What Are The Properties Of Air
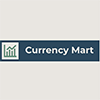
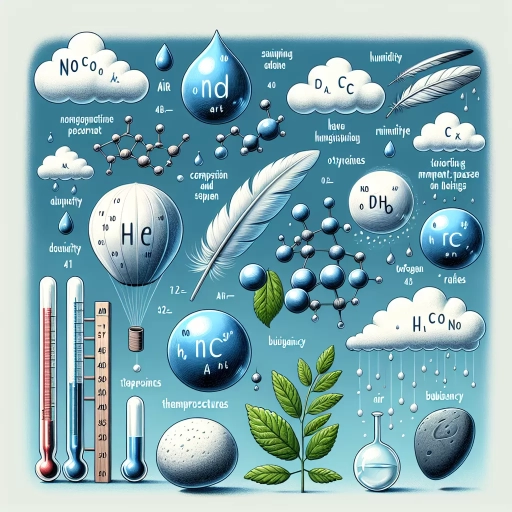
Air, the invisible yet indispensable medium that surrounds us, is often taken for granted despite its critical role in sustaining life and influencing our environment. To truly appreciate the complexity and importance of air, it is essential to delve into its various properties. This article will explore the multifaceted nature of air, examining its physical properties, chemical composition, and dynamic characteristics. We will begin by discussing the physical properties of air, which include its density, temperature, and pressure—factors that significantly impact weather patterns and atmospheric conditions. Next, we will delve into the chemical composition of air, highlighting the mix of gases that make up our atmosphere and their respective roles. Finally, we will examine the dynamic properties of air, including its movement and behavior under different conditions. By understanding these aspects, we can gain a deeper appreciation for the intricate balance and functionality of our atmosphere. Let us start by exploring the physical properties of air, which form the foundation of our atmospheric environment.
Physical Properties of Air
Understanding the physical properties of air is crucial for various scientific and engineering applications, as these properties influence how air behaves under different conditions. This article delves into three key aspects of air's physical properties: density and specific gravity, viscosity and fluidity, and thermal conductivity. Each of these properties plays a significant role in understanding air's behavior in various contexts. Density and specific gravity are essential for calculating the mass of air in a given volume, which is vital in fields such as meteorology and aviation. Viscosity and fluidity determine how air resists flow and how easily it can be moved, affecting phenomena like wind resistance and air flow in ducts. Thermal conductivity, on the other hand, governs how efficiently air can transfer heat, impacting climate modeling and HVAC systems. By exploring these properties in depth, we gain a comprehensive understanding of air's behavior and its implications across diverse disciplines. Let's begin by examining the first of these critical properties: density and specific gravity.
Density and Specific Gravity
**Density and Specific Gravity** When discussing the physical properties of air, two crucial concepts that often come into play are density and specific gravity. **Density** is defined as the mass per unit volume of a substance, typically expressed in units such as kilograms per cubic meter (kg/m³) or grams per cubic centimeter (g/cm³). For air, density varies significantly with temperature and pressure. At standard atmospheric conditions (20°C and 1 atm), the density of dry air is approximately 1.2 kg/m³. This value decreases with increasing temperature due to the expansion of air molecules and increases with higher pressure due to the compression of these molecules. **Specific gravity**, on the other hand, is a dimensionless quantity that represents the ratio of the density of a substance to the density of water at a reference temperature (usually 4°C). Since water has a density of about 1 g/cm³ at this temperature, specific gravity essentially tells us how dense a substance is relative to water. For gases like air, specific gravity is calculated by comparing their densities to that of air at standard conditions. Given that air's density is much lower than that of water, its specific gravity is significantly less than 1. Understanding these properties is vital in various fields such as engineering, meteorology, and environmental science. In engineering, knowing the density of air helps in designing systems like ventilation systems and wind turbines where airflow characteristics are critical. In meteorology, changes in air density due to temperature and humidity variations are essential for predicting weather patterns and understanding atmospheric circulation. Additionally, in environmental science, the density of air affects the dispersion of pollutants and particulate matter in the atmosphere. The interplay between density and specific gravity also influences many everyday phenomena. For instance, hot air balloons rise because heated air expands and becomes less dense than the surrounding cooler air, illustrating how changes in temperature affect air's density. Similarly, aircraft performance is heavily dependent on air density; pilots must adjust for variations in air density due to altitude and weather conditions to ensure safe flight operations. In summary, density and specific gravity are fundamental physical properties that underpin our understanding of air's behavior under different conditions. These concepts are not only theoretically important but also have practical applications across various disciplines, making them essential components in the broader study of air's properties.
Viscosity and Fluidity
**Viscosity and Fluidity** Viscosity and fluidity are fundamental physical properties that describe the behavior of fluids, including gases like air. Viscosity is a measure of a fluid's resistance to flow, essentially quantifying how "thick" or "thin" it is. The higher the viscosity, the more resistance a fluid offers to flow, while lower viscosity indicates less resistance. In contrast, fluidity is the reciprocal of viscosity, representing how easily a fluid can flow. For air, viscosity is relatively low compared to liquids. At standard temperature and pressure (STP), the viscosity of air is approximately 1.8 × 10^-5 kg/m·s, which is much lower than that of water or oil. This low viscosity allows air to flow easily and smoothly, which is crucial for various natural and industrial processes. For instance, in meteorology, the low viscosity of air enables it to circulate freely around the globe, influencing weather patterns and climate conditions. The viscosity of air can be influenced by temperature and pressure. Generally, as temperature increases, the viscosity of air decreases slightly due to increased molecular motion. Conversely, higher pressures can slightly increase viscosity because molecules are packed more tightly together. These variations are important in engineering applications such as aerodynamics and HVAC systems where precise control over airflow is necessary. Fluidity, being inversely related to viscosity, means that air has high fluidity due to its low viscosity. This high fluidity allows air to penetrate small spaces and fill containers quickly, which is essential for processes like respiration in living organisms and the operation of pneumatic tools in industry. Understanding viscosity and fluidity is critical in various fields such as aviation, where aerodynamic performance depends on the interaction between air and aircraft surfaces. In environmental science, these properties help explain phenomena like wind patterns and the dispersion of pollutants in the atmosphere. In summary, the low viscosity and high fluidity of air are key physical properties that underpin many natural and technological processes. These characteristics make air an ideal medium for numerous applications ranging from weather dynamics to industrial engineering, highlighting their importance in understanding the broader physical properties of air.
Thermal Conductivity
Thermal conductivity, a fundamental physical property, plays a crucial role in understanding how air and other materials transfer heat. In the context of air, thermal conductivity is the measure of how efficiently heat energy is conducted through the gas. Unlike solids and liquids, gases like air have relatively low thermal conductivity due to the greater distance between molecules, which reduces the frequency and effectiveness of molecular collisions that facilitate heat transfer. At standard temperature and pressure (STP), the thermal conductivity of air is approximately 0.024 W/m·K, which is significantly lower than that of metals but comparable to other gases. The thermal conductivity of air is influenced by several factors, including temperature, pressure, and humidity. As temperature increases, the kinetic energy of air molecules also increases, leading to more frequent and energetic collisions, thereby enhancing thermal conductivity. Conversely, at lower temperatures, the reduced kinetic energy results in less efficient heat transfer. Pressure affects thermal conductivity by altering the density of air; higher pressures compress the gas, bringing molecules closer together and increasing the rate of heat transfer. Humidity also impacts thermal conductivity because water vapor has a higher thermal conductivity than dry air, so moist air tends to conduct heat more efficiently than dry air. Understanding the thermal conductivity of air is essential in various engineering and scientific applications. For instance, in building insulation, knowing the thermal conductivity of air helps in designing materials that minimize heat loss or gain, thereby improving energy efficiency. In aerospace engineering, accurate calculations of air's thermal conductivity are critical for predicting heat transfer around aircraft and spacecraft, which is vital for maintaining optimal operating temperatures. Additionally, in meteorology, thermal conductivity influences atmospheric circulation patterns and weather phenomena by affecting how heat is distributed within the atmosphere. In everyday life, the thermal conductivity of air affects our comfort and energy consumption. For example, in heating and cooling systems, air's low thermal conductivity means that it is often necessary to use fans or blowers to distribute heated or cooled air effectively throughout a space. This principle is also seen in natural ventilation techniques where architects design buildings to maximize airflow, leveraging air's thermal properties to maintain comfortable indoor temperatures without relying heavily on mechanical systems. In summary, the thermal conductivity of air is a critical physical property that underpins many aspects of our daily lives and technological advancements. Its relatively low value compared to other materials necessitates careful consideration in engineering designs and everyday applications, ensuring efficient heat transfer and energy management. As part of the broader discussion on the physical properties of air, understanding thermal conductivity provides valuable insights into how this ubiquitous gas behaves under various conditions, enhancing our ability to harness its properties for practical benefits.
Chemical Composition of Air
The chemical composition of air is a complex and dynamic mixture that sustains life on Earth. Understanding its components is crucial for appreciating the delicate balance of our atmosphere. This article delves into the key elements that make up the air we breathe, focusing on three critical aspects: the dominant presence of nitrogen and oxygen, the roles of trace gases, and the impact of water vapor and humidity. Nitrogen and oxygen, which together account for nearly 99% of the atmosphere, form the backbone of air's composition. Trace gases, though present in smaller quantities, play vital roles in regulating climate and atmospheric chemistry. Water vapor, a significant variable component, influences humidity levels and weather patterns. By examining these elements, we gain a comprehensive understanding of how air's chemical composition supports life and shapes our environment. Let's begin by exploring the foundational role of nitrogen and oxygen in the air we breathe.
Nitrogen and Oxygen Content
Nitrogen and oxygen are the two most abundant components of the Earth's atmosphere, collectively making up approximately 99% of the air we breathe. Nitrogen, with its molecular formula N₂, constitutes about 78% of the atmospheric composition. This inert gas plays a crucial role in various biological and chemical processes, including the synthesis of amino acids and nucleotides, which are essential for life. Despite its abundance, nitrogen is relatively unreactive under normal conditions due to the strong triple bond between its atoms, making it a stable component of the atmosphere. Oxygen, with its molecular formula O₂, accounts for roughly 21% of the atmospheric composition. It is a highly reactive gas that supports respiration in most living organisms by facilitating the process of cellular respiration, where oxygen is used to produce energy from food. Oxygen also plays a vital role in combustion reactions, which are fundamental to many industrial processes and natural phenomena such as wildfires. The balance between nitrogen and oxygen in the atmosphere is crucial for maintaining life on Earth; any significant deviation from this balance could have profound effects on ecosystems and human health. The stability of nitrogen and the reactivity of oxygen are key factors in their respective roles within the Earth's ecosystem. Nitrogen's inertness helps maintain atmospheric stability, while oxygen's reactivity supports metabolic processes and chemical reactions essential for life. The interplay between these two gases is also influenced by other atmospheric components like trace gases (such as argon, carbon dioxide, and water vapor) and pollutants, which can alter local air quality and global climate patterns. Understanding the nitrogen and oxygen content in air is vital for various scientific disciplines, including meteorology, ecology, and environmental science. For instance, changes in oxygen levels can indicate alterations in ocean health or forest productivity due to their role in photosynthesis and respiration cycles. Similarly, monitoring nitrogen levels helps track pollution from agricultural runoff or industrial activities that release nitrogen oxides into the atmosphere. In summary, the chemical composition of air is predominantly defined by its nitrogen and oxygen content. These gases not only support life but also influence Earth's climate and ecosystems. Their precise balance ensures that our atmosphere remains conducive to life as we know it, making them integral components of our planet's ecological health.
Trace Gases and Their Roles
Trace gases, though present in minute concentrations, play pivotal roles in the Earth's atmosphere, significantly influencing its chemical composition and overall environmental health. These gases, including methane (CH₄), nitrous oxide (N₂O), ozone (O₃), and chlorofluorocarbons (CFCs), among others, are crucial components of the air we breathe. Methane, for instance, is a potent greenhouse gas with a global warming potential 28 times higher than carbon dioxide over a 100-year time frame. It originates from natural sources such as wetlands and agricultural activities, as well as human activities like natural gas production and landfills. Nitrous oxide, another trace gas, is also a strong greenhouse gas and contributes to stratospheric ozone depletion. It is emitted through agricultural practices, industrial processes, and the burning of fossil fuels. Ozone, while harmful at ground level where it forms part of smog, is vital in the stratosphere where it protects life on Earth by absorbing harmful ultraviolet radiation from the sun. However, the depletion of stratospheric ozone due to CFCs and other halogenated compounds has been a significant environmental concern, leading to international agreements like the Montreal Protocol aimed at phasing out these substances. Chlorofluorocarbons, once widely used as refrigerants and propellants, have been largely banned due to their role in ozone depletion but still linger in the atmosphere due to their long lifetimes. Other trace gases such as sulfur dioxide (SO₂) and nitrogen oxides (NOₓ) contribute to acid rain formation and air pollution, affecting both human health and ecosystems. Sulfur dioxide primarily comes from the combustion of fossil fuels by power plants and automobiles, while nitrogen oxides are emitted during high-temperature combustion processes in vehicles and industrial facilities. These gases can react with water, oxygen, and other chemicals in the atmosphere to form sulfuric and nitric acids, which then fall to the ground as acid rain, damaging forests, lakes, and buildings. In addition to their direct impacts on climate and air quality, trace gases also influence the Earth's energy balance. For example, water vapor (H₂O), though not typically considered a trace gas due to its higher concentration compared to others, is a critical greenhouse gas that amplifies the warming effect of other gases. Its concentration varies significantly with temperature and humidity levels, making it a key factor in climate feedback loops. Understanding the roles of trace gases is essential for addressing global environmental challenges such as climate change, air pollution, and ozone depletion. By monitoring their concentrations and mitigating their harmful effects through policy and technological innovations, we can work towards maintaining a healthier and more sustainable atmosphere. This knowledge also underscores the importance of comprehensive air quality management strategies that consider the interplay between various trace gases and their collective impact on the chemical composition of air. Ultimately, recognizing the significance of trace gases highlights our responsibility to manage and protect the delicate balance of our atmospheric environment.
Water Vapor and Humidity
Water vapor and humidity are crucial components of the Earth's atmosphere, playing a significant role in the chemical composition of air. Water vapor, the gaseous form of water, is a vital constituent of air, making up approximately 0.01% to 4% of the atmosphere's volume, depending on temperature and humidity levels. This variability is due to the fact that air's capacity to hold water vapor increases with temperature; warmer air can hold more moisture than cooler air. Humidity, which is the measure of the amount of water vapor present in the air, is categorized into three main types: absolute humidity, relative humidity, and specific humidity. Absolute humidity refers to the total mass of water vapor in a given volume of air, while relative humidity is the ratio of the amount of water vapor in the air to the maximum amount the air can hold at a given temperature. Specific humidity, on the other hand, is the mass of water vapor per unit mass of air. The presence of water vapor in the atmosphere has several important implications. It acts as a greenhouse gas, trapping heat and contributing to the Earth's climate system. This process is essential for maintaining a habitable temperature on Earth. Additionally, water vapor is a key factor in weather patterns and precipitation. When air cools, its capacity to hold water vapor decreases, leading to condensation and the formation of clouds, fog, or dew. These processes are fundamental to the hydrological cycle, which involves the continuous movement of water between the Earth and the atmosphere. Understanding humidity is also critical for various practical applications. In meteorology, humidity measurements are used to predict weather conditions and potential storms. In agriculture, knowing the humidity levels helps in managing crop health and optimizing irrigation practices. In industrial settings, controlling humidity is essential for maintaining product quality and preventing damage from moisture. Moreover, the interplay between water vapor and other atmospheric gases influences air quality and human health. High humidity can exacerbate respiratory issues by making it harder for the body to cool itself through sweating. Conversely, very low humidity can lead to dry skin and respiratory discomfort. The balance of water vapor in the air also affects the concentration of other pollutants; for instance, high humidity can increase the formation of ground-level ozone and particulate matter. In summary, water vapor and humidity are integral to the chemical composition of air, influencing climate, weather patterns, and various practical applications. Their dynamic interaction with other atmospheric components underscores their importance in understanding and managing our environment effectively. As a component of air's chemical makeup, water vapor's role in maintaining Earth's climate and supporting life makes it a fascinating and critical aspect of atmospheric science.
Dynamic Properties of Air
The dynamic properties of air are multifaceted and play a crucial role in understanding various atmospheric phenomena. Air, as a fluid, exhibits characteristics that are influenced by several key factors, each of which contributes to its overall behavior. This article delves into three primary aspects: Pressure and Atmospheric Conditions, Temperature and Heat Transfer, and Wind and Air Movement. Pressure and Atmospheric Conditions are fundamental in shaping the environment around us. Atmospheric pressure, which varies with altitude and weather patterns, affects everything from weather forecasting to aviation safety. Understanding these conditions is essential for predicting weather events and ensuring safe flight operations. Temperature and Heat Transfer are equally important, as they influence the thermal properties of air. The transfer of heat within the atmosphere drives climate patterns and weather systems, impacting global climate conditions and local weather forecasts. Wind and Air Movement complete the picture by explaining how air circulates globally and locally. Wind patterns are driven by temperature differences and pressure gradients, influencing weather systems, ocean currents, and even the distribution of pollutants. By examining these three interrelated aspects—Pressure and Atmospheric Conditions, Temperature and Heat Transfer, and Wind and Air Movement—we gain a comprehensive understanding of the dynamic properties of air. Let us begin by exploring the critical role of Pressure and Atmospheric Conditions in shaping our environment.
Pressure and Atmospheric Conditions
Pressure and atmospheric conditions are fundamental aspects of understanding the dynamic properties of air. Atmospheric pressure, the force exerted by the weight of air in the atmosphere, varies significantly with altitude and weather patterns. At sea level, standard atmospheric pressure is approximately 1013 millibars (mbar) or 1 atmosphere (atm), but this decreases as one ascends, reaching about half of its sea-level value at an altitude of around 5 kilometers. This variation in pressure is crucial for aviation and weather forecasting, as it influences air density and the behavior of weather systems. The relationship between pressure and temperature is another key factor. According to the ideal gas law, pressure and temperature are directly proportional when volume is constant. This means that as temperature increases, so does the pressure of the air, assuming no change in volume. Conversely, a decrease in temperature results in lower pressure. This principle is evident in weather phenomena such as high and low-pressure systems, where temperature gradients drive air movement and influence weather patterns. Humidity, or the amount of water vapor in the air, also plays a significant role in atmospheric conditions. Air can hold varying amounts of moisture depending on its temperature; warmer air can hold more water vapor than cooler air. When air reaches its dew point—the temperature at which it becomes saturated with water vapor and can no longer hold any more moisture—it condenses, forming clouds or precipitation. This process is integral to the Earth's hydrological cycle and affects both local weather and global climate patterns. Additionally, atmospheric pressure and conditions are influenced by geographical factors such as topography and latitude. Mountainous regions experience lower atmospheric pressures due to their higher elevations, while areas near the equator generally have higher temperatures and thus higher pressures compared to polar regions. These geographical variations contribute to diverse climatic zones around the globe. Understanding these aspects of pressure and atmospheric conditions is essential for predicting weather patterns, managing aviation safety, and comprehending broader environmental phenomena. For instance, meteorologists use pressure systems to forecast weather events such as storms or fair weather. Similarly, pilots must be aware of changes in atmospheric pressure to ensure safe flight operations. In conclusion, the interplay between pressure and atmospheric conditions forms a complex yet vital component of the dynamic properties of air. By grasping these principles—how pressure varies with altitude and temperature, how humidity affects air behavior, and how geographical factors influence local climates—we can better appreciate the intricate mechanisms that govern our atmosphere and its impact on our daily lives. This knowledge not only enhances our ability to predict and prepare for various weather scenarios but also underscores the importance of continued research into the ever-changing dynamics of our atmosphere.
Temperature and Heat Transfer
Temperature and heat transfer are fundamental concepts that play a crucial role in understanding the dynamic properties of air. Temperature, a measure of the average kinetic energy of particles in a substance, is essential for determining the thermal state of air. When air is heated, its molecules gain kinetic energy and move more rapidly, causing the air to expand. This expansion can lead to changes in air pressure and density, which are critical factors in atmospheric science and meteorology. For instance, warm air rises because it is less dense than cooler air, a principle that drives convection currents and influences weather patterns. Heat transfer, the movement of thermal energy from one body to another due to a temperature difference, occurs through three primary mechanisms: conduction, convection, and radiation. In the context of air, convection is particularly significant. As heated air rises and cooler air sinks, it creates circulation patterns that facilitate heat distribution within the atmosphere. This process is vital for regulating Earth's climate and weather systems. For example, ocean currents and wind patterns are driven by these convective processes, distributing heat around the globe and maintaining regional climate conditions. Radiative heat transfer also plays a crucial role in the Earth's energy balance. The sun emits solar radiation that heats the Earth's surface, which then radiates infrared radiation back into the atmosphere. Greenhouse gases such as carbon dioxide and water vapor absorb this infrared radiation, trapping heat and contributing to the greenhouse effect. This natural process helps maintain Earth's temperature at a level conducive to life but can be disrupted by human activities that increase greenhouse gas concentrations. Understanding temperature and heat transfer is essential for various applications, including engineering, meteorology, and environmental science. In engineering, knowledge of heat transfer is critical for designing efficient heating and cooling systems, such as HVAC systems that regulate indoor air quality and temperature. In meteorology, understanding how temperature and heat transfer influence atmospheric circulation helps predict weather patterns and climate trends. Additionally, recognizing the impact of human activities on global temperature and heat transfer processes informs policies aimed at mitigating climate change. In summary, temperature and heat transfer are integral components of the dynamic properties of air. They influence atmospheric circulation, weather patterns, and global climate conditions. By grasping these concepts, scientists and engineers can better predict and manage environmental phenomena, ensuring a more sustainable future for our planet.
Wind and Air Movement
Wind and air movement are fundamental aspects of the dynamic properties of air, influencing various environmental and climatic phenomena. Wind is essentially the movement of air from high-pressure areas to low-pressure areas, driven by the uneven heating of the Earth's surface by the sun. This differential heating creates temperature gradients, which in turn generate pressure differences. As air moves from high to low pressure, it follows the path of least resistance, often resulting in the formation of wind patterns. The speed and direction of wind are influenced by several factors, including the Coriolis effect, which is caused by the Earth's rotation. This effect deflects moving objects to the right in the Northern Hemisphere and to the left in the Southern Hemisphere, leading to the development of large-scale circulation patterns such as trade winds and westerlies. Additionally, topography plays a crucial role; mountains and valleys can redirect wind flows, creating localized wind patterns like mountain breezes and valley breezes. Air movement is also affected by the concept of buoyancy. Warm air is less dense than cool air, causing it to rise and create areas of low pressure near the ground. Conversely, cool air sinks, forming high-pressure zones. This process drives convection currents, which are essential for weather phenomena such as thunderstorms and the formation of clouds. Furthermore, air movement is integral to global climate regulation. Wind helps distribute heat around the globe through ocean currents and atmospheric circulation. For instance, the Gulf Stream carries warm water from the equator towards the North Pole, moderating temperatures in Western Europe. Similarly, atmospheric circulation patterns like jet streams and Hadley cells facilitate the transfer of heat and moisture across different latitudes. Understanding wind and air movement is crucial for various practical applications. Meteorologists rely on these dynamics to predict weather patterns and issue forecasts. In aviation, pilots must consider wind direction and speed to navigate safely and efficiently. Additionally, wind energy is harnessed using wind turbines, providing a renewable source of power. In conclusion, wind and air movement are vital components of the dynamic properties of air, shaping our climate, weather, and daily lives. Their study helps us comprehend complex environmental processes and leverage these forces for sustainable energy solutions and improved weather forecasting. By grasping these principles, we can better appreciate the intricate balance of our atmosphere and its role in sustaining life on Earth.