What Is Effective Nuclear Charge
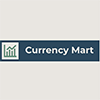
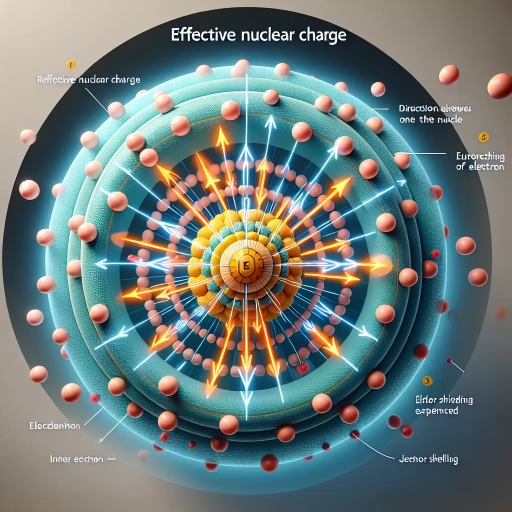
Effective nuclear charge is a fundamental concept in chemistry that plays a crucial role in understanding the behavior of atoms and their interactions. It refers to the net positive charge experienced by an electron in an atom, which is influenced by the number of protons in the nucleus and the shielding effect of inner electrons. This concept is pivotal in explaining various chemical properties and phenomena. In this article, we will delve into the intricacies of effective nuclear charge, starting with a comprehensive explanation of what it entails. We will then explore the factors that influence effective nuclear charge, including electron shielding and the atomic number. Finally, we will discuss the applications and implications of effective nuclear charge in chemistry, highlighting its significance in molecular bonding and reactivity. By understanding these aspects, readers will gain a deeper insight into how effective nuclear charge shapes the chemical landscape. Let us begin by **Understanding Effective Nuclear Charge**.
Understanding Effective Nuclear Charge
Understanding effective nuclear charge is a fundamental concept in chemistry that underpins the behavior of atoms and molecules. This crucial idea is rooted in the definition and concept of nuclear charge, which involves the attraction between the positively charged nucleus and the negatively charged electrons in an atom. Historically, the development of this concept has been shaped by key scientific discoveries and theories, from the early models of atomic structure to modern quantum mechanics. The importance of effective nuclear charge in chemistry cannot be overstated; it influences atomic radius, electronegativity, and ionization energy, thereby affecting chemical bonding and reactivity. By delving into the definition and concept, historical development, and importance in chemistry, we can gain a comprehensive understanding of effective nuclear charge and its pivotal role in shaping the chemical properties of elements. This article will explore these facets to provide a thorough insight into understanding effective nuclear charge.
Definition and Concept
Effective nuclear charge is a fundamental concept in chemistry that helps explain the behavior of electrons within an atom. At its core, effective nuclear charge refers to the net positive charge experienced by an electron in a multi-electron atom. This concept is crucial because it accounts for the shielding effect, where inner electrons partially shield outer electrons from the full nuclear charge. The actual nuclear charge is the total positive charge of the nucleus, but due to shielding, outer electrons experience a reduced effective nuclear charge. Understanding effective nuclear charge is essential for predicting various chemical properties and behaviors. For instance, as you move across a period in the periodic table, the effective nuclear charge increases due to the addition of protons to the nucleus and the inefficient shielding by electrons in the same energy level. This increase in effective nuclear charge leads to a decrease in atomic radius and an increase in electronegativity, making elements more reactive as you move from left to right across a period. Conversely, moving down a group in the periodic table, the effective nuclear charge remains relatively constant because each new energy level adds an additional shell of electrons that effectively shields the outermost electrons from the increasing nuclear charge. This results in larger atomic radii and lower electronegativities as you descend a group. The concept of effective nuclear charge also plays a significant role in explaining trends in ionization energy and electron affinity. Higher effective nuclear charges result in higher ionization energies because it becomes more difficult to remove an electron from an atom with a stronger electrostatic pull. Similarly, higher effective nuclear charges can lead to higher electron affinities as atoms with stronger electrostatic attractions are more likely to attract additional electrons. In summary, effective nuclear charge is a critical concept that helps chemists understand how electrons interact with the nucleus and other electrons within an atom. By considering this concept, chemists can predict various chemical properties and trends across the periodic table, providing a deeper understanding of atomic behavior and reactivity. This foundational knowledge is indispensable for advancing our understanding of chemical bonding, reactivity, and the periodic relationships that govern the behavior of elements.
Historical Development
The concept of effective nuclear charge has its roots in the historical development of atomic theory, particularly in the early 20th century. The journey began with Ernest Rutherford's 1911 gold foil experiment, which led to the proposal of the nuclear atom model. This model posited that atoms consist of a small, dense nucleus containing protons and neutrons, surrounded by electrons in orbitals. However, this simplistic view did not fully explain the complexities of atomic structure and electron behavior. The next significant milestone was the introduction of quantum mechanics by Niels Bohr in 1913. Bohr's atomic model introduced energy levels or shells where electrons reside, but it still did not account for the detailed interactions between electrons and the nucleus. It was not until the development of the quantum mechanical model by Erwin Schrödinger in 1926 that a more comprehensive understanding emerged. Schrödinger's wave mechanics provided a framework for understanding how electrons are distributed within an atom, laying the groundwork for modern atomic theory. In the 1930s, the concept of effective nuclear charge began to take shape. This idea was crucial because it acknowledged that the actual charge experienced by an electron in a multi-electron atom is less than the full nuclear charge due to shielding by inner electrons. The effective nuclear charge is a measure of this reduced charge, which varies depending on the electron's position within the atom. This concept was further refined through the work of scientists like John Slater and others who developed empirical rules to estimate effective nuclear charges for different electrons within an atom. Understanding effective nuclear charge is pivotal because it helps explain several key phenomena in chemistry and physics. For instance, it clarifies why ionization energies increase across a period in the periodic table and why certain elements exhibit specific chemical properties. Effective nuclear charge also plays a critical role in molecular orbital theory, influencing bond strengths and molecular geometry. As our understanding of atomic structure has evolved, so too has our ability to predict and explain a wide range of chemical and physical behaviors at the atomic level. In summary, the historical development of effective nuclear charge is intertwined with major advancements in atomic theory from Rutherford's nuclear atom to Schrödinger's quantum mechanics and beyond. This concept has been refined over decades through empirical observations and theoretical models, providing a fundamental tool for understanding electron behavior and chemical properties. Its significance extends beyond theoretical interest; it is essential for predicting and explaining various phenomena in chemistry and physics, making it a cornerstone of modern atomic theory.
Importance in Chemistry
Understanding the concept of effective nuclear charge is pivotal in chemistry, as it underpins many fundamental principles that govern the behavior of atoms and molecules. Effective nuclear charge refers to the net positive charge experienced by an electron in a multi-electron atom, which is less than the actual nuclear charge due to shielding by inner electrons. This concept is crucial for several reasons. Firstly, it influences the atomic radius: as effective nuclear charge increases across a period, the electrons are drawn closer to the nucleus, resulting in a decrease in atomic radius. This, in turn, affects chemical reactivity and the ability of an atom to form bonds. For instance, elements with higher effective nuclear charges tend to be more electronegative and thus more likely to attract electrons in covalent bonds. Secondly, effective nuclear charge plays a significant role in determining ionization energy—the energy required to remove an electron from an atom. As the effective nuclear charge increases, it becomes more difficult to remove an electron, leading to higher ionization energies. This explains why ionization energy generally increases across a period and decreases down a group in the periodic table. Understanding these trends is essential for predicting chemical properties and reactivity patterns of elements. Moreover, effective nuclear charge impacts the stability of ions and the formation of ionic compounds. For example, elements with high effective nuclear charges are more likely to form stable anions by gaining electrons, while those with low effective nuclear charges tend to form stable cations by losing electrons. This principle is fundamental in understanding ionic bonding and the structure of ionic compounds. In addition, the concept of effective nuclear charge is integral to understanding molecular orbital theory and the distribution of electrons within molecules. It helps explain why certain molecules exhibit specific geometries and why some bonds are stronger than others. For instance, in molecules like CO₂, the effective nuclear charge on carbon influences the hybridization of its orbitals, leading to a linear molecular geometry. Finally, effective nuclear charge has practical implications in various fields such as materials science and biochemistry. In materials science, understanding how effective nuclear charge affects the properties of materials can help in designing new materials with specific characteristics. In biochemistry, it helps explain the behavior of ions and molecules in biological systems, which is crucial for understanding metabolic pathways and drug interactions. In summary, the concept of effective nuclear charge is a cornerstone of chemistry that explains a wide range of atomic and molecular phenomena. Its importance lies in its ability to predict chemical properties, reactivity patterns, and molecular structures, making it an indispensable tool for chemists across various disciplines.
Factors Influencing Effective Nuclear Charge
Effective nuclear charge is a fundamental concept in chemistry that influences the behavior of atoms and their interactions. It refers to the net positive charge experienced by an electron in an atom, which is crucial for understanding various chemical properties and reactivity. However, this charge is not a fixed value but is influenced by several key factors. One significant factor is the shielding effect by inner electrons, which reduces the effective nuclear charge by blocking some of the positive charge from the nucleus. Another critical factor is the penetration of outer electrons, where electrons in different orbitals have varying degrees of proximity to the nucleus, affecting how much they experience the nuclear charge. Additionally, the atomic number and electron configuration play pivotal roles in determining the effective nuclear charge, as they dictate the arrangement and number of electrons around the nucleus. Understanding these factors is essential for grasping the concept of effective nuclear charge, which in turn helps explain many aspects of atomic and molecular behavior. By delving into these influences, we can gain a deeper insight into the intricate dynamics at play within atoms and how they shape chemical phenomena. This article will explore each of these factors in detail to provide a comprehensive understanding of effective nuclear charge.
Shielding Effect by Inner Electrons
The shielding effect by inner electrons is a crucial factor influencing the effective nuclear charge experienced by outer electrons in an atom. This phenomenon arises because the inner electrons, which are closer to the nucleus, partially shield the outer electrons from the full attractive force of the positively charged nucleus. As a result, the effective nuclear charge felt by the outer electrons is reduced compared to the actual nuclear charge. To understand this concept better, consider an atom with multiple electron shells. The innermost electrons occupy the lowest energy levels and are positioned closest to the nucleus. These inner electrons effectively "shield" or "screen" the outer electrons from the full nuclear charge by distributing their negative charge around the nucleus. This distribution creates a partial cancellation of the positive charge of the nucleus, leading to a reduced effective nuclear charge for the outer electrons. The extent of shielding depends on several factors, including the number of inner electrons and their orbital configurations. For instance, electrons in s-orbitals are more effective at shielding than those in p-orbitals due to their spherical symmetry and closer proximity to the nucleus. Additionally, as you move down a group in the periodic table, the number of inner electron shells increases, which enhances the shielding effect and reduces the effective nuclear charge experienced by the outermost electrons. This shielding effect has significant implications for atomic properties such as ionization energy and electron affinity. For example, despite an increase in actual nuclear charge as you move across a period from left to right, the effective nuclear charge experienced by outer electrons may not increase proportionally due to incomplete shielding by inner electrons. This explains why ionization energies generally increase across a period but with some irregularities. In summary, the shielding effect by inner electrons is essential for understanding how effective nuclear charge varies within an atom. It highlights how the arrangement and number of inner electrons can significantly influence the electrostatic environment experienced by outer electrons, thereby impacting various chemical and physical properties of elements. This concept underscores the complex interplay between electrons and the nucleus in determining atomic behavior and reactivity.
Penetration of Outer Electrons
The penetration of outer electrons plays a crucial role in understanding the concept of effective nuclear charge, which is a fundamental principle in chemistry. Effective nuclear charge refers to the net positive charge experienced by an electron in an atom, taking into account the shielding effect of inner electrons. Penetration, in this context, describes how deeply an electron can approach the nucleus. Electrons in lower energy levels (or shells) penetrate closer to the nucleus compared to those in higher energy levels. This proximity affects the effective nuclear charge felt by these electrons. When outer electrons penetrate closer to the nucleus, they experience a stronger attraction due to the increased proximity to the positive charge of the protons. This increased attraction results in a higher effective nuclear charge for these electrons. Conversely, electrons that do not penetrate as deeply are shielded more effectively by inner electrons and thus experience a lower effective nuclear charge. The degree of penetration varies among different types of orbitals; for instance, s-orbitals penetrate more than p-orbitals, which in turn penetrate more than d-orbitals. This variation in penetration leads to differences in the effective nuclear charge experienced by electrons in different orbital types. The impact of electron penetration on effective nuclear charge is evident in several chemical phenomena. For example, it influences ionization energies and electron affinities. Atoms with electrons that penetrate closer to the nucleus tend to have higher ionization energies because these electrons are more tightly bound. Similarly, electron affinity is affected as atoms with higher penetrating power tend to attract additional electrons more strongly. Understanding the penetration of outer electrons is essential for predicting atomic properties and chemical behavior, making it a critical component of the broader concept of effective nuclear charge. In summary, the penetration of outer electrons significantly influences the effective nuclear charge experienced by these electrons. By considering how deeply electrons approach the nucleus and how this affects their interaction with the positive charge of protons, chemists can better understand various atomic and chemical properties. This nuanced understanding is vital for explaining and predicting a wide range of chemical phenomena, underscoring the importance of electron penetration in the study of effective nuclear charge.
Atomic Number and Electron Configuration
The atomic number and electron configuration are fundamental concepts in understanding the structure of atoms, which in turn play a crucial role in determining the effective nuclear charge. The atomic number, denoted by Z, is the number of protons present in the nucleus of an atom and defines the element's identity in the periodic table. This number dictates how many electrons an atom will have in its neutral state, as the number of electrons equals the number of protons. Electron configuration, on the other hand, describes how these electrons are arranged in various energy levels or shells around the nucleus. It follows specific rules such as the Aufbau principle and Hund's rule, which ensure that electrons occupy the lowest available energy levels and that each orbital within a subshell is singly occupied before any pairing occurs. Understanding electron configuration is essential because it influences the effective nuclear charge experienced by each electron. Effective nuclear charge is the net positive charge felt by an electron due to the protons in the nucleus minus the shielding effect caused by other electrons. As electrons fill higher energy levels, they experience a reduced effective nuclear charge due to increased shielding from inner-shell electrons. This shielding effect diminishes as you move across a period in the periodic table because additional protons in the nucleus increase the effective nuclear charge, pulling electrons closer to the nucleus despite increased electron-electron repulsion. Moreover, electron configuration affects chemical properties and reactivity. For instance, atoms with full outer energy levels (noble gases) are chemically inert due to their stable electron configuration, while atoms with partially filled outer levels are more reactive as they seek to achieve a stable configuration through chemical bonding. The arrangement of electrons also influences ionization energy and electron affinity, which are critical in understanding how atoms interact with other atoms and molecules. In summary, the atomic number sets the stage for understanding electron configuration, which in turn significantly influences the effective nuclear charge experienced by electrons. This interplay between atomic number, electron configuration, and effective nuclear charge underpins many fundamental principles in chemistry and physics, making it a cornerstone of atomic theory and essential for predicting and explaining various chemical phenomena.
Applications and Implications of Effective Nuclear Charge
Effective nuclear charge, a fundamental concept in chemistry, plays a pivotal role in understanding various chemical phenomena. This article delves into the applications and implications of effective nuclear charge, exploring its significance across multiple dimensions. First, we will examine how effective nuclear charge influences the prediction of ionization energy and electron affinity, crucial parameters that determine an atom's ability to lose or gain electrons. Next, we will discuss its impact on chemical bonding and reactivity, shedding light on how this concept shapes the formation and stability of molecules. Finally, we will analyze the broader implications of effective nuclear charge on periodic trends and properties, highlighting how it underpins the periodic table's structure and the diverse characteristics of elements. By understanding these facets, we can gain a deeper insight into the underlying principles that govern chemical behavior, ultimately leading to a comprehensive understanding of effective nuclear charge itself.
Predicting Ionization Energy and Electron Affinity
Predicting ionization energy and electron affinity are crucial aspects of understanding the chemical behavior of elements, and these predictions are significantly influenced by the concept of effective nuclear charge. Effective nuclear charge, which is the net positive charge experienced by an electron in a multi-electron atom, plays a pivotal role in determining how easily an electron can be removed (ionization energy) or added (electron affinity) to an atom. Ionization energy, the energy required to remove an electron from a neutral atom, increases as the effective nuclear charge increases. This is because a higher effective nuclear charge results in a stronger attraction between the nucleus and the electrons, making it more difficult to remove an electron. For instance, elements in the same period (horizontal row) of the periodic table exhibit increasing ionization energies as you move from left to right due to the increase in effective nuclear charge. Conversely, elements in the same group (vertical column) show decreasing ionization energies as you move down the group because the outermost electrons are farther away from the nucleus, reducing the effective nuclear charge they experience. Electron affinity, which is the energy change when an electron is added to a neutral atom, also correlates with effective nuclear charge. Generally, atoms with higher effective nuclear charges tend to have more negative electron affinities because they attract additional electrons more strongly. However, there are exceptions due to electron configuration and the stability of certain electron shells. For example, elements like nitrogen and oxygen have relatively low electron affinities compared to their neighbors because adding an electron would disrupt their stable half-filled or full subshells. Understanding these trends is essential for predicting chemical reactivity and stability. For instance, metals typically have low ionization energies and positive electron affinities, making them good electron donors in chemical reactions. Nonmetals, on the other hand, have high ionization energies and negative electron affinities, making them good electron acceptors. This knowledge is critical in fields such as materials science, where the design of new materials often relies on the manipulation of electron configurations to achieve desired properties. In summary, the effective nuclear charge is a fundamental concept that underpins our ability to predict ionization energies and electron affinities. By understanding how this charge influences these properties, chemists can better explain and predict a wide range of chemical phenomena, from the reactivity of elements to the stability of compounds. This predictive power has far-reaching implications across various scientific disciplines, enabling advancements in fields ranging from catalysis and drug design to energy storage and electronic materials.
Understanding Chemical Bonding and Reactivity
Understanding chemical bonding and reactivity is fundamental to grasping the intricacies of molecular interactions and transformations. At its core, chemical bonding involves the sharing or transfer of electrons between atoms to form stable molecules. This process is deeply influenced by the concept of effective nuclear charge, which refers to the net positive charge experienced by an electron in a multi-electron atom. Effective nuclear charge plays a crucial role in determining the strength and nature of chemical bonds. In atoms, the nuclear charge is the positive charge of the nucleus, but in multi-electron atoms, inner electrons shield outer electrons from this full nuclear charge, reducing its effective value. This shielding effect varies depending on the electron configuration and the presence of other electrons in the same or inner shells. As a result, effective nuclear charge increases as you move across a period in the periodic table due to the addition of protons to the nucleus without a corresponding increase in shielding by inner electrons. Conversely, it decreases down a group because additional energy levels add more shielding electrons. The implications of effective nuclear charge on chemical bonding are profound. For instance, elements with higher effective nuclear charges tend to form stronger bonds due to a greater attraction between the nucleus and electrons. This is why fluorine, with its high effective nuclear charge, forms very strong bonds with other elements, making it highly reactive. Conversely, elements like cesium, which have lower effective nuclear charges due to extensive shielding, form weaker bonds and are less reactive. Effective nuclear charge also influences reactivity patterns. For example, in molecules where atoms have significantly different electronegativities (a measure of an atom's ability to attract electrons), the bond polarity increases. This polarity arises because atoms with higher effective nuclear charges pull shared electrons closer to themselves, leading to partial positive and negative charges within the molecule. Such polar bonds are more reactive than nonpolar bonds because they can participate in dipole-dipole interactions and other intermolecular forces that facilitate chemical reactions. Moreover, understanding effective nuclear charge helps predict the stability and reactivity of molecules. For instance, molecules with high bond energies (which are often a result of strong nuclear-electron attraction) are more stable and less reactive than those with lower bond energies. This knowledge is crucial in fields such as organic chemistry and materials science where designing stable yet reactive molecules is essential for synthesizing new compounds and materials. In summary, effective nuclear charge is a pivotal concept in understanding chemical bonding and reactivity. By influencing the strength and nature of chemical bonds, it shapes the reactivity patterns of elements and molecules. Recognizing how effective nuclear charge varies across the periodic table allows chemists to predict and manipulate molecular properties, enabling advancements in various scientific disciplines. This fundamental understanding underpins many applications in chemistry, from drug design to materials engineering, highlighting the critical role of effective nuclear charge in modern science.
Impact on Periodic Trends and Properties
Effective nuclear charge significantly influences periodic trends and properties, shaping the behavior of elements across the periodic table. As you move from left to right within a period, the effective nuclear charge increases due to the addition of protons in the nucleus without a corresponding increase in electron shielding. This rise in effective nuclear charge pulls electrons closer to the nucleus, resulting in a decrease in atomic radius and an increase in electronegativity. Consequently, elements on the right side of a period tend to form ions with higher charges and exhibit stronger tendencies to attract electrons in covalent bonds. In contrast, moving down a group, the effective nuclear charge remains relatively constant because each additional energy level adds more electrons and protons but also introduces additional electron shells that shield the nucleus effectively. This leads to an increase in atomic radius and a decrease in electronegativity as you descend a group. The consistent effective nuclear charge down a group explains why elements in the same group exhibit similar chemical properties despite differences in their physical properties. The impact of effective nuclear charge is also evident in ionization energy and electron affinity trends. As effective nuclear charge increases across a period, it becomes more difficult to remove an electron from an atom, leading to higher ionization energies. Conversely, as effective nuclear charge decreases down a group, ionization energy decreases because it becomes easier to remove an electron from an atom with more energy levels and greater shielding. Furthermore, effective nuclear charge affects the reactivity of elements. For instance, metals on the left side of the periodic table have lower effective nuclear charges and thus tend to lose electrons easily to form positive ions, making them highly reactive. In contrast, nonmetals on the right side have higher effective nuclear charges and tend to gain electrons to form negative ions, making them less reactive but highly electronegative. Understanding these trends is crucial for predicting chemical behavior and explaining various phenomena such as the formation of ionic and covalent bonds, the stability of molecules, and the reactivity of elements in different chemical reactions. The concept of effective nuclear charge provides a fundamental framework for interpreting periodic trends and properties, enabling chemists to make informed predictions about how elements will behave under different conditions. This knowledge has significant implications for fields like materials science, where understanding the properties of elements is essential for designing new materials with specific characteristics. In summary, effective nuclear charge is a pivotal concept that underpins our understanding of periodic trends and properties, facilitating a deeper insight into the chemical behavior of elements.