What Is Charging By Friction
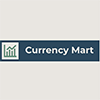
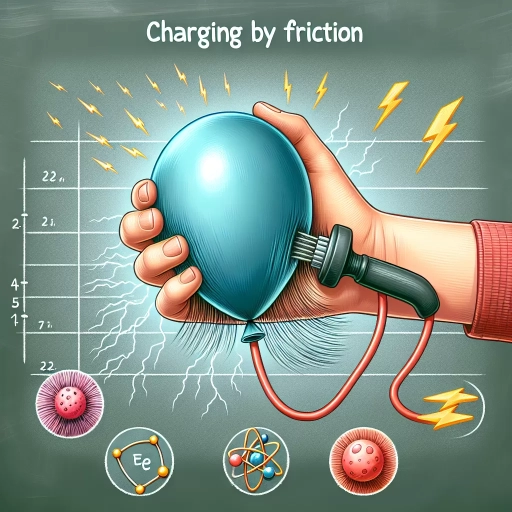
Charging by friction, a fundamental concept in physics, is a process where objects acquire electric charges through physical contact and subsequent separation. This phenomenon is not just a curiosity but has significant implications across various fields. To delve into the intricacies of charging by friction, it is essential to first understand the underlying concept. This involves grasping the principles of how electrons are transferred between materials, leading to the generation of static electricity. Once we have a solid foundation in the concept, we can explore how charging by friction actually occurs, including the role of material properties and environmental conditions. Finally, examining the applications and implications of this process reveals its importance in fields such as electronics, manufacturing, and even everyday life. By understanding these aspects, we can better appreciate the mechanisms and consequences of charging by friction. Let us begin by **Understanding the Concept of Charging by Friction**.
Understanding the Concept of Charging by Friction
Understanding the concept of charging by friction is a multifaceted topic that delves into the fundamental principles of physics, historical observations, and scientific theories. At its core, charging by friction involves the transfer of electrons between two surfaces when they come into contact, resulting in one surface becoming positively charged and the other negatively charged. To grasp this phenomenon fully, it is essential to explore three key areas: the definition and basic principles, the historical context and early observations, and the scientific theories behind frictional charging. **Definition and Basic Principles** will provide a clear understanding of what charging by friction entails, including the mechanisms by which electrons are transferred and the conditions necessary for this process to occur. This section will lay the groundwork for a deeper exploration of the subject. **Historical Context and Early Observations** will delve into how ancient civilizations first noticed and documented the effects of frictional charging, highlighting key milestones and contributions that have shaped our current understanding. **Scientific Theories Behind Frictional Charging** will examine the modern scientific explanations for why and how friction leads to the transfer of electrons, incorporating insights from materials science, electromagnetism, and quantum mechanics. By understanding these three aspects, we can gain a comprehensive insight into the intriguing world of charging by friction. Let us begin by defining and exploring the basic principles that underpin this fascinating phenomenon.
Definition and Basic Principles
**Understanding the Concept of Charging by Friction** Charging by friction, a fundamental process in the realm of electrostatics, involves the transfer of electrons between two surfaces when they come into contact and then separate. This phenomenon is rooted in the basic principles of atomic structure and the behavior of electrons at the surface of materials. **Definition and Basic Principles** Charging by friction is defined as the process where two neutral objects become electrically charged after being rubbed together. The core principle behind this is the transfer of electrons from one object to another due to differences in their electron affinities. When two surfaces are brought into contact, electrons can move from one surface to the other, resulting in one object gaining excess electrons (becoming negatively charged) while the other loses electrons (becoming positively charged). This electron transfer occurs because different materials have varying tendencies to attract and hold onto electrons. The process can be broken down into several key steps: **1) Contact**, where the surfaces of the two objects are brought together, allowing electrons to move freely between them. **2) Electron Transfer**, where electrons migrate from one surface to the other based on their relative electron affinities. **3) Separation**, where the objects are pulled apart, leaving each with a net charge due to the imbalance of electrons. For instance, when a rubber balloon is rubbed against a woolen cloth, electrons are transferred from the wool to the balloon. The wool, having lost electrons, becomes positively charged, while the balloon, having gained electrons, becomes negatively charged. This simple yet illustrative example highlights how everyday materials can exhibit charging by friction. Understanding these basic principles is crucial for grasping various electrostatic phenomena and applications, from static electricity in household items to advanced technologies involving surface interactions and charge transfer. By recognizing how different materials interact at a microscopic level, scientists and engineers can design and optimize systems that either exploit or mitigate charging by friction, depending on the desired outcome. In summary, charging by friction is an intrinsic property of material interactions that relies on electron transfer between surfaces. This fundamental concept underpins many aspects of electrostatics and continues to play a significant role in both theoretical understanding and practical applications across various fields.
Historical Context and Early Observations
The concept of charging by friction has its roots in ancient observations and experiments, providing a rich historical context that underscores the evolution of our understanding of electricity. The earliest recorded evidence of static electricity dates back to around 600 BCE, when the Greek philosopher Thales of Miletus noticed that rubbing amber against certain materials could attract light objects such as feathers and straw. This simple yet intriguing phenomenon sparked curiosity among ancient Greeks, who began to explore the properties of various substances and their ability to generate what we now recognize as static electric charges. As civilizations advanced, so did the depth of these observations. In the 17th century, English scientist William Gilbert coined the term "electricity" from the Greek word "ēlektron," meaning amber, and published his seminal work "De Magnete" in 1600. Gilbert's work not only documented his experiments with magnetism but also included detailed accounts of his observations on static electricity generated by friction. He identified that other materials besides amber could produce similar effects when rubbed against each other, laying foundational groundwork for later scientists. The 18th century saw significant advancements in understanding charging by friction, particularly through the work of Benjamin Franklin. Franklin's extensive research led him to propose the concept of positive and negative charges, which he demonstrated through his famous kite experiment during a thunderstorm in 1752. This experiment showed that lightning is a form of electricity and helped establish a clearer understanding of how friction can generate electric charges. Later, in the late 18th and early 19th centuries, scientists like Alessandro Volta and Michael Faraday further elucidated the principles behind charging by friction. Volta invented the first battery, known as the voltaic pile, which provided a steady source of electricity and allowed for more controlled experiments on electrical phenomena. Faraday's work on electrostatic induction and the discovery of the laws of electrolysis added critical pieces to the puzzle, enabling a more comprehensive grasp of how frictional forces can induce electrical charges. The historical context of charging by friction is thus marked by a series of incremental discoveries and observations that span millennia. From Thales' initial curiosity about amber to Franklin's groundbreaking experiments and the subsequent contributions of Volta and Faraday, each step has enriched our understanding of this fundamental aspect of electricity. These early observations not only highlight human ingenuity and scientific inquiry but also serve as a testament to how continuous exploration can lead to profound insights into the natural world. Understanding these historical milestones is essential for appreciating the full scope of charging by friction and its role in shaping modern electrical science.
Scientific Theories Behind Frictional Charging
**Scientific Theories Behind Frictional Charging** Frictional charging, a fundamental concept in physics, is rooted in several scientific theories that explain how the interaction between two surfaces can lead to the transfer of electrons and subsequent charging. At its core, frictional charging involves the triboelectric effect, where the physical contact and subsequent separation of materials result in an exchange of electrons. This phenomenon is governed by the principles of quantum mechanics and the behavior of electrons at the atomic level. When two materials come into contact, their surfaces interact at the molecular and atomic levels. The electrons in the outermost energy levels of these atoms can be transferred from one material to another due to differences in their electron affinities—the measure of how strongly an atom attracts electrons. Materials with higher electron affinities tend to gain electrons, becoming negatively charged, while those with lower electron affinities lose electrons, becoming positively charged. This process is influenced by factors such as the surface roughness, cleanliness, and the presence of impurities or moisture. The triboelectric series is a useful tool for predicting which material will lose or gain electrons when in contact with another. This series ranks materials based on their tendency to lose or gain electrons; for example, rubber tends to gain electrons (becoming negatively charged) when rubbed against fur, which loses electrons (becoming positively charged). The actual transfer of electrons occurs during the brief moments of contact and separation, where the mechanical stress and heat generated by friction facilitate the movement of electrons across the interface. Furthermore, the concept of work function plays a crucial role in understanding frictional charging. The work function is the minimum energy required to remove an electron from a material's surface. When two materials with different work functions come into contact, electrons flow from the material with the lower work function to the one with the higher work function until equilibrium is reached. This flow of electrons results in one material becoming positively charged and the other negatively charged. Additionally, environmental factors such as humidity and temperature can influence the efficiency of frictional charging. Moisture can reduce the effectiveness of charging by providing an alternative path for electrons to return to their original state, thereby neutralizing the charge. Conversely, dry conditions enhance the triboelectric effect by minimizing such neutralization pathways. In summary, frictional charging is a complex process driven by the interplay of electron affinities, work functions, and environmental conditions. Understanding these underlying scientific theories provides insight into why certain materials become charged when rubbed together and how this phenomenon can be harnessed or mitigated in various applications. By grasping these principles, we can better appreciate the mechanisms behind everyday observations like static electricity and develop innovative technologies that leverage or control frictional charging.
How Charging by Friction Occurs
Charging by friction, a fundamental phenomenon in physics, occurs through a complex interplay of various factors. At its core, this process involves the transfer of electrons between surfaces when they come into contact. This transfer is influenced by the properties of the materials involved, such as their electron affinity and work function. The role of surface materials and properties cannot be overstated, as different materials have varying tendencies to either gain or lose electrons. Additionally, environmental factors such as humidity and temperature can significantly impact the efficiency of this electron transfer. Understanding these elements is crucial for grasping how charging by friction occurs. By delving into the specifics of each factor, we can gain a deeper insight into this phenomenon. Let's begin by examining the transfer of electrons between surfaces, which sets the stage for understanding the broader mechanisms at play.
Transfer of Electrons Between Surfaces
The transfer of electrons between surfaces is a fundamental process that underlies the phenomenon of charging by friction. When two different materials come into contact, they exchange electrons due to differences in their electron affinities and work functions. This exchange is not symmetrical; one material tends to lose electrons (becoming positively charged), while the other gains electrons (becoming negatively charged). The material with a higher electron affinity or lower work function tends to attract and retain more electrons, leading to an imbalance in charge distribution. For instance, when a rubber balloon is rubbed against a woolen cloth, the woolen fibers tend to lose electrons to the rubber surface. This occurs because rubber has a higher electron affinity compared to wool. As a result, the rubber balloon becomes negatively charged due to the excess electrons it has acquired, while the woolen cloth becomes positively charged due to the loss of electrons. This transfer of electrons is facilitated by the frictional contact between the two surfaces, which disrupts the surface bonds and allows for electron migration. The extent of electron transfer depends on several factors, including the nature of the materials involved, the duration and intensity of contact, and environmental conditions such as humidity and temperature. In general, materials that are farther apart on the triboelectric series—a list ranking materials by their tendency to lose or gain electrons—tend to exhibit more pronounced charging effects when brought into contact. Understanding this mechanism is crucial for explaining various everyday phenomena where charging by friction occurs. For example, walking across a carpet can cause your body to become statically charged due to electron transfer from your shoes to the carpet fibers. Similarly, peeling off adhesive tape can leave it with a static charge because of electron transfer between the tape and the surface it was stuck to. In addition to these practical examples, the principle of electron transfer between surfaces has significant implications in fields such as electronics and materials science. It influences the design of devices that rely on static electricity, such as electrostatic precipitators used in air pollution control systems or electrostatic generators like Van de Graaff generators used in scientific research. In summary, the transfer of electrons between surfaces is a key process in charging by friction. It is driven by differences in electron affinity and work function between materials and facilitated by frictional contact. This fundamental principle not only explains common static electricity phenomena but also has broader applications across various scientific and technological domains.
Role of Surface Materials and Properties
The role of surface materials and their properties is pivotal in the process of charging by friction. When two different materials come into contact and then separate, electrons are transferred from one material to the other, resulting in the charging of these surfaces. This phenomenon is fundamentally driven by the differences in the electron affinity and ionization energies of the materials involved. For instance, when a piece of rubber is rubbed against a glass rod, electrons tend to move from the glass to the rubber due to the higher electron affinity of rubber compared to glass. This transfer leaves the glass rod positively charged (due to the loss of electrons) and the rubber negatively charged (due to the gain of electrons). The nature of the surface materials significantly influences this electron transfer process. Materials with high electron affinity, such as certain polymers and insulators, are more likely to attract electrons during frictional contact. Conversely, materials with lower electron affinity, like metals, tend to lose electrons more readily. The surface roughness and cleanliness also play crucial roles; smoother and cleaner surfaces can facilitate better contact between materials, enhancing the efficiency of electron transfer. Additionally, environmental factors such as humidity and temperature can affect the charging process by altering the conductivity and electron mobility on the surfaces. Understanding these properties is essential for predicting and controlling the outcome of charging by friction. For example, in industrial applications where static electricity is a concern, selecting appropriate materials with similar electron affinities can minimize unwanted charging. In scientific research, carefully choosing materials with known properties allows for controlled experiments that can shed light on the fundamental mechanisms behind triboelectric charging. Furthermore, advancements in material science have led to the development of specialized coatings and treatments that can either enhance or suppress triboelectric effects, offering practical solutions in fields ranging from electronics to textiles. In summary, the role of surface materials and their properties is central to understanding how charging by friction occurs. By recognizing how different materials interact at a molecular level during contact and separation, we can better explain why certain combinations result in significant charging while others do not. This knowledge not only aids in explaining natural phenomena but also provides a foundation for innovative technological applications where control over static electricity is critical.
Environmental Factors Influencing Frictional Charging
Environmental factors play a crucial role in influencing the process of frictional charging, which is a fundamental mechanism by which objects acquire static electricity. The primary environmental factors that impact frictional charging include humidity, temperature, air pressure, and the presence of airborne particles. **Humidity** is one of the most significant environmental factors affecting frictional charging. High humidity levels in the air can significantly reduce the amount of static electricity generated during friction. This is because moisture in the air acts as a conductor, allowing electrons to dissipate more easily and reducing the buildup of static charge. Conversely, low humidity environments facilitate higher levels of static electricity generation due to the reduced availability of moisture to neutralize charges. **Temperature** also has a notable influence on frictional charging. Generally, lower temperatures enhance the efficiency of frictional charging by reducing the thermal energy available for electron movement and dissipation. At higher temperatures, thermal agitation increases, leading to more rapid dissipation of static charges and thus reducing the overall charge accumulation. **Air pressure** can indirectly affect frictional charging by altering the density of air and its ability to conduct electricity. While air pressure itself does not directly influence static generation, changes in air pressure can affect humidity levels and air density, which in turn impact frictional charging. The **presence of airborne particles**, such as dust and pollutants, can also influence frictional charging. These particles can act as charge carriers or neutralizers depending on their composition and charge state. For instance, certain types of dust may carry a static charge that can either enhance or diminish the charging process when they come into contact with other surfaces. Additionally, **surface properties** of materials involved in friction are heavily influenced by environmental conditions. For example, surfaces that are exposed to high humidity may develop a thin layer of moisture that alters their electrical properties and reduces their ability to generate static electricity through friction. Understanding these environmental factors is crucial for predicting and controlling the outcomes of frictional charging in various applications, from industrial processes to everyday situations where static electricity might be a concern. By manipulating these factors, it is possible to optimize or mitigate the effects of static electricity generation through friction. In summary, environmental conditions such as humidity, temperature, air pressure, and the presence of airborne particles all contribute to the complex dynamics of frictional charging. Recognizing how these factors interplay allows for better management and utilization of static electricity in diverse contexts. This knowledge is essential for ensuring safety and efficiency in environments where static electricity could pose risks or opportunities.
Applications and Implications of Charging by Friction
The phenomenon of charging by friction, where two materials come into contact and exchange electrons, resulting in one becoming positively charged and the other negatively charged, has far-reaching applications and implications across various domains. This article delves into the multifaceted aspects of frictional charging, exploring its industrial uses and the critical safety considerations that accompany these applications. We will also examine everyday examples and common experiences that illustrate the ubiquity of frictional charging in our daily lives. Additionally, we will discuss the ongoing research and future developments in this field, highlighting potential advancements and innovations. By understanding these different facets, we can better appreciate the significance of frictional charging and its potential to shape both industrial practices and everyday life. Transitioning to the industrial realm, it is crucial to consider the safety implications of frictional charging, as it can pose significant risks if not managed properly, making **Industrial Uses and Safety Considerations** a paramount focus area.
Industrial Uses and Safety Considerations
**Industrial Uses and Safety Considerations** Charging by friction, a fundamental process where static electricity is generated through the contact and separation of materials, has numerous industrial applications that are both versatile and critical. In manufacturing, static electricity is harnessed to improve the efficiency of various processes. For instance, in the textile industry, static charges can be used to align fibers during the production of fabrics, enhancing their strength and uniformity. Similarly, in the automotive sector, electrostatic painting ensures a uniform coat on car bodies by attracting paint particles to the metal surface, reducing waste and improving finish quality. In the pharmaceutical industry, static charges are utilized to mix powders and granules evenly, ensuring consistent drug formulations. Additionally, in electronics manufacturing, anti-static devices and materials are essential to prevent damage to sensitive components from static discharges. However, these applications come with significant safety considerations. Static electricity can ignite flammable gases or dusts in environments such as chemical plants or grain silos, leading to catastrophic explosions. Therefore, grounding and bonding techniques are crucial to dissipate static charges safely. Moreover, personal protective equipment (PPE) designed to prevent static buildup is mandatory in many industrial settings. Workers handling sensitive electronics or working in hazardous environments must wear anti-static clothing and footwear to prevent accidental discharges. Regular maintenance of equipment and facilities is also vital; ensuring that machinery and surfaces are properly grounded can mitigate the risk of static-related accidents. In terms of safety protocols, industries often implement strict guidelines for handling materials that generate static electricity. For example, in clean rooms where semiconductor devices are manufactured, strict anti-static measures are enforced to prevent contamination and damage. Training programs for employees focus on recognizing and managing static risks, emphasizing the importance of proper grounding techniques and the use of appropriate PPE. The integration of advanced technologies such as ionizers and humidifiers further enhances safety by neutralizing static charges in the air and on surfaces. These devices are particularly useful in environments where static control is paramount but difficult to achieve through traditional methods alone. By combining these technologies with robust safety protocols and continuous employee training, industries can effectively harness the benefits of charging by friction while minimizing its inherent risks. Overall, the industrial use of charging by friction underscores the delicate balance between leveraging a powerful physical phenomenon for productivity gains and ensuring the safety of workers and facilities. By understanding and addressing the potential hazards associated with static electricity, industries can optimize their processes while maintaining a safe and reliable operational environment.
Everyday Examples and Common Experiences
Charging by friction, a fundamental concept in physics, is not just a theoretical phenomenon but an integral part of our everyday lives. This process, where two surfaces in contact generate static electricity due to the transfer of electrons, manifests in various common experiences. For instance, when you walk across a carpeted floor and then touch a metal doorknob, you might feel a slight shock. This is because the friction between your shoes and the carpet has transferred electrons from one surface to another, leaving you with an excess of electrons and thus a static charge. Similarly, in dry weather, rubbing a balloon against your hair can make it stick to a wall due to the static electricity generated by friction. In the workplace, static electricity can be both a nuisance and a hazard. For example, in manufacturing environments where sensitive electronic components are handled, static discharge can damage these components. To mitigate this, workers often wear anti-static wristbands or work on anti-static mats that dissipate static charges safely. In textile production, static electricity can cause fabrics to cling together or attract dust, affecting the quality of the final product. Here, anti-static sprays or humidifiers are used to reduce static buildup. Even in our daily grooming routines, charging by friction plays a role. When you use a hairbrush, especially on dry hair, the friction between the brush and your hair can cause your hair to stand up due to static electricity. This is why some hairbrushes are designed with anti-static materials to minimize this effect. Furthermore, in the realm of technology, understanding charging by friction is crucial for designing safe and efficient devices. For instance, in the development of high-speed printers and photocopiers, managing static electricity is essential to prevent paper jams and ensure smooth operation. The same principle applies to the handling of powders in industrial processes; static charges can cause powders to clump or stick to surfaces, affecting product quality and safety. In addition to these practical applications, charging by friction also has significant implications for environmental phenomena. For example, lightning is partially caused by the buildup of static electricity in clouds due to friction between ice crystals and water droplets. Understanding this process helps meteorologists predict weather patterns more accurately. In conclusion, charging by friction is not just a scientific concept but an omnipresent force that influences various aspects of our daily lives. From the mundane experience of getting a shock from a doorknob to the critical importance in industrial manufacturing and environmental science, this phenomenon underscores the interconnectedness of physics and everyday experiences. By recognizing and understanding these examples, we can better appreciate the intricate ways in which fundamental physical principles shape our world.
Research and Future Developments in Frictional Charging
Research and future developments in frictional charging are pivotal in unlocking the full potential of this phenomenon, which has been a cornerstone of static electricity since ancient times. Current studies are focused on understanding the intricate mechanisms behind frictional charging, particularly at the nanoscale. Scientists are employing advanced techniques such as atomic force microscopy and scanning electron microscopy to observe and analyze the transfer of electrons between surfaces during frictional contact. These investigations aim to elucidate the role of surface roughness, material properties, and environmental conditions in determining the efficiency and polarity of charge transfer. One promising area of research involves the development of novel materials with tailored tribological properties. Researchers are designing and synthesizing materials that can enhance or control the frictional charging process, such as nanomaterials with specific surface functionalities or composite materials with optimized mechanical properties. For instance, graphene and other 2D materials have shown remarkable potential due to their high surface area and unique electronic properties, which can significantly influence the frictional charging behavior. Another significant direction is the integration of frictional charging into energy harvesting technologies. By leveraging the principle of triboelectricity, researchers are developing innovative devices that can convert mechanical energy from everyday activities—such as walking or vibrations—into electrical energy. These triboelectric generators (TEGs) hold great promise for powering small electronic devices in remote or resource-constrained environments, thereby contributing to the development of sustainable and self-sustaining systems. Future developments also include the exploration of biomedical applications. Frictional charging can be harnessed to create implantable devices that generate power from bodily movements, potentially revolutionizing the field of bioelectronics. Additionally, there is ongoing research into using frictional charging for sensing applications, such as detecting changes in pressure or vibration, which could have significant implications for industrial monitoring and healthcare diagnostics. Moreover, advancements in computational modeling and simulation are crucial for predicting and optimizing frictional charging phenomena. Researchers are developing sophisticated models that account for the complex interactions between surfaces at the atomic level, allowing for better design and optimization of devices that rely on frictional charging. These computational tools will be essential for scaling up the technology and ensuring its reliability in various practical applications. In conclusion, ongoing research and future developments in frictional charging are poised to transform our understanding and utilization of this fundamental phenomenon. By advancing our knowledge of the underlying mechanisms, developing new materials and technologies, and exploring diverse applications, scientists are paving the way for innovative solutions that could impact fields ranging from energy harvesting to biomedical engineering. As these advancements continue to unfold, we can expect significant improvements in the efficiency, reliability, and versatility of devices that harness the power of frictional charging.