What Is The Ph Of Milk
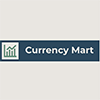
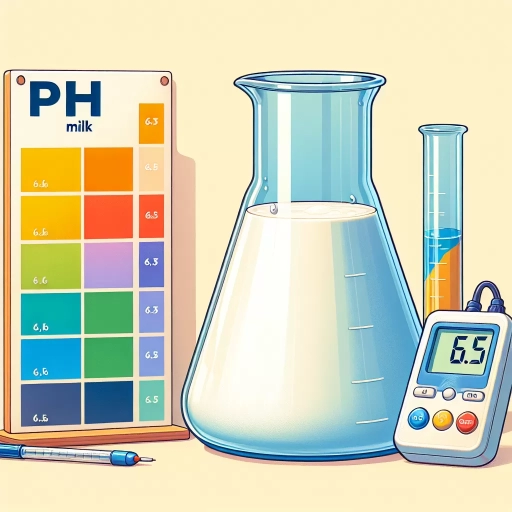
Milk, a staple in many diets around the world, is often perceived as a simple, nutritious beverage. However, its chemical composition and the factors influencing its properties are far more complex. One crucial aspect of milk that underscores its quality and safety is its pH level. The pH of milk is not just a static value; it is influenced by various factors and plays a significant role in determining the milk's overall health benefits and shelf life. To fully appreciate the significance of the pH of milk, it is essential to first understand the concept of pH and its importance in biological and chemical contexts. This understanding will provide a foundation for delving into the specific chemical composition of milk and how it affects its pH. Additionally, exploring the various factors that influence the pH of milk will offer insights into why this measure is so critical in dairy science. By examining these aspects, we can gain a comprehensive understanding of what the pH of milk truly represents and why it matters. Let's begin by understanding pH and its importance.
Understanding pH and Its Importance
Understanding pH is a fundamental concept in various scientific disciplines, including chemistry, biology, and environmental science. The importance of pH cannot be overstated, as it plays a crucial role in biological systems, affects the health of ecosystems, and is essential for numerous industrial processes. This article delves into three key aspects of pH: its definition and scale, its importance in biological systems, and the methods used to measure it. First, we will explore the definition of pH and its scale, which provides a quantitative measure of the concentration of hydrogen ions in a solution. This foundational understanding is vital for grasping how pH influences chemical reactions and biological processes. Next, we will examine the importance of pH in biological systems, highlighting how it affects enzyme activity, protein structure, and overall organism health. Finally, we will discuss the various methods for measuring pH, including pH meters, pH paper, and titration techniques, which are essential tools in both laboratory settings and everyday applications. By understanding these three facets of pH, readers will gain a comprehensive insight into why pH is such a critical parameter across different fields. Let us begin by defining pH and its scale, which serves as the cornerstone for all subsequent discussions on this topic.
Definition of pH and Its Scale
**Definition of pH and Its Scale** pH, a fundamental concept in chemistry, is a measure of the concentration of hydrogen ions in a solution, indicating its acidity or alkalinity. The term "pH" was coined by the Danish chemist Søren Sørensen in 1909, derived from the German word "Potenz," meaning power, and "H," representing hydrogen. The pH scale ranges from 0 to 14, with 7 being neutral, below 7 indicating acidic conditions, and above 7 signifying alkaline conditions. This logarithmic scale is based on the concentration of hydrogen ions (H⁺) in moles per liter; each step up or down the scale represents a tenfold change in hydrogen ion concentration. For instance, a pH of 6 is ten times more acidic than a pH of 7, and a pH of 5 is 100 times more acidic than a pH of 7. At the extremes, a pH of 0 is highly acidic (like hydrochloric acid), while a pH of 14 is highly alkaline (like sodium hydroxide). Understanding the pH scale is crucial because it influences various chemical reactions and biological processes, including enzyme activity, nutrient availability, and the stability of molecules. In the context of milk, for example, its pH is slightly acidic, typically around 6.5 to 6.7, which is important for its nutritional properties and shelf life. The pH of milk can also be an indicator of its quality and freshness, as significant deviations from this range could suggest spoilage or contamination. Thus, grasping the concept of pH and its scale is essential for comprehending the chemical and biological behaviors of substances like milk, highlighting its importance in fields such as food science, agriculture, and medicine.
Importance of pH in Biological Systems
The importance of pH in biological systems cannot be overstated, as it plays a crucial role in maintaining the delicate balance necessary for life. pH, or the measure of hydrogen ion concentration, influences virtually every biochemical reaction and process within living organisms. At the cellular level, enzymes, which are biological catalysts, function optimally within narrow pH ranges. If the pH deviates from this range, enzyme activity can be significantly impaired or even halted, leading to disruptions in metabolic pathways and potentially harmful consequences for the cell. For instance, in humans, blood pH must be tightly regulated between 7.35 and 7.45; deviations outside this range can lead to conditions such as acidosis or alkalosis, which can be life-threatening if not promptly addressed. In addition to enzyme function, pH affects protein structure and stability. Proteins, which are essential for various cellular functions including signaling, transport, and structural support, can denature or lose their native conformation if exposed to inappropriate pH levels. This denaturation can render proteins non-functional and may trigger cellular stress responses or even cell death. Furthermore, pH influences the ionization state of molecules, which is critical for membrane transport and signaling processes. For example, neurotransmitter release and synaptic transmission in the nervous system are highly pH-dependent, ensuring proper communication between neurons. Moreover, pH is vital for maintaining the integrity of cellular membranes. The fluid mosaic model of cell membranes suggests that these structures are dynamic and composed of lipids and proteins that require specific pH conditions to maintain their fluidity and function. Changes in pH can alter membrane permeability and disrupt ion gradients, which are essential for cellular homeostasis. In multicellular organisms, pH regulation is also crucial at the tissue and organ levels. For example, the stomach maintains a highly acidic environment (pH around 1.5-3.5) to activate digestive enzymes like pepsin, while the small intestine has a more alkaline environment (pH around 7-8) to facilitate nutrient absorption. Similarly, milk, with its slightly acidic pH (around 6.5-6.7), provides an optimal environment for the growth of beneficial bacteria during fermentation processes like yogurt production. In summary, the importance of pH in biological systems is multifaceted and critical for maintaining cellular homeostasis, enzyme function, protein stability, membrane integrity, and overall organismal health. Understanding and regulating pH levels are essential for preventing diseases and ensuring proper physiological function across all levels of biological organization. This underscores why pH measurement and control are fundamental aspects of both basic biological research and clinical practice.
Methods for Measuring pH
Measuring pH is a crucial aspect of understanding the chemical properties of various substances, including milk. There are several methods to determine the pH of a solution, each with its own advantages and specific applications. One of the most common and straightforward methods is using pH paper or pH strips. These strips change color in response to different pH levels, providing a quick and approximate measurement. However, for more precise measurements, pH meters are the gold standard. These electronic devices use a glass electrode to detect hydrogen ions in the solution, offering highly accurate readings. Another method involves titration, where a strong acid or base is added to the solution until it reaches a known pH, often indicated by a color change with an indicator such as phenolphthalein or methyl orange. For more complex or sensitive samples, spectroscopic methods can be employed. For instance, nuclear magnetic resonance (NMR) spectroscopy can indirectly measure pH by detecting changes in the chemical shifts of certain nuclei that are sensitive to the hydrogen ion concentration. Similarly, infrared (IR) spectroscopy can be used to measure pH by analyzing the absorption spectra of specific molecules that change with pH. In addition to these laboratory-based methods, there are also field-based techniques that allow for on-site pH measurement. Portable pH meters and handheld devices equipped with ion-selective electrodes are widely used in environmental monitoring and agricultural settings. These tools provide rapid and reliable pH readings without the need for extensive laboratory equipment. Understanding the pH of milk, specifically, is important for both dairy producers and consumers. Milk typically has a pH around 6.5 to 6.7, which is slightly acidic. This pH range is crucial for maintaining the stability and nutritional quality of milk. Deviations from this range can indicate spoilage or contamination, making accurate pH measurement essential for quality control. In summary, the choice of method for measuring pH depends on the precision required, the nature of the sample, and the context in which the measurement is being taken. From simple pH strips to sophisticated spectroscopic techniques, each method offers unique benefits and contributes to a comprehensive understanding of pH and its importance in various fields, including dairy science.
The pH of Milk: Chemical Composition
The pH of milk is a critical factor that influences its quality, shelf life, and overall nutritional profile. Understanding the chemical composition of milk and how various components affect its pH is essential for dairy producers, consumers, and researchers alike. This article delves into the intricate balance of milk's pH, exploring three key aspects: the major components of milk that affect its pH, the role of lactic acid in maintaining this balance, and the influence of other compounds on milk's acidity. By examining these elements, we can gain a comprehensive insight into how milk's chemical makeup determines its pH. Starting with the major components of milk, such as proteins, fats, and minerals, we will analyze how these constituents interact to establish the baseline pH of milk. This foundational understanding will then be expanded upon by discussing the pivotal role of lactic acid in modulating milk's pH and finally, how other compounds contribute to this delicate equilibrium. Let us begin by exploring the major components of milk that set the stage for its pH dynamics.
Major Components of Milk Affecting pH
The pH of milk is intricately influenced by several major components, each playing a crucial role in its chemical composition. At the heart of this complex interplay are the proteins, particularly casein and whey proteins. Casein, which constitutes about 80% of milk proteins, forms micelles that help maintain the stability of milk's pH. These micelles are sensitive to changes in pH and ionic strength, making them pivotal in buffering against acidic or alkaline shifts. Whey proteins, on the other hand, are more soluble and contribute to the overall protein balance that affects milk's buffering capacity. Another significant component is lactose, the primary carbohydrate in milk. Lactose can undergo fermentation by lactic acid bacteria, leading to the production of lactic acid. This process lowers the pH of milk, a phenomenon commonly observed in yogurt and cheese production. The concentration of lactose and the presence of these bacteria are critical factors in determining the final pH of fermented dairy products. Minerals such as calcium, magnesium, and phosphate ions also play a vital role in maintaining the pH balance of milk. Calcium phosphate is particularly important as it forms complexes with casein micelles, influencing their stability and thus affecting the overall pH. Changes in mineral concentrations can alter the buffering capacity of milk, making it more susceptible to pH fluctuations. Additionally, the fat content of milk has an indirect impact on its pH. While fat itself does not directly influence pH, it can affect the distribution and behavior of other components. For instance, fat globules can interact with proteins and other molecules, potentially altering their availability for reactions that might influence pH. Lastly, the presence of various enzymes and other minor components like citrate and bicarbonate ions contributes to the complex pH dynamics of milk. Enzymes such as lactoperoxidase and alkaline phosphatase can catalyze reactions that produce or consume hydrogen ions, thereby affecting the pH. Citrate and bicarbonate ions act as natural buffers, helping to stabilize the pH against external changes. In summary, the pH of milk is a delicate balance maintained by a synergy of proteins, lactose, minerals, fat content, enzymes, and other minor components. Understanding these interactions is crucial for managing dairy products' quality and shelf life, as well as for optimizing processes in dairy manufacturing. The intricate interplay among these components underscores the complexity and importance of pH regulation in milk chemistry.
Role of Lactic Acid in Milk's pH
The pH of milk is intricately linked to its chemical composition, and one of the key players in this dynamic is lactic acid. Lactic acid, a naturally occurring compound, plays a pivotal role in maintaining the pH balance of milk. When milk is produced, it has a slightly alkaline pH, typically ranging from 6.5 to 6.7. However, this pH can fluctuate due to various factors, including bacterial activity and storage conditions. Lactic acid is produced through the fermentation process initiated by lactic acid bacteria such as Lactococcus lactis and Lactobacillus acidophilus. These bacteria consume lactose (milk sugar) and convert it into lactic acid, which significantly lowers the pH of milk. This decrease in pH is crucial for several reasons. Firstly, it acts as a natural preservative by creating an environment that inhibits the growth of many pathogenic bacteria. The acidic environment makes it difficult for harmful bacteria to thrive, thereby extending the shelf life of milk. Moreover, the production of lactic acid influences the texture and consistency of milk products. For instance, in cheese production, lactic acid helps in coagulating casein proteins, leading to the formation of curds. This process is essential for creating different types of cheeses with unique textures and flavors. Similarly, in yogurt production, lactic acid contributes to the characteristic tanginess and thick consistency. In addition to its preservative and textural roles, lactic acid also impacts the nutritional profile of milk. The fermentation process enhances the bioavailability of certain nutrients like calcium and phosphorus by breaking down some of the complex molecules into more easily absorbed forms. This makes fermented dairy products such as yogurt and cheese more nutritious compared to their non-fermented counterparts. The role of lactic acid in maintaining milk's pH is also relevant from a health perspective. The slightly acidic pH helps in preserving the integrity of milk's nutritional content by preventing spoilage and ensuring that essential nutrients remain available for consumption. Furthermore, lactic acid has been linked to various health benefits, including improved gut health due to its prebiotic properties. In summary, lactic acid is a critical component in the chemical composition of milk, influencing its pH and thereby affecting its preservation, texture, nutritional value, and overall quality. The balance of lactic acid levels is essential for maintaining the optimal pH of milk, which in turn ensures that milk remains safe for consumption and retains its nutritional benefits. Understanding the role of lactic acid in milk's pH provides valuable insights into the complex interplay of chemical processes that underpin the quality and utility of dairy products.
Influence of Other Compounds on Milk's pH
The pH of milk, typically ranging from 6.5 to 6.7, is influenced by a variety of compounds present within its complex chemical composition. One of the primary factors affecting milk's pH is the concentration of lactic acid, which is produced by the natural fermentation process involving lactic acid bacteria. These bacteria convert lactose (milk sugar) into lactic acid, thereby lowering the pH and contributing to the acidic nature of milk. However, this process is not the sole determinant; other compounds such as casein, whey proteins, and various minerals also play significant roles. Casein, a major protein in milk, forms micelles that help maintain the stability of milk's pH. These micelles are composed of casein molecules and calcium phosphate, which buffer against significant pH changes by releasing or absorbing hydrogen ions as needed. This buffering capacity ensures that milk's pH remains relatively stable despite minor fluctuations in its environment. Whey proteins, on the other hand, contribute to the overall ionic balance of milk but have a lesser impact on its pH compared to casein. However, they can influence the pH indirectly by interacting with other components and affecting their availability for reactions that might alter the pH. Minerals such as calcium, magnesium, and potassium are also crucial in maintaining milk's pH. Calcium ions, in particular, are integral to the casein micelle structure and help regulate the solubility of calcium phosphate, which is essential for buffering against pH changes. The presence of these minerals can affect the activity of enzymes and the stability of proteins, thereby influencing the overall chemical environment and pH of milk. Additionally, factors such as the type of feed consumed by dairy cattle can indirectly influence milk's pH. For instance, diets high in certain nutrients or additives can alter the metabolic pathways in cows, leading to variations in the composition of their milk. This includes changes in fatty acid profiles and protein structures that might subtly affect the pH. In summary, the pH of milk is a dynamic equilibrium influenced by a multitude of compounds including lactic acid, casein micelles, whey proteins, and various minerals. These components interact in complex ways to maintain a stable acidic environment that is essential for milk's nutritional value and shelf life. Understanding these interactions is crucial for dairy science and technology as it allows for better control over milk quality and processing outcomes.
Factors Influencing the pH of Milk
The pH of milk is a critical factor that influences its quality, shelf life, and nutritional value. Several key factors contribute to the pH levels in milk, each playing a distinct role in its overall characteristics. One of the primary influences is bacterial fermentation, which can significantly alter the pH through the production of lactic acid and other metabolic byproducts. Additionally, storage conditions such as temperature, humidity, and exposure to light can also impact the pH of milk by affecting the rate of bacterial growth and enzymatic reactions. Furthermore, different types of milk, including cow's milk, goat's milk, and plant-based alternatives, exhibit natural variations in pH due to their unique compositions and processing methods. Understanding these factors is essential for maintaining the integrity and safety of milk products. This article will delve into these aspects, starting with the impact of bacterial fermentation on milk's pH, to provide a comprehensive overview of how these elements interact and influence the final product.
Impact of Bacterial Fermentation
Bacterial fermentation plays a pivotal role in the dairy industry, particularly in influencing the pH of milk. This process involves the action of microorganisms such as lactic acid bacteria, which convert lactose (milk sugar) into lactic acid. This metabolic activity significantly lowers the pH of milk, transforming it from a neutral to an acidic environment. The reduction in pH is crucial for several reasons: it enhances the preservation of milk by creating an environment less favorable for the growth of pathogenic bacteria, thereby extending shelf life. Additionally, the acidification process triggers the coagulation of casein proteins, which is essential for cheese production and yogurt manufacturing. The specific type and concentration of bacteria used can vary, influencing both the rate and extent of acidification. For instance, mesophilic bacteria like *Lactococcus lactis* are commonly used in cheese production, while thermophilic bacteria such as *Lactobacillus bulgaricus* and *Streptococcus thermophilus* are preferred for yogurt fermentation due to their ability to thrive at higher temperatures. The pH level achieved through fermentation also affects the texture and flavor profile of dairy products; for example, a lower pH can result in a tangier taste and a firmer texture. Furthermore, bacterial fermentation contributes to the nutritional value of dairy products by increasing their content of beneficial compounds like probiotics and bioactive peptides. These compounds have been linked to various health benefits, including improved gut health and enhanced immune function. In summary, bacterial fermentation is a critical factor influencing the pH of milk, driving significant changes that are essential for both the preservation and transformation of milk into various dairy products with distinct characteristics and nutritional profiles.
Effect of Storage Conditions on Milk's pH
The pH of milk is a critical parameter that can be significantly influenced by storage conditions, making it an essential factor to consider in dairy processing and handling. When milk is stored under optimal conditions, its pH remains relatively stable, typically ranging between 6.5 and 6.7. However, deviations from these ideal storage conditions can lead to noticeable changes in the milk's pH. **Temperature** is one of the most significant storage conditions affecting milk's pH. At refrigerated temperatures (around 4°C), the growth of psychrotrophic bacteria is slowed down, which helps maintain the natural pH of milk. Conversely, if milk is stored at higher temperatures, bacterial growth accelerates, leading to increased production of lactic acid and other metabolic by-products. This results in a decrease in pH, making the milk more acidic. For instance, if milk is left at room temperature for an extended period, its pH can drop below 6.0 due to the rapid proliferation of bacteria. **Storage Time** also plays a crucial role in altering the pH of milk. Freshly collected milk has a higher pH compared to milk that has been stored for several days. Over time, even under refrigeration, there is a gradual decrease in pH due to the natural enzymatic activities and microbial growth that occur within the milk. This effect is more pronounced when milk is not stored properly or when it is exposed to contaminants that can introduce additional bacteria. **Packaging and Handling** are other critical factors influencing the pH of stored milk. The type of packaging material used can affect the permeability of gases such as oxygen and carbon dioxide, which in turn can impact microbial growth and metabolic activities within the milk. For example, packaging materials with high oxygen permeability can lead to increased oxidation reactions that may alter the pH over time. Additionally, improper handling practices such as agitation or exposure to light can introduce oxygen into the milk, promoting bacterial growth and acid production. **Contamination** is another significant storage condition that affects milk's pH. Contaminants such as bacteria, yeast, or mold can introduce extraneous enzymes and metabolic pathways that alter the chemical composition of milk. These contaminants often produce acidic compounds as by-products of their metabolism, leading to a decrease in milk's pH. Proper sanitation and hygiene practices during milking, processing, and storage are essential to minimize contamination risks. In summary, the storage conditions of milk—temperature, storage time, packaging, handling practices, and contamination levels—collectively influence its pH. Maintaining optimal storage conditions is crucial for preserving the natural pH of milk and ensuring its quality and safety for consumption. Understanding these factors helps dairy producers and processors implement effective strategies to manage milk quality throughout the supply chain. By controlling these variables, it is possible to maintain a stable pH in milk, thereby extending its shelf life and preserving its nutritional value.
Variations in pH Across Different Types of Milk
The pH of milk can vary significantly across different types, influenced by factors such as the type of dairy animal, feed composition, and processing methods. For instance, cow's milk, which is the most commonly consumed type, typically has a pH range of 6.4 to 6.7. This slightly acidic nature is due to the presence of lactic acid and other organic acids produced during the natural fermentation process within the mammary gland. In contrast, goat's milk tends to have a slightly higher pH, often ranging from 6.5 to 6.8, attributed to its different fatty acid profile and lower concentration of lactic acid. Sheep's milk, known for its rich nutritional content and often used in cheese production, generally has a pH between 6.5 and 6.7. The higher pH in sheep's milk can be linked to its higher calcium content and the unique metabolic processes in sheep that affect the acid-base balance of their milk. Buffalo milk, another variant with high nutritional value, typically falls within a pH range of 6.7 to 7.0, reflecting its distinct fatty acid composition and lower levels of lactic acid compared to cow's milk. Beyond traditional dairy sources, plant-based milks also exhibit a wide range of pH levels. Almond milk and soy milk, for example, tend to be more alkaline with pH values often around 7.0 to 8.0 due to their base ingredients and added stabilizers. Coconut milk, on the other hand, can have a more variable pH depending on whether it is full-fat or low-fat; however, it generally falls within a slightly acidic to neutral range (pH 6.0-7.0). Oat milk and rice milk usually have pH values close to neutral (around 7.0), reflecting their minimal processing and lack of significant acidic or alkaline components. The variations in pH across these different types of milk are not merely academic; they have practical implications for both consumers and manufacturers. For consumers, understanding these differences can help in choosing the right type of milk for specific dietary needs or preferences. For manufacturers, knowing the pH of milk is crucial for ensuring proper storage, processing, and shelf life, as well as for maintaining the quality and safety of dairy products. Additionally, these variations can influence the taste, texture, and nutritional properties of milk-based products, making pH an important factor in product development and quality control. In summary, the pH of milk is not a constant value but varies significantly depending on the source and type of milk. These variations are influenced by a combination of biological, nutritional, and processing factors, making each type of milk unique in terms of its chemical properties and potential uses. Understanding these differences is essential for both consumers seeking to make informed choices and producers aiming to optimize their products' quality and shelf life.