What Is Zeff
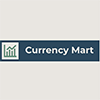
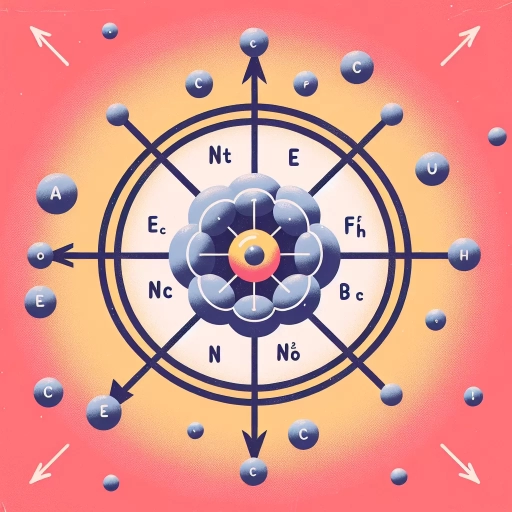
In the realm of atomic physics, the concept of Zeff, or effective nuclear charge, plays a pivotal role in understanding the behavior of electrons within an atom. This fundamental idea is crucial for grasping how electrons interact with the nucleus and other electrons, influencing various chemical and physical properties. The article delves into the multifaceted nature of Zeff, starting with an in-depth exploration of **Understanding the Concept of Zeff**, where we dissect the theoretical underpinnings and how it is calculated. We then examine **Applications and Uses of Zeff**, highlighting its significance in fields such as chemistry and materials science. Finally, we address **Criticisms and Limitations of Zeff**, discussing the challenges and limitations associated with this concept. By navigating these aspects, readers will gain a comprehensive understanding of Zeff's importance and its practical implications. Let us begin by **Understanding the Concept of Zeff**, the foundation upon which all other discussions are built.
Understanding the Concept of Zeff
Understanding the concept of Zeff, or effective nuclear charge, is a fundamental aspect of atomic physics that has far-reaching implications in chemistry and materials science. This concept, which describes the net positive charge experienced by an electron in a multi-electron atom, is crucial for predicting the chemical properties and behaviors of elements. To delve into the intricacies of Zeff, it is essential to explore its definition and historical context, tracing back to the early 20th century when scientists began to grapple with the complexities of atomic structure. The theoretical background and development of Zeff involve key principles from quantum mechanics and the work of pioneers like Erwin Schrödinger and Niels Bohr. Additionally, understanding the contributions of key scientists who have shaped our current understanding of Zeff is vital, as their research has refined our ability to calculate and apply this concept effectively. By examining these facets—definition and historical context, theoretical background and development, and key contributors and their roles—we can gain a comprehensive understanding of the concept of Zeff.
Definition and Historical Context
The concept of Zeff, or effective nuclear charge, is deeply rooted in the historical development of atomic theory and quantum mechanics. To understand Zeff, it is crucial to delve into its definition and historical context. Zeff represents the net positive charge experienced by an electron in a multi-electron atom, which is different from the actual nuclear charge due to the shielding effect of inner electrons. This concept emerged as scientists sought to explain the behavior of electrons within atoms more accurately. Historically, the journey to defining Zeff began with the early 20th-century models of the atom. The Rutherford model, proposed by Ernest Rutherford in 1911, introduced the idea of a small, dense nucleus surrounded by electrons. However, this model did not account for electron-electron interactions or the distribution of electrons within the atom. It was not until the development of quantum mechanics by Niels Bohr, Louis de Broglie, and Erwin Schrödinger that a more nuanced understanding of atomic structure began to take shape. In the 1920s and 1930s, quantum mechanics provided a framework for understanding how electrons occupy specific energy levels or orbitals around the nucleus. The concept of shielding became central as it was recognized that inner electrons shield outer electrons from the full nuclear charge, reducing the effective charge experienced by these outer electrons. This realization led to the development of the effective nuclear charge (Zeff) as a way to quantify this effect. The work of physicists like Hans Bethe and Enrico Fermi further refined these ideas, incorporating them into more sophisticated models of atomic structure. By the mid-20th century, Zeff had become a fundamental tool in understanding chemical properties and electron configurations. It helps explain why certain elements exhibit specific trends in their periodic behavior, such as ionization energies and electron affinities. In contemporary chemistry and physics, Zeff remains a vital concept for predicting and explaining various phenomena at the atomic level. It is used in computational chemistry to model molecular interactions and in materials science to understand the properties of solids. The evolution of Zeff from early atomic models to its current application underscores the continuous refinement of our understanding of atomic structure and electron behavior. Understanding Zeff is essential for grasping how atoms interact with each other and how their properties are influenced by the interplay between nuclear charge and electron shielding. This concept bridges the gap between theoretical models and empirical observations, providing a robust framework for interpreting chemical phenomena across diverse fields. As research continues to advance our knowledge of atomic interactions, the significance of Zeff as a foundational concept remains unwavering.
Theoretical Background and Development
The theoretical background and development of the concept of Zeff, or effective nuclear charge, are deeply rooted in the evolution of atomic theory and quantum mechanics. The journey begins with the early 20th-century models of the atom, particularly the Rutherford model, which introduced the concept of a nucleus surrounded by electrons. However, this model was soon found to be insufficient due to its inability to explain the stability of atoms and the emission spectra of elements. The next significant milestone was the development of quantum mechanics by pioneers such as Niels Bohr, Erwin Schrödinger, and Werner Heisenberg. Bohr's atomic model introduced energy levels and electron shells, laying the groundwork for understanding how electrons interact with the nucleus. Schrödinger's wave mechanics provided a more comprehensive framework by describing electrons in terms of probability distributions rather than definite positions. The concept of Zeff emerged as a way to reconcile the complexities of multi-electron atoms with the simplicity of hydrogen-like models. In a multi-electron atom, inner electrons shield the outer electrons from the full nuclear charge, reducing the effective nuclear charge experienced by these outer electrons. This shielding effect was first quantitatively addressed by Erwin Madelung in his work on atomic structure and later refined by other scientists. One of the key contributors to this field was John Slater, who developed Slater's rules for estimating Zeff. These rules provide a systematic approach to calculating the effective nuclear charge by considering the shielding effects of different electron groups. Slater's work was instrumental in making Zeff a practical tool for chemists and physicists to predict chemical properties and behaviors of atoms. The development of computational methods and density functional theory (DFT) further enhanced our ability to calculate Zeff with greater accuracy. DFT allows for the treatment of many-electron systems by approximating the exchange-correlation functional, which includes effects such as electron-electron interactions and shielding. Understanding Zeff is crucial because it bridges the gap between theoretical models and empirical observations. It helps explain why certain elements exhibit specific chemical properties, such as ionization energies and electron affinities. Moreover, Zeff is essential in predicting molecular structures and reactivity patterns, making it a cornerstone in fields like chemistry and materials science. In summary, the theoretical background and development of Zeff are intertwined with major advancements in atomic theory and quantum mechanics. From early atomic models to sophisticated computational techniques, each step has refined our understanding of how electrons interact with nuclei in multi-electron atoms. This evolution has transformed Zeff from a conceptual tool into a precise method for predicting chemical behavior, underscoring its importance in modern scientific inquiry.
Key Contributors and Their Roles
Understanding the concept of Zeff, or effective nuclear charge, is deeply rooted in the contributions of several key figures in chemistry and physics. One of the most pivotal contributors is Erwin Madelung, a German physicist who introduced the concept of effective nuclear charge in the early 20th century. Madelung's work laid the foundation for understanding how electrons in an atom experience a reduced nuclear charge due to the shielding effect of inner electrons. This idea revolutionized the way chemists and physicists approached atomic structure and electron configuration. Another significant contributor is Niels Bohr, whose atomic model provided a framework for understanding electron shells and their relationship with the nucleus. Bohr's model, although simplified compared to modern quantum mechanics, was instrumental in visualizing how electrons are arranged around the nucleus and how this arrangement affects the effective nuclear charge experienced by outer electrons. His work complemented Madelung's by providing a visual and conceptual basis for understanding atomic structure. The development of quantum mechanics by pioneers such as Werner Heisenberg and Erwin Schrödinger further refined our understanding of Zeff. Their work introduced wave mechanics and the concept of electron probability distributions, which allowed for more precise calculations of effective nuclear charges. These advancements enabled chemists to predict chemical properties and reactivity with greater accuracy, as they could now account for the subtle variations in effective nuclear charge across different elements. Additionally, the contributions of Linus Pauling should not be overlooked. Pauling's work on electronegativity and his development of the concept of hybridization provided practical tools for chemists to apply the principles of effective nuclear charge in predicting molecular structures and properties. His approach made it possible to correlate the effective nuclear charge with observable chemical phenomena, bridging theoretical physics with practical chemistry. In contemporary times, computational chemists have played a crucial role in refining our understanding of Zeff through advanced computational methods. Researchers like John Pople and Walter Kohn have developed sophisticated algorithms and models that allow for precise calculations of effective nuclear charges in complex molecular systems. These computational tools have enabled scientists to study a wide range of chemical phenomena with unprecedented accuracy, further solidifying our grasp on the concept of Zeff. In summary, the concept of Zeff is a culmination of contributions from various scientists across different eras. From Madelung's initial formulation to the refinements brought about by quantum mechanics and computational chemistry, each contributor has added a layer of depth to our understanding of how electrons interact with the nucleus. This collective effort has transformed our ability to predict and explain chemical behavior, making Zeff a cornerstone in modern chemistry and physics.
Applications and Uses of Zeff
The concept of Zeff, or effective nuclear charge, is a fundamental principle in chemistry and physics that underpins our understanding of atomic and molecular behavior. This critical concept influences various aspects of chemical and physical phenomena, making it essential to explore its applications and uses comprehensively. In this article, we will delve into the multifaceted nature of Zeff by examining its role in **Chemical Bonding and Molecular Structure**, where it affects the formation and stability of chemical bonds. We will also discuss **Electron Configuration and Atomic Properties**, highlighting how Zeff shapes the arrangement of electrons within atoms and their resulting properties. Additionally, we will explore **Practical Implications in Chemistry and Physics**, illustrating how Zeff impacts real-world applications across these disciplines. By understanding these interconnected themes, we can gain a deeper insight into the concept of Zeff and its far-reaching implications, ultimately enhancing our grasp of the underlying principles that govern the behavior of matter at the atomic level. This comprehensive approach will lead us to a nuanced understanding of the concept of Zeff.
Chemical Bonding and Molecular Structure
Chemical bonding and molecular structure are fundamental concepts in chemistry that underpin the understanding of how atoms interact to form molecules. At the heart of these interactions lies the principle of electron sharing or transfer, which gives rise to various types of chemical bonds. The most common types include covalent bonds, where electrons are shared between atoms, ionic bonds, where electrons are transferred from one atom to another, and metallic bonds, which involve the delocalization of electrons among a lattice of metal atoms. Understanding these bonding mechanisms is crucial because they determine the shape, stability, and reactivity of molecules. The molecular structure, influenced by these bonds, can be described using several models such as the Lewis structure, VSEPR (Valence Shell Electron Pair Repulsion) theory, and molecular orbital theory. The Lewis structure provides a simple representation of electron distribution within a molecule, while VSEPR theory predicts the three-dimensional arrangement of atoms based on electron pair repulsions. Molecular orbital theory offers a more detailed view by describing the distribution of electrons in terms of molecular orbitals formed from atomic orbitals. These concepts have far-reaching implications across various fields. For instance, in materials science, understanding chemical bonding helps in designing new materials with specific properties such as strength, conductivity, or optical characteristics. In pharmaceuticals, knowledge of molecular structure is essential for drug design and development; drugs often work by interacting with specific biological molecules through precise spatial arrangements. Additionally, in environmental science, understanding how pollutants form and interact at a molecular level can inform strategies for their removal or degradation. The applications of these principles extend to advanced technologies as well. For example, in nanotechnology, precise control over chemical bonding allows for the creation of nanostructures with unique properties. In energy storage and conversion devices like batteries and solar cells, the efficiency of charge transfer depends on the molecular structure of the materials involved. In the context of Zeff (effective nuclear charge), which is a measure of the net positive charge experienced by an electron in a multi-electron atom, understanding chemical bonding and molecular structure becomes even more critical. Zeff influences how electrons are distributed within an atom and thus affects the formation of chemical bonds. A higher Zeff can lead to stronger bonds due to increased electron attraction towards the nucleus. This concept is particularly relevant in predicting trends in periodic properties and explaining why certain elements form specific types of compounds. In summary, chemical bonding and molecular structure are foundational to understanding how atoms combine to form molecules with unique properties. These principles have widespread applications across various scientific disciplines and technologies, making them indispensable tools for scientists and engineers seeking to develop new materials, drugs, and technologies. The interplay between these concepts and Zeff further underscores their importance in predicting and explaining chemical behavior at both the atomic and molecular levels.
Electron Configuration and Atomic Properties
Electron configuration is a fundamental concept in chemistry that describes the arrangement of electrons within an atom, which in turn influences various atomic properties. This arrangement is crucial because it determines the chemical behavior and physical characteristics of an element. The electron configuration is typically represented by a series of numbers and letters that indicate the energy levels (shells) and subshells where electrons are located. For instance, the electron configuration of carbon is \(1s^2 2s^2 2p^2\), indicating that the first shell has two electrons in the s-orbital, and the second shell has two electrons in the s-orbital and two electrons in the p-orbitals. Understanding electron configuration is essential for predicting atomic properties such as atomic radius, electronegativity, and ionization energy. The atomic radius, for example, generally decreases across a period (from left to right) due to the increasing effective nuclear charge (\(Z_{eff}\)), which pulls electrons closer to the nucleus. This trend is directly related to the electron configuration; as more protons are added to the nucleus and more electrons occupy the same principal energy level, the effective nuclear charge increases, leading to a smaller atomic radius. Electronegativity, another key property influenced by electron configuration, measures an atom's ability to attract electrons in a covalent bond. Elements with higher \(Z_{eff}\) values tend to have higher electronegativities because their outermost electrons are more tightly bound to the nucleus. This is evident when comparing elements across a period; as \(Z_{eff}\) increases, so does the electronegativity. Ionization energy, the energy required to remove an electron from an atom, is also significantly affected by electron configuration. Atoms with full or half-full subshells (stable configurations) have higher ionization energies due to the stability of these arrangements. For example, helium (\(1s^2\)) and neon (\(1s^2 2s^2 2p^6\)) have particularly high ionization energies because their outermost energy levels are completely filled. In practical terms, understanding these relationships between electron configuration and atomic properties is vital for various applications. For instance, in materials science, knowing how changes in electron configuration affect atomic radius and electronegativity helps in designing materials with specific properties such as conductivity or reactivity. In chemistry labs, predicting ionization energies based on electron configurations aids in understanding chemical reactivity and stability of compounds. The effective nuclear charge (\(Z_{eff}\)) plays a central role in these phenomena because it quantifies the net positive charge experienced by an electron in an atom. As \(Z_{eff}\) increases across a period due to the addition of protons and electrons in the same shell, it directly impacts atomic radius, electronegativity, and ionization energy. Thus, understanding electron configuration provides a foundational framework for comprehending these critical atomic properties and their applications in various fields.
Practical Implications in Chemistry and Physics
The practical implications of chemistry and physics are multifaceted and far-reaching, particularly when considering the concept of effective nuclear charge, or Zeff. This fundamental principle in atomic physics has significant applications across various fields, underscoring its importance in both theoretical and applied sciences. In chemistry, Zeff plays a crucial role in understanding the periodic trends of elements. For instance, as you move across a period in the periodic table, the effective nuclear charge increases due to the addition of electrons to the same principal energy level without a corresponding increase in shielding. This increase in Zeff leads to a decrease in atomic radius and an increase in electronegativity, which are critical factors in determining chemical reactivity and bonding properties. These trends are essential for predicting the behavior of elements in different chemical environments, thereby guiding the synthesis of new compounds and materials. In physics, Zeff is pivotal in the study of atomic structure and spectroscopy. The effective nuclear charge influences the energy levels of electrons within an atom, which in turn affects the emission and absorption spectra. Understanding these spectra is vital for various analytical techniques such as atomic absorption spectroscopy (AAS) and inductively coupled plasma mass spectrometry (ICP-MS), which are widely used in environmental monitoring, forensic science, and industrial quality control. Moreover, the concept of Zeff is integral to quantum mechanics, where it helps in the development of more accurate models of atomic behavior. This has implications for advanced technologies like semiconductor manufacturing and quantum computing, where precise control over atomic interactions is necessary. Furthermore, the practical implications extend into materials science and engineering. The manipulation of Zeff through doping or alloying can significantly alter the properties of materials. For example, in semiconductor technology, adjusting the effective nuclear charge by introducing impurities allows for the creation of p-type and n-type semiconductors, which are fundamental components in electronic devices. Similarly, in nanotechnology, understanding how Zeff affects the electronic structure of nanoparticles is crucial for designing materials with specific optical, electrical, or magnetic properties. In addition to these technological applications, Zeff also has implications for our understanding of biological systems. The effective nuclear charge influences the chemical properties of ions and molecules, which are essential for biological processes such as enzyme catalysis and protein folding. This knowledge can be leveraged to design drugs that target specific biochemical pathways more effectively. In summary, the concept of Zeff has profound practical implications across chemistry, physics, materials science, and even biology. Its influence on atomic structure, chemical reactivity, and material properties makes it a cornerstone for advancing various technologies and understanding complex systems. As research continues to refine our understanding of Zeff, its applications will likely expand further, driving innovation in multiple fields.
Criticisms and Limitations of Zeff
The concept of Zeff, or effective nuclear charge, is a fundamental principle in chemistry that helps explain the attraction between electrons and the nucleus in an atom. However, despite its utility, Zeff is not without its criticisms and limitations. This article delves into the various challenges and shortcomings associated with Zeff, providing a comprehensive overview of its assumptions and simplifications, comparisons with other models, and modern alternatives and improvements. By examining these aspects, we can better understand the inherent complexities and the evolving nature of atomic theory. The assumptions and simplifications underlying Zeff will be scrutinized to highlight where this model falls short. Additionally, comparisons with other models will reveal how Zeff stands in relation to more advanced or alternative theories. Finally, an exploration of modern alternatives and improvements will shed light on how scientists are refining our understanding of atomic interactions. Through this analysis, we aim to enhance our understanding of the concept of Zeff and its place within the broader landscape of atomic theory. Understanding the Concept of Zeff requires a nuanced appreciation of these critiques and advancements, which will be explored in detail in the following sections.
Assumptions and Simplifications
When delving into the criticisms and limitations of Zeff, a crucial aspect to consider is the role of assumptions and simplifications. Zeff, as a theoretical framework, relies heavily on certain assumptions about human behavior, economic conditions, and market dynamics. These assumptions often simplify complex realities to make the model more manageable and predictable. However, this simplification can lead to significant deviations from real-world scenarios, thereby undermining the model's accuracy and applicability. For instance, Zeff assumes that investors act rationally and make decisions based on complete information. This assumption overlooks the inherent biases and emotional factors that influence investment decisions in real life. Behavioral finance has shown that investors are prone to cognitive biases such as confirmation bias, loss aversion, and herd behavior, which can significantly alter market outcomes. By ignoring these psychological aspects, Zeff may fail to capture the full spectrum of market dynamics. Another critical assumption involves the stability of economic conditions. Zeff often assumes a steady-state economy with minimal external shocks. However, real-world economies are subject to numerous unpredictable events such as geopolitical tensions, natural disasters, and technological disruptions. These external factors can drastically alter market conditions and render Zeff's predictions less reliable. Furthermore, Zeff simplifies the complexity of financial markets by assuming uniformity among investors. In reality, investors have diverse risk tolerance levels, investment horizons, and information sets. This heterogeneity can lead to diverse reactions to market stimuli, which may not be accurately captured by Zeff's homogeneous assumptions. Additionally, the model's reliance on historical data for future predictions is another simplification that can be problematic. Historical data may not reflect future market conditions accurately due to structural changes in the economy or unforeseen events. This issue is particularly pertinent in today's rapidly changing world where technological advancements and global interconnectedness are redefining traditional economic paradigms. In conclusion, while Zeff provides a useful framework for understanding certain aspects of financial markets, its reliance on assumptions and simplifications limits its predictive power and real-world applicability. Recognizing these limitations is essential for a more nuanced understanding of Zeff's strengths and weaknesses, allowing for more informed decision-making in financial analysis and investment strategies. By acknowledging the complexities that Zeff simplifies or overlooks, practitioners can better integrate this model into a broader toolkit that accounts for the multifaceted nature of financial markets.
Comparisons with Other Models
When evaluating the criticisms and limitations of Zeff, it is crucial to compare it with other models in the same domain to provide a comprehensive understanding of its strengths and weaknesses. Zeff, as a cutting-edge AI model, faces competition from several other advanced AI systems such as GPT-4, Bard, and LaMDA. One key comparison lies in their training data and algorithms. Unlike GPT-4, which is trained on a vast and diverse dataset that includes a wide range of internet content, Zeff's training data may be more curated and focused on specific domains. This can result in Zeff excelling in niche areas but potentially lacking the broad general knowledge base of GPT-4. Another significant comparison is in terms of interpretability and transparency. Models like LaMDA are designed with more emphasis on explainability, allowing users to understand the reasoning behind the AI's responses. In contrast, Zeff might prioritize performance over interpretability, which could be a limitation for users who need to understand the underlying logic of the AI's outputs. Additionally, Bard's ability to incorporate real-time information and updates makes it more dynamic compared to Zeff, which may rely on pre-trained data that could become outdated. From a usability perspective, Zeff's interface and user experience can be compared to that of other models. For instance, GPT-4 offers a more intuitive and user-friendly interface that caters to a broader audience, including non-technical users. Zeff, on the other hand, might require more technical expertise to fully leverage its capabilities, which could limit its accessibility. Moreover, the ethical considerations and safety features of these models also warrant comparison. LaMDA and Bard have been developed with robust safety protocols to prevent harmful or biased outputs. Zeff's approach to ethical AI might differ, and any shortcomings in this area could be a significant limitation. In terms of scalability and deployment, Zeff may face challenges similar to those encountered by other large language models. The computational resources required to run Zeff efficiently could be substantial, making it less feasible for widespread adoption compared to more lightweight models. Overall, while Zeff offers unique advantages in specific domains, its limitations become more apparent when compared to other models. Understanding these comparisons is essential for critiquing Zeff's performance and identifying areas for improvement. By acknowledging these differences, developers can work towards enhancing Zeff's capabilities and addressing its limitations to make it a more robust and versatile AI tool.
Modern Alternatives and Improvements
In the context of criticisms and limitations of Zeff, it is crucial to explore modern alternatives and improvements that address the shortcomings of this traditional framework. Zeff, a widely used method for estimating the economic value of a company, has been criticized for its simplicity and lack of nuance in capturing complex market dynamics. To overcome these limitations, several modern alternatives have emerged, offering more sophisticated and accurate valuation techniques. One significant improvement is the use of discounted cash flow (DCF) models, which provide a more detailed and forward-looking approach to valuation. Unlike Zeff, which relies on historical data and simple multiples, DCF models forecast future cash flows and discount them back to their present value using a cost of capital. This method allows for a more precise estimation of a company's intrinsic value by accounting for growth rates, risk factors, and other critical variables. Another advancement is the application of Monte Carlo simulations and scenario analysis. These tools enable analysts to incorporate uncertainty and variability into their valuations, providing a range of possible outcomes rather than a single point estimate. This approach is particularly useful in volatile markets where traditional methods like Zeff may fail to capture the full spectrum of potential outcomes. Additionally, the integration of machine learning and artificial intelligence (AI) has revolutionized the field of corporate valuation. AI-driven models can analyze vast amounts of data, including non-financial metrics such as social media sentiment and customer feedback, to provide a more holistic view of a company's value. These models can also identify patterns and trends that might be overlooked by traditional methods, leading to more accurate and dynamic valuations. Furthermore, the increasing adoption of real options analysis (ROA) offers another layer of sophistication. ROA recognizes that companies have flexibility in their investment decisions and can adjust strategies in response to changing market conditions. This approach values not just the company's assets but also its strategic options, providing a more comprehensive picture of its economic value. In conclusion, while Zeff remains a foundational tool in corporate valuation, its limitations are being addressed by modern alternatives that offer greater precision, flexibility, and adaptability. By leveraging advanced methodologies such as DCF models, Monte Carlo simulations, AI-driven analytics, and real options analysis, analysts can achieve more accurate and insightful valuations that better reflect the complexities of contemporary business environments. These improvements not only enhance the reliability of valuation estimates but also provide stakeholders with a clearer understanding of a company's true economic worth.