What Is A Frame Structure
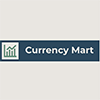
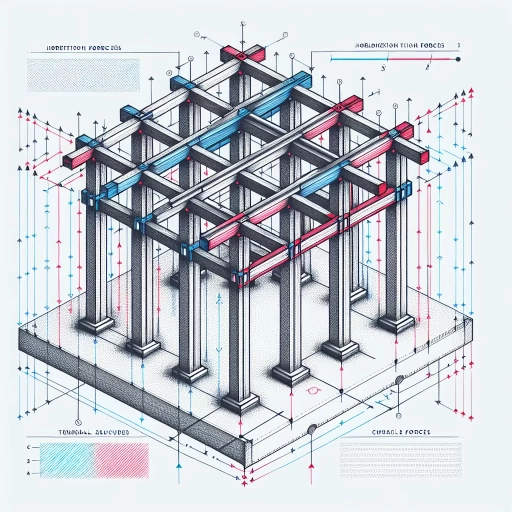
In the realm of civil engineering and architecture, frame structures stand as a cornerstone of modern construction, offering a versatile and robust framework for building design. These structures are characterized by their ability to distribute loads efficiently, providing stability and strength to a wide range of buildings, from residential homes to towering skyscrapers. This article delves into the intricacies of frame structures, beginning with an **Introduction to Frame Structures**, where we explore the fundamental principles and historical development of these architectural marvels. We then examine the **Components and Design of Frame Structures**, detailing the various elements that make up these frameworks and the engineering considerations that go into their design. Finally, we discuss the **Advantages and Challenges of Frame Structures**, highlighting their benefits in terms of flexibility, durability, and cost-effectiveness, as well as the potential drawbacks and complexities they present. By understanding these aspects, readers will gain a comprehensive insight into why frame structures remain a preferred choice in contemporary construction. Let us start by diving into the **Introduction to Frame Structures**.
Introduction to Frame Structures
Frame structures are a fundamental component of modern architecture, providing the skeletal framework that supports and shapes buildings. Understanding these structures is crucial for architects, engineers, and anyone interested in the construction industry. This article delves into the world of frame structures, offering a comprehensive introduction that covers three key aspects. First, we will explore the **Definition and Basic Principles** of frame structures, laying out the foundational concepts that govern their design and functionality. Next, we will examine the **Historical Development and Evolution** of these structures, tracing their origins and how they have adapted over time to meet changing needs and technological advancements. Finally, we will discuss **Common Applications in Architecture**, highlighting how frame structures are used in various types of buildings and their role in contemporary design. By the end of this article, you will have a solid understanding of what frame structures are, how they have evolved, and their significance in modern architecture, leading you to a deeper appreciation of the **Introduction to Frame Structures**.
Definition and Basic Principles
In the realm of structural engineering, a frame structure is a fundamental concept that underpins the design and construction of buildings, bridges, and other architectural marvels. At its core, a frame structure is defined as a three-dimensional assembly of interconnected members that provide stability and support to the entire edifice. These members, typically beams and columns, are arranged in such a way that they form a rigid framework capable of resisting various external loads such as gravity, wind, and seismic forces. The basic principles governing frame structures are rooted in the principles of mechanics and materials science. One of the primary principles is the concept of load transfer, where forces applied to the structure are distributed through the interconnected members to the foundation or other supporting elements. This distribution is crucial for ensuring that no single member bears an excessive load that could lead to failure. Another key principle is the idea of redundancy, where multiple paths for load transfer exist, enhancing the overall stability and resilience of the structure. The geometry of the frame also plays a critical role. Frames can be categorized into different types based on their configuration, such as portal frames, braced frames, and moment-resisting frames. Each type has its unique characteristics and advantages, tailored to specific applications and load conditions. For instance, braced frames utilize diagonal members to provide additional stiffness against lateral loads, while moment-resisting frames rely on the rigidity of beam-column connections to resist bending moments. Material properties are another essential consideration in frame structures. The choice of materials—whether steel, concrete, wood, or composite materials—significantly influences the structural behavior and performance. Factors such as strength, stiffness, durability, and cost must be carefully evaluated to ensure that the selected materials meet the design requirements and can withstand the anticipated loads over the structure's lifespan. Furthermore, the connections between members are vital components of frame structures. These connections must be designed to transfer forces efficiently while maintaining the integrity of the overall framework. Various connection types exist, including bolted, welded, and pinned connections, each with its own set of advantages and limitations. In summary, the definition and basic principles of frame structures revolve around the interconnected assembly of members that distribute loads effectively, redundancy for enhanced stability, geometric configurations tailored to specific needs, appropriate material selection based on performance criteria, and robust connections that ensure structural integrity. Understanding these principles is fundamental for engineers and architects aiming to design safe, efficient, and aesthetically pleasing structures that meet modern building standards.
Historical Development and Evolution
The historical development and evolution of frame structures are deeply intertwined with human ingenuity and the quest for durable, versatile, and aesthetically pleasing architectural solutions. From ancient civilizations to modern times, the concept of framing has undergone significant transformations, driven by advances in materials, technological innovations, and changing societal needs. In ancient times, early forms of frame structures were evident in the construction of wooden temples in Japan and the use of post-and-beam systems in Greek and Roman architecture. These early frames relied on simple yet effective techniques such as mortise and tenon joints, demonstrating an early understanding of structural integrity. The Middle Ages saw the rise of timber framing in Europe, particularly in the construction of churches and castles, where intricate carvings and joinery showcased craftsmanship. The Industrial Revolution marked a pivotal moment in the evolution of frame structures. The introduction of iron and steel allowed for the creation of larger, more complex frames that could support heavier loads and span greater distances. This led to the development of iconic structures like the Eiffel Tower and the first skyscrapers in Chicago, which revolutionized urban landscapes. The late 19th and early 20th centuries saw further innovations with the introduction of reinforced concrete, enabling the construction of robust and durable frames that combined strength with fire resistance. In the mid-20th century, advances in materials science and engineering led to the widespread adoption of steel framing in both residential and commercial construction. This period also saw significant improvements in prefabrication techniques, making frame structures more accessible and cost-effective. Modern frame structures incorporate a wide range of materials including aluminum, composite materials, and advanced steel alloys, each offering unique advantages in terms of strength, sustainability, and design flexibility. Today, frame structures continue to evolve with the integration of cutting-edge technologies such as 3D printing and Building Information Modeling (BIM). These tools enable architects and engineers to design and construct frames with unprecedented precision and efficiency. Additionally, there is a growing emphasis on sustainability, with many contemporary frame structures incorporating green building principles and renewable materials. Throughout their historical development, frame structures have not only reflected technological advancements but also cultural and societal values. From ancient temples to modern skyscrapers, these structures have played a crucial role in shaping urban environments and human experiences. As we move forward into an era marked by environmental challenges and technological innovation, the evolution of frame structures will continue to be a cornerstone of architectural progress.
Common Applications in Architecture
In the realm of architecture, frame structures are a cornerstone of modern building design, offering versatility and strength that cater to a wide array of applications. These structures, characterized by their skeletal framework of beams and columns, provide the necessary support for various types of buildings while allowing for open floor plans and flexible interior layouts. One of the most common applications of frame structures is in residential construction. Here, they enable the creation of spacious living areas with minimal internal walls, making them ideal for contemporary homes where open-plan living is preferred. Additionally, frame structures are widely used in commercial buildings such as office spaces and retail stores, where the need for adaptability and ease of renovation is paramount. The ability to easily reconfigure interior spaces without compromising the structural integrity of the building makes frame structures highly appealing to businesses that may need to adjust their layouts frequently. Another significant application of frame structures is in industrial settings. Warehouses, factories, and other industrial facilities benefit from the high ceilings and open spaces that frame structures provide, allowing for efficient use of space and easy movement of heavy machinery. Furthermore, frame structures are often employed in public buildings such as schools, libraries, and community centers due to their ability to support large spans without intermediate columns, creating expansive areas suitable for various community activities. In urban planning, frame structures also play a crucial role in high-rise buildings and skyscrapers, where they provide the necessary strength and stability to support multiple floors while minimizing the footprint on the ground. The use of frame structures extends beyond traditional buildings to include innovative applications such as prefabricated homes and modular construction. These methods leverage the efficiency of frame structures to produce housing units quickly and cost-effectively, addressing housing shortages and providing sustainable living solutions. Moreover, advancements in materials science have led to the development of advanced frame systems using materials like steel, aluminum, and even sustainable wood products, further expanding the possibilities for architects and engineers. In conclusion, the versatility and strength of frame structures make them an indispensable component of modern architecture. From residential homes to commercial buildings, industrial facilities, public institutions, and even cutting-edge prefabricated housing solutions, frame structures offer a robust foundation that supports a wide range of architectural needs. Their adaptability, ease of construction, and ability to accommodate various design requirements make them a preferred choice for architects seeking to create functional yet aesthetically pleasing spaces. As architectural trends continue to evolve, the role of frame structures will remain pivotal in shaping the built environment of the future.
Components and Design of Frame Structures
Frame structures are a cornerstone of modern engineering, providing the skeletal framework for buildings, bridges, and other critical infrastructure. These structures are composed of interconnected elements that work together to distribute loads efficiently and maintain structural integrity. In this article, we will delve into the components and design of frame structures, exploring three key aspects that underpin their functionality. First, we will examine the different types of frames—rigid, semi-rigid, and flexible—each with its own set of characteristics and applications. Next, we will discuss the key elements that make up these frames: beams, columns, and connections, highlighting their roles and importance in the overall structure. Finally, we will analyze how load distribution and structural integrity are achieved through careful design and engineering. By understanding these fundamental concepts, readers will gain a comprehensive insight into the intricacies of frame structures. This introduction sets the stage for a detailed exploration of these critical components, leading us seamlessly into an in-depth examination of **Introduction to Frame Structures**.
Types of Frames: Rigid, Semi-Rigid, and Flexible
In the realm of frame structures, the type of frame used can significantly impact the overall integrity, functionality, and aesthetic appeal of the building. There are three primary types of frames: rigid, semi-rigid, and flexible, each with its own set of characteristics and applications. **Rigid Frames** are characterized by their ability to resist both vertical and lateral loads without significant deformation. These frames are typically composed of beams and columns that are rigidly connected, often through welding or bolting, to form a strong and stable structure. Rigid frames are ideal for high-rise buildings, industrial facilities, and other structures that require substantial load-bearing capacity and resistance to wind and seismic forces. Their rigidity ensures minimal deflection under load, making them suitable for structures where precise alignment and stability are crucial. **Semi-Rigid Frames** strike a balance between rigidity and flexibility. Unlike rigid frames, semi-rigid frames allow for some degree of rotation at the connections between beams and columns. This flexibility can be advantageous in certain scenarios, such as in seismic zones where some degree of movement can help absorb shock. Semi-rigid frames are often used in residential and commercial buildings where the load-bearing requirements are not as extreme as those in industrial settings. They offer a compromise between structural stability and cost-effectiveness, making them a popular choice for many construction projects. **Flexible Frames**, on the other hand, are designed to accommodate significant deflection under load. These frames typically consist of pinned connections between beams and columns, allowing for free rotation at the joints. Flexible frames are commonly used in lightweight structures such as prefabricated homes, temporary buildings, and certain types of bridges. Their flexibility makes them less suitable for heavy load-bearing applications but highly adaptable to situations where ease of assembly and disassembly is important. Understanding the differences between these types of frames is essential for architects, engineers, and builders to select the most appropriate frame type for a given project. Each type has its own set of advantages and limitations, and choosing the right one can significantly impact the structural integrity, cost, and overall performance of the building. By considering factors such as load-bearing capacity, seismic resistance, and construction ease, professionals can ensure that their frame structures meet both functional and aesthetic requirements while adhering to safety standards. Ultimately, the selection of a rigid, semi-rigid, or flexible frame is a critical component in the design and construction of any frame structure.
Key Elements: Beams, Columns, and Connections
In the realm of frame structures, the key elements that underpin their stability and functionality are beams, columns, and connections. These components work in harmony to distribute loads efficiently and ensure the structural integrity of the building. **Beams** are horizontal or sloping members that resist loads from floors, roofs, and walls. They are designed to withstand bending forces, which can cause them to sag or deflect under load. The choice of beam material—such as steel, concrete, or timber—depends on the specific requirements of the structure, including strength, durability, and aesthetic considerations. For instance, steel beams offer high strength-to-weight ratios and are often used in large-scale commercial buildings, while concrete beams provide excellent resistance to fire and are commonly found in residential construction. **Columns**, on the other hand, are vertical members that support the loads from beams and transfer them to the foundation. They must resist compressive forces that could cause them to buckle or crush. The design of columns involves careful consideration of their cross-sectional area, material properties, and the presence of any eccentric loads that might induce bending. In many modern structures, composite columns combining different materials like steel and concrete are used to leverage the strengths of each material. **Connections** are the critical links between beams and columns, ensuring that the loads are transferred smoothly and that the frame behaves as a cohesive unit. These connections can be simple bolted or welded joints in steel frames or more complex detailing in concrete structures involving rebar and anchorages. The design of connections must account for various types of forces including shear, tension, and bending to prevent failure under load. Advanced techniques such as moment-resisting connections are used in seismic regions to enhance the ductility and resilience of the frame structure during earthquakes. The interplay between these elements is crucial for the overall performance of a frame structure. For example, in a moment-resisting frame designed for seismic resistance, the beams and columns are connected in such a way that they form rigid joints capable of resisting significant lateral forces. This synergy allows the structure to absorb and distribute seismic energy effectively, thereby enhancing its survivability during extreme events. In summary, beams provide horizontal support against bending forces; columns offer vertical support against compressive forces; and connections ensure seamless load transfer between these members. Together, these key elements form the backbone of frame structures, enabling them to support various loads while maintaining stability and safety. Understanding their roles and interactions is essential for engineers and architects to design robust and efficient frame structures that meet both functional and aesthetic requirements.
Load Distribution and Structural Integrity
Load distribution and structural integrity are pivotal components in the design and construction of frame structures. A frame structure, by definition, relies on a network of interconnected members to support loads and maintain stability. The distribution of loads within this framework is crucial for ensuring that no single member is subjected to excessive stress, which could compromise the entire structure's integrity. When designing a frame structure, engineers must meticulously analyze how various types of loads—such as dead loads (the weight of the structure itself), live loads (occupants, furniture, etc.), wind loads, and seismic loads—will be distributed across the frame. This involves understanding the behavior of each member under different loading conditions. For instance, beams are designed to resist bending forces, while columns are engineered to withstand compressive forces. The connections between these members, such as joints and welds, must also be robust enough to transfer loads efficiently without failing. Structural integrity is maintained through a combination of material selection, geometric design, and precise engineering calculations. Materials with high strength-to-weight ratios are often chosen to minimize the overall weight of the structure while maximizing its load-bearing capacity. The geometric configuration of the frame—whether it is a simple rectangular frame or a more complex truss system—plays a significant role in distributing loads evenly. Advanced computational tools and finite element analysis are frequently employed to simulate various loading scenarios and predict potential stress concentrations or weaknesses in the structure. Moreover, adherence to building codes and standards is essential for ensuring that frame structures meet minimum requirements for safety and performance. Regular inspections and maintenance are also critical for identifying any signs of wear or damage that could affect the structural integrity over time. In addition, innovative techniques such as prestressing and post-tensioning can be used to enhance the load-carrying capacity of certain members by introducing compressive forces that counteract tensile stresses under load. In summary, load distribution and structural integrity are interdependent aspects that must be carefully considered in the design of frame structures. By understanding how loads are distributed across the frame and ensuring that each component is capable of withstanding these forces without compromising the overall structure's stability, engineers can create robust and reliable frame structures that meet both functional and safety requirements. This meticulous approach not only ensures the longevity of the structure but also safeguards against potential failures that could have catastrophic consequences.
Advantages and Challenges of Frame Structures
Frame structures, a cornerstone of modern architecture, offer a myriad of benefits that have made them a preferred choice for builders and architects alike. One of the primary advantages of frame structures is their flexibility and adaptability in design, allowing for creative freedom and the ability to accommodate various architectural styles. Additionally, these structures are renowned for their strength, stability, and durability, ensuring that buildings can withstand the test of time and environmental stresses. However, despite these advantages, frame structures also present significant construction challenges and cost considerations that must be carefully managed. In this article, we will delve into the intricacies of frame structures, exploring their flexibility and adaptability in design, their strength, stability, and durability, as well as the construction challenges and cost considerations associated with them. By understanding these aspects, we can better appreciate the complexities and opportunities inherent in using frame structures. **Introduction to Frame Structures**.
Flexibility and Adaptability in Design
Flexibility and adaptability are crucial elements in the design of frame structures, offering a multitude of advantages that enhance their functionality and longevity. One of the primary benefits of incorporating flexibility into frame design is the ability to accommodate various loads and stresses without compromising structural integrity. This adaptability allows frame structures to respond effectively to different environmental conditions, such as seismic activity or extreme weather, by distributing forces more evenly across the structure. For instance, in regions prone to earthquakes, flexible frame designs can absorb seismic shocks, reducing the risk of catastrophic failure. Additionally, flexible frames can be easily modified or expanded as needs change over time, making them highly versatile for evolving urban landscapes or changing business requirements. However, achieving this level of flexibility and adaptability also presents several challenges. One significant challenge is ensuring that the structure maintains its stability while allowing for movement and deformation. This requires meticulous engineering and precise material selection to balance strength with flexibility. Another challenge is the potential for increased complexity in design and construction, which can lead to higher initial costs and longer project timelines. Furthermore, the integration of flexible components may necessitate advanced computational models and simulations to predict and manage stress distributions accurately. Despite these challenges, the advantages of flexibility and adaptability in frame structures far outweigh the drawbacks. For example, flexible frames can be designed with sustainable materials and practices in mind, allowing for more eco-friendly construction methods. They also offer aesthetic benefits, as they can be configured to create unique architectural forms that blend seamlessly into diverse urban environments. In commercial settings, adaptable frames enable businesses to reconfigure spaces efficiently, supporting dynamic workflows and fostering innovation. In conclusion, flexibility and adaptability are essential design considerations for frame structures, offering enhanced resilience, versatility, and sustainability. While these attributes introduce certain complexities and costs, they ultimately contribute to the creation of robust, efficient, and aesthetically pleasing structures that can meet a wide range of needs over their lifespan. By embracing these principles, architects and engineers can develop frame structures that not only stand the test of time but also adapt to the evolving demands of modern society.
Strength, Stability, and Durability
**Strength, Stability, and Durability** Frame structures are renowned for their exceptional strength, stability, and durability, making them a preferred choice in various architectural and engineering applications. The strength of a frame structure lies in its ability to distribute loads efficiently across its members. By forming a rigid framework, these structures can withstand significant external forces such as wind, seismic activity, and heavy loads without compromising their integrity. This is particularly evident in high-rise buildings and large-span structures where the frame's strength ensures the safety and functionality of the entire edifice. Stability is another critical advantage of frame structures. The interconnected nature of the frames provides excellent resistance to lateral loads, preventing the structure from collapsing or deforming under stress. This stability is crucial in regions prone to natural disasters like earthquakes or hurricanes, where the ability to maintain structural integrity is paramount. Additionally, the stability offered by frame structures allows for greater flexibility in design, enabling architects to create complex and innovative layouts that might not be feasible with other types of construction. Durability is a hallmark of well-designed frame structures. When constructed with high-quality materials and proper engineering techniques, these structures can last for decades with minimal maintenance. The use of materials like steel and reinforced concrete ensures that the frame can resist corrosion and degradation over time, maintaining its structural integrity even in harsh environmental conditions. Furthermore, the modular nature of frame structures makes them easier to repair and upgrade, extending their lifespan and reducing long-term maintenance costs. However, achieving these benefits comes with its own set of challenges. Ensuring the strength, stability, and durability of a frame structure requires meticulous planning and execution. Engineers must carefully design the frame to handle various types of loads while also considering factors such as material properties, environmental conditions, and potential hazards. Moreover, the construction process itself can be complex and labor-intensive, necessitating skilled labor and specialized equipment. Despite these challenges, the advantages of frame structures in terms of strength, stability, and durability make them an indispensable component in modern construction, offering unparalleled performance and longevity in a wide range of applications.
Construction Challenges and Cost Considerations
When it comes to the construction of frame structures, several challenges and cost considerations must be carefully navigated to ensure successful project execution. One of the primary challenges is **structural integrity**, as frame structures rely heavily on the strength and stability of their skeletal framework. Ensuring that all components are precisely engineered and assembled is crucial to prevent structural failures, which can lead to significant delays and additional costs. Another challenge is **site-specific conditions**, such as uneven terrain or extreme weather conditions, which can complicate the construction process and necessitate specialized equipment or techniques. **Material selection** also plays a critical role in frame structure construction. The choice of materials must balance durability, sustainability, and cost-effectiveness. For instance, steel frames offer high strength-to-weight ratios but may be more expensive than timber or composite alternatives. Additionally, **labor costs** can vary widely depending on the complexity of the design and the availability of skilled workers in the region. Complex designs often require specialized labor, which can drive up costs. **Regulatory compliance** is another significant consideration. Frame structures must adhere to local building codes and standards, which can vary significantly from one region to another. Non-compliance can result in costly rework or even project shutdowns. Furthermore, **sustainability** has become an increasingly important factor in modern construction. Incorporating green building practices and materials can enhance the environmental profile of a frame structure but may also increase initial costs. From a financial perspective, **budgeting** for contingencies is essential due to the inherent uncertainties in construction projects. Unexpected site issues, changes in material prices, or labor shortages can all impact the bottom line. Effective project management involves thorough risk assessment and contingency planning to mitigate these risks. In summary, while frame structures offer numerous advantages in terms of flexibility and efficiency, their construction is not without its challenges. Addressing issues related to structural integrity, site conditions, material selection, labor costs, regulatory compliance, sustainability, and budgeting is critical for ensuring that these projects are completed on time and within budget. By carefully considering these factors, builders can maximize the benefits of frame structures while minimizing potential drawbacks.