: **The Speed of Light**.
The Speed of Light
The speed of light is a fundamental constant in the universe, playing a pivotal role in our understanding of physics. This article delves into three key aspects that highlight its significance: the universal speed limit, its constancy in all frames of reference, and its foundational role in modern physics. The speed of light serves as a universal speed limit, dictating that no object can reach or exceed this velocity. This concept is crucial for understanding the constraints of physical motion and the behavior of particles at high energies. Additionally, the speed of light remains constant in all frames of reference, a principle that underpins Einstein's theory of special relativity. This constancy has profound implications for our understanding of space and time. Finally, the speed of light is a cornerstone of modern physics, influencing theories from quantum mechanics to cosmology. By exploring these facets, we gain a deeper appreciation for the speed of light's central role in shaping our current scientific knowledge. Let us begin by examining how the speed of light acts as a universal speed limit.
Universal Speed Limit
The concept of a universal speed limit is intricately tied to the speed of light, which is the fastest speed at which any object or information can travel in a vacuum. This speed limit, approximately 299,792 kilometers per second (186,282 miles per second), is a fundamental constant in physics and is denoted by the letter \(c\). The speed of light serves as a universal speed limit due to the constraints imposed by the theory of special relativity proposed by Albert Einstein. According to this theory, as an object approaches the speed of light, its mass increases and time appears to slow down relative to an observer at a lower speed. This makes it impossible for any object with mass to reach or exceed the speed of light because it would require an infinite amount of energy. Additionally, the speed of light is the maximum speed at which any information or matter can travel, ensuring that causality is preserved and preventing paradoxes that could arise if faster-than-light travel were possible. This universal speed limit has profound implications for our understanding of space, time, and the nature of reality itself, making it a cornerstone of modern physics and a key aspect of understanding what is indeed the fastest thing in the world.
Constant in All Frames of Reference
The concept of a constant in all frames of reference is fundamentally tied to the speed of light, which is the fastest thing in the world. According to Einstein's theory of special relativity, the speed of light (approximately 299,792 kilometers per second) remains constant and unchanging for all observers, regardless of their relative motion. This principle, known as the invariance of the speed of light, is a cornerstone of modern physics. It means that whether you are stationary or moving at high speeds relative to a light source, you will always measure the speed of light as the same. This constancy has profound implications for our understanding of space and time, as it leads to phenomena such as time dilation and length contraction. For instance, if you were to travel close to the speed of light relative to an observer on Earth, time would appear to pass more slowly for you compared to the Earth-based observer. This effect becomes more pronounced as you approach the speed of light, highlighting the unique status of light as a universal constant that transcends relative motion. The invariance of the speed of light has been experimentally verified numerous times and forms the basis for many technological advancements, including GPS technology and particle physics research. In essence, the speed of light's constancy in all frames of reference underscores its role as a fundamental limit in the universe, dictating how fast information and matter can travel and influencing our understanding of the cosmos on both microscopic and macroscopic scales.
Foundation of Modern Physics
The foundation of modern physics is rooted in the groundbreaking work of several key figures, particularly Albert Einstein, Max Planck, and Niels Bohr. These scientists revolutionized our understanding of the universe by challenging classical mechanics and introducing new concepts that form the basis of quantum mechanics and relativity. Einstein's theory of special relativity, introduced in 1905, posits that the laws of physics are the same for all observers in uniform motion relative to one another. Central to this theory is the speed of light, which Einstein showed to be a universal constant, denoted as \(c\), approximately equal to 299,792 kilometers per second. This constant speed of light is invariant, meaning it remains the same for all observers regardless of their relative motion. This concept fundamentally altered our understanding of space and time, leading to the famous equation \(E = mc^2\), which demonstrates the equivalence of mass and energy. Max Planck's work on black-body radiation laid the groundwork for quantum theory. In 1900, Planck proposed that energy is quantized, meaning it comes in discrete packets called quanta. This idea was a departure from classical physics, which treated energy as continuous. Planck's constant (\(h\)) relates the energy of a photon to its frequency, further solidifying the role of light in modern physics. Niels Bohr built upon Planck's work by developing the Bohr model of the atom, which introduced the concept of energy levels and electron spin. Bohr's model explained how electrons jump between these levels by emitting or absorbing photons, reinforcing the importance of light in atomic interactions. The speed of light, as a fundamental constant, has been a cornerstone in these developments. It has been consistently measured and confirmed across various experiments, including those involving interferometry and high-speed particle collisions. The constancy of the speed of light underpins many technological advancements, such as fiber optic communications and GPS systems, which rely on precise calculations involving this constant. In summary, the foundation of modern physics is deeply intertwined with the concept of the speed of light. Through the contributions of Einstein, Planck, and Bohr, we have come to understand that light's constant speed is a universal principle that governs both the macroscopic and microscopic worlds. This understanding has not only reshaped our theoretical framework but also driven significant technological innovations, making it clear why the speed of light remains one of the most fascinating and critical constants in physics.
High-Speed Particles in Physics
High-speed particles are a cornerstone of modern physics, offering insights into the fundamental nature of matter and energy. The study of these particles is multifaceted, involving various fields and experimental methods. One key area of research focuses on **Accelerated Particles in Particle Colliders**, where scientists use powerful machines to accelerate particles to nearly the speed of light, allowing for detailed studies of subatomic interactions. Another critical aspect is **Cosmic Rays and Their Velocities**, which involves analyzing high-energy particles originating from outside the Earth's atmosphere to understand astrophysical phenomena. Additionally, **Quantum Mechanics and Particle Speeds** delves into the theoretical framework that explains the behavior of particles at the quantum level, where speeds can be influenced by wave-particle duality and other quantum effects. By exploring these areas, physicists can gain a comprehensive understanding of the universe's most energetic and elusive entities. Transitioning to the specifics of particle acceleration, the next section will delve into the intricacies of **Accelerated Particles in Particle Colliders**.
Accelerated Particles in Particle Colliders
In the realm of high-speed particles, particle colliders play a pivotal role in accelerating particles to nearly the speed of light, enabling scientists to explore the fundamental nature of matter and energy. These sophisticated machines, such as the Large Hadron Collider (LHC) at CERN, are designed to accelerate subatomic particles like protons and electrons to incredibly high energies. The acceleration process involves powerful magnetic fields and electric fields that propel the particles around a circular or linear path. As these particles gain speed, they approach relativistic velocities, where their mass increases and time appears to slow down relative to an observer, phenomena predicted by Einstein's theory of special relativity. The LHC, for instance, accelerates protons to energies of approximately 6.5 TeV (tera-electronvolts), which is roughly 99.9999991% of the speed of light. This immense energy allows for the creation of new, heavier particles when these accelerated protons collide with each other or with stationary targets. These collisions are then analyzed by sophisticated detectors that capture the fleeting existence of these particles, providing insights into the Standard Model of particle physics and beyond. Accelerated particles in colliders also facilitate the study of quantum field theories and the search for new physics beyond the Standard Model. For example, the discovery of the Higgs boson in 2012 at the LHC was a landmark achievement that confirmed a key prediction of the Standard Model. Furthermore, ongoing and future experiments aim to explore phenomena such as dark matter and supersymmetry, which could explain some of the universe's most profound mysteries. The technology behind particle colliders is highly advanced and involves precise engineering to maintain stable beams and ensure efficient collisions. Superconducting magnets are used to steer and focus the particle beams, while sophisticated cooling systems keep these magnets at extremely low temperatures. The data generated from these collisions are analyzed using complex algorithms and computational resources, often involving international collaborations of thousands of scientists. In summary, accelerated particles in particle colliders represent one of the fastest phenomena in the universe, allowing physicists to probe the deepest secrets of matter and energy. These experiments not only validate existing theories but also open doors to new discoveries that could revolutionize our understanding of the cosmos. As technology continues to advance, future colliders promise even higher energies and more precise measurements, driving human knowledge further into the mysteries of the universe.
Cosmic Rays and Their Velocities
Cosmic rays, primarily composed of high-energy particles from outside the Earth's atmosphere, are among the fastest-moving entities in the universe. These particles, which include protons, electrons, and heavier ions, can travel at speeds approaching the speed of light. The velocities of cosmic rays are influenced by their energy; the higher the energy, the closer their speed is to the speed of light. For instance, cosmic rays with energies in the range of billions of electronvolts (GeV) can reach speeds of approximately 99.9% of the speed of light, while those with even higher energies, such as ultra-high-energy cosmic rays (UHECRs), can achieve speeds as high as 99.999% of the speed of light. This near-luminal velocity is a result of their immense kinetic energy, which propels them through interstellar and intergalactic space with minimal deceleration due to interactions with matter or radiation. The study of these high-speed particles provides valuable insights into astrophysical phenomena, such as supernovae explosions and active galactic nuclei, and contributes significantly to our understanding of the universe's most energetic processes. In the context of high-speed particles in physics, cosmic rays represent a unique class that underscores the extreme conditions and energies present in various cosmic environments, making them a fascinating subject for research and exploration.
Quantum Mechanics and Particle Speeds
Quantum Mechanics plays a pivotal role in understanding the behavior of high-speed particles, which are among the fastest phenomena in the universe. At the quantum level, particles such as electrons, photons, and other subatomic entities exhibit unique properties that defy classical physics. According to the principles of Quantum Mechanics, these particles can exist in multiple states simultaneously and exhibit wave-particle duality, meaning they can behave as both waves and particles depending on how they are observed. One of the key aspects of Quantum Mechanics relevant to high-speed particles is the concept of wave functions and probability amplitudes. These mathematical constructs help predict the likelihood of finding a particle in a particular state or location. For high-speed particles, such as those accelerated in particle colliders, understanding these probabilities is crucial for predicting collision outcomes and studying fundamental forces like the strong and weak nuclear forces. Another critical aspect is the Heisenberg Uncertainty Principle, which states that certain properties of a particle, like position and momentum, cannot be precisely known at the same time. This principle becomes particularly significant at high speeds where even small uncertainties can lead to significant variations in particle behavior. For instance, in high-energy collisions, particles can achieve speeds close to the speed of light, where relativistic effects become dominant. Relativity, especially special relativity, is integral to understanding these high-speed phenomena. As particles approach the speed of light, their mass increases and time appears to slow down relative to an observer at rest—a phenomenon known as time dilation. This effect is crucial in particle physics experiments where particles are accelerated to nearly light speed, allowing scientists to study the properties of these particles under extreme conditions. Photons, which are massless particles of light, represent the ultimate limit of speed in the universe according to Einstein's theory of special relativity. They always travel at the speed of light (approximately 299,792 kilometers per second) in a vacuum and are the fastest known entities in the universe. Other particles like electrons and protons can be accelerated to nearly this speed but never quite reach it due to their mass. In summary, Quantum Mechanics provides the theoretical framework for understanding how high-speed particles behave under various conditions. By combining principles from Quantum Mechanics with those from special relativity, physicists can accurately predict and study the behavior of these particles at speeds that are a significant fraction of the speed of light. This understanding is essential for advancing our knowledge of fundamental physics and exploring the mysteries of the universe at its most extreme scales.
Fastest Man-Made Objects
The pursuit of speed has driven human innovation to remarkable heights, culminating in the creation of some of the fastest man-made objects in history. This article delves into three key areas where speed records have been shattered: spacecraft, high-speed aircraft, and advanced rockets. Starting with **Spacecraft and Their Speed Records**, we explore how vehicles like NASA's Parker Solar Probe have achieved unprecedented velocities, allowing us to study the cosmos in ways previously unimaginable. Next, **High-Speed Aircraft and Their Capabilities** examines the technological advancements that have enabled planes like the Lockheed SR-71 Blackbird to break sound barriers and push the limits of aerodynamics. Finally, **Advanced Rockets and Propulsion Systems** discusses the cutting-edge technology driving modern rocketry, enabling faster and more efficient travel into space. By understanding these achievements, we gain insight into the engineering marvels that are redefining our understanding of speed and space exploration. Let's begin by looking at the incredible speed records set by spacecraft.
Spacecraft and Their Speed Records
Spacecraft have consistently pushed the boundaries of speed, achieving remarkable records that underscore human ingenuity and technological advancement. One of the earliest and most notable examples is the Apollo 10 mission, which in 1969 reached a speed of approximately 24,791 miles per hour (39,897 kilometers per hour) during its return from the Moon. This mission set the stage for future endeavors, demonstrating the capability to achieve high velocities necessary for interplanetary travel. The Helios 2 spacecraft, launched in 1976, holds the record for the fastest man-made object ever built, reaching an astonishing speed of about 157,000 miles per hour (253,000 kilometers per hour) as it approached the Sun. This speed was achieved due to the gravitational slingshot effect from Earth and the Sun, which significantly accelerated the spacecraft. More recently, the Parker Solar Probe, launched in 2018, has been breaking multiple speed records as it orbits the Sun. By leveraging the Sun's gravity, it has reached speeds of over 150,000 miles per hour (240,000 kilometers per hour), making it one of the fastest-moving human-made objects in space. The probe's mission to study the Sun's corona and solar wind has provided invaluable data while pushing the limits of spacecraft design and propulsion. Another notable example is the New Horizons spacecraft, which flew by Pluto in 2015 at a speed of about 36,000 miles per hour (57,900 kilometers per hour). Although not as fast as some other spacecraft, its speed was remarkable given its distance from Earth and the precision required for such a flyby. These achievements highlight not only the engineering prowess behind spacecraft design but also the scientific discoveries that such speeds enable. As technology continues to evolve, it is likely that future spacecraft will break even more speed records, further expanding our understanding of the universe and pushing the boundaries of what is thought possible.
High-Speed Aircraft and Their Capabilities
High-speed aircraft are among the fastest man-made objects, pushing the boundaries of aerodynamics and engineering. These aircraft, such as military jets and experimental vehicles, are designed to operate at speeds that exceed the sound barrier, often reaching Mach 2 or higher. For instance, the Lockheed SR-71 Blackbird, a Cold War-era spy plane, can achieve speeds up to Mach 3.5 (around 2,200 mph), making it one of the fastest operational aircraft ever built. The X-15, an experimental rocket-powered aircraft, has even reached speeds of over Mach 6 (approximately 4,500 mph), demonstrating the potential for extreme velocity in controlled environments. The capabilities of high-speed aircraft are multifaceted. They offer unparalleled reconnaissance and surveillance abilities due to their speed and altitude, allowing them to gather critical information quickly and safely. In military contexts, these aircraft can deliver precision strikes with minimal warning time for the enemy, leveraging their speed to evade defenses. Additionally, high-speed aircraft play a crucial role in advancing aerodynamic research, helping scientists understand and mitigate the effects of supersonic flight such as sonic booms and heat generated by friction. Technologically, these aircraft are equipped with advanced materials and cooling systems to withstand the intense heat generated at high speeds. They often feature sophisticated avionics and control systems to maintain stability and control during supersonic flight. The development of high-speed aircraft also drives innovation in propulsion systems, including powerful jet engines and rocket motors that are capable of generating immense thrust. Despite their impressive capabilities, high-speed aircraft face significant challenges. Operating at such extreme velocities requires precise engineering to ensure structural integrity and stability. Moreover, the environmental impact of supersonic flight, particularly the generation of sonic booms, has led to regulatory restrictions on overland flights. However, ongoing research into quieter supersonic flight technologies aims to mitigate these issues, paving the way for future commercial applications. In summary, high-speed aircraft represent the pinnacle of human engineering in terms of speed and performance. Their capabilities extend beyond military and surveillance roles to drive scientific innovation and push the limits of what is thought possible in aviation. As technology continues to evolve, these aircraft will remain at the forefront of speed and aerodynamic research, contributing significantly to our understanding of flight at the edge of human capability.
Advanced Rockets and Propulsion Systems
Advanced rockets and propulsion systems are the backbone of achieving the fastest man-made objects, pushing the boundaries of speed and efficiency in space exploration. At the forefront of this technology are liquid-fueled rockets, which offer high specific impulse—a measure of efficiency—by combining fuels like liquid hydrogen with oxidizers such as liquid oxygen. These systems, exemplified by NASA's Space Shuttle main engines and the Saturn V rocket, provide the necessary thrust to escape Earth's gravitational pull and propel spacecraft to incredible speeds. Another significant advancement is the development of solid rocket boosters, which offer simplicity and reliability. Although they have lower specific impulse compared to liquid-fueled systems, their high thrust-to-weight ratio makes them ideal for initial launch phases. The Ariane 5 and Space Shuttle solid rocket boosters are prime examples of this technology. Ion engines represent a different paradigm in propulsion, leveraging electrical energy to accelerate ions at high speeds. These engines, such as those used in NASA's Deep Space 1 and Dawn missions, achieve remarkable efficiency over long periods, making them suitable for deep space missions where continuous thrust is more valuable than high initial acceleration. Hall effect thrusters (HETs) are another type of electric propulsion system that use a magnetic field to ionize and accelerate propellant. HETs have been successfully employed in various satellites and interplanetary missions due to their high specific impulse and long operational lifetimes. Nuclear propulsion systems are also being explored for future missions. These systems can significantly enhance mission efficiency by providing higher specific impulse compared to traditional chemical rockets. Concepts like nuclear-electric propulsion and nuclear pulse propulsion are under development, promising substantial improvements in travel times to distant planets. Additionally, advanced materials and manufacturing techniques have enabled the creation of lighter yet stronger rocket components. Carbon fiber and advanced composites are increasingly used in modern rocket designs, such as SpaceX's Falcon 9 and Falcon Heavy, allowing for more efficient use of fuel and higher payload capacities. Reusability is another critical factor in modern rocket technology. Companies like SpaceX and Blue Origin have pioneered reusable launch systems, significantly reducing the cost of access to space by recovering and reusing rocket stages. This innovation not only makes space travel more affordable but also increases the frequency of launches, thereby accelerating the pace of space exploration. In summary, advanced rockets and propulsion systems are driving the creation of the fastest man-made objects through a combination of high-efficiency fuels, innovative materials, and cutting-edge technologies like ion engines and reusable launch systems. These advancements are crucial for pushing the limits of speed in space travel and enabling humanity to explore deeper into the cosmos.