What Animal Cannot Walk Backwards
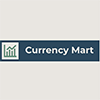
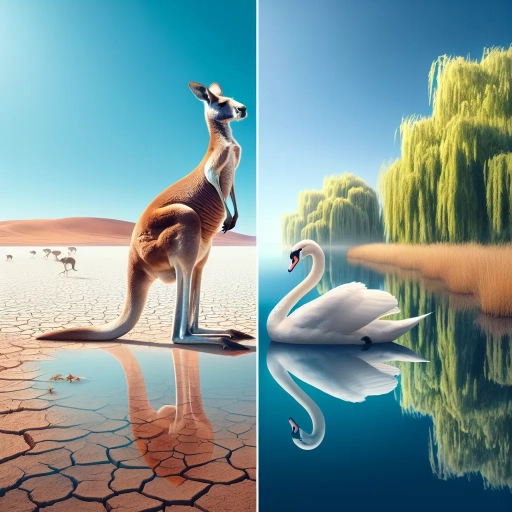
In the vast and diverse world of animals, each species has evolved unique adaptations to navigate their environments efficiently. One fascinating aspect of animal locomotion is the ability—or lack thereof—to move in different directions. Among the myriad creatures that inhabit our planet, there exists an animal that stands out for its inability to walk backwards. This intriguing characteristic is not merely a quirk but is deeply rooted in the animal's anatomy, physiology, and evolutionary history. To understand why this animal is confined to forward movement, we must delve into the unique aspects of its locomotion, explore the anatomical and physiological features that underpin this limitation, and examine the behavioral and evolutionary reasons that have shaped its movement patterns. In this article, we will begin by introducing the unique locomotion of animals, setting the stage for a deeper exploration of the specific animal in question and the reasons behind its forward-only movement. **Introduction to the Unique Locomotion of Animals**
Introduction to the Unique Locomotion of Animals
The unique locomotion of animals is a fascinating and complex aspect of their biology, offering insights into their evolution, adaptation, and survival strategies. This article delves into the diverse ways animals move, highlighting the intricate mechanisms and adaptations that enable them to thrive in various environments. We will begin by providing an **Overview of Animal Movement Patterns**, exploring the different types of locomotion such as walking, running, swimming, flying, and crawling, and how these movements are tailored to specific habitats. Understanding these patterns is crucial for appreciating the **Importance of Understanding Locomotion in Different Species**, as it reveals how locomotion influences behavior, social interactions, and overall fitness. Additionally, we will offer a **Preview of the Main Animal in Focus**, showcasing a particular species that exemplifies remarkable locomotor adaptations. By examining these aspects, we gain a deeper appreciation for the remarkable diversity and ingenuity of animal movement. Let us start by exploring the **Overview of Animal Movement Patterns** to understand the foundational principles behind this incredible array of locomotor strategies.
Overview of Animal Movement Patterns
Animal movement patterns are as diverse and fascinating as the creatures themselves, reflecting adaptations to various environments and survival strategies. From the majestic strides of giraffes to the agile leaps of kangaroos, each species has evolved unique locomotion techniques that enable them to navigate their habitats efficiently. One of the most intriguing aspects of animal movement is the directionality of their locomotion. While many animals can move in multiple directions, there are some that are incapable of walking backwards. This limitation is often a result of specific anatomical features or evolutionary pressures that prioritize forward movement. For instance, kangaroos and wallabies, members of the macropod family, are renowned for their powerful hind legs and specialized hopping mechanism. Their unique gait allows them to cover vast distances at high speeds, but their anatomy makes backward movement nearly impossible. The structure of their legs and the way they use their tail for balance during hopping render reverse locomotion impractical and energetically costly. Similarly, many species of birds, such as ostriches and emus, have powerful legs designed for running and kicking but lack the flexibility and musculature necessary for backward movement. In aquatic environments, fish like sharks and rays exhibit streamlined bodies optimized for forward propulsion through water. Their skeletal systems and muscle arrangements are tailored for efficient swimming in one direction, making reverse movement difficult or impossible without significant energy expenditure. Even among terrestrial animals like humans and other primates, while we can technically move backwards, it is not as natural or efficient as forward locomotion due to our bipedal stance and the structure of our lower limbs. Understanding these movement patterns not only highlights the incredible diversity of animal locomotion but also underscores the importance of adaptation in evolutionary biology. Each species' ability or inability to move in certain directions is a testament to how their environment and lifestyle have shaped their physical attributes over time. This insight into animal movement patterns serves as a foundation for exploring the unique locomotion strategies that have evolved across different species, each with its own set of advantages and limitations that define their place in the natural world. By examining these patterns, we gain a deeper appreciation for the intricate relationships between anatomy, environment, and behavior that make each animal uniquely suited to its ecological niche.
Importance of Understanding Locomotion in Different Species
Understanding locomotion in different species is a cornerstone of biological and ecological research, offering profound insights into the evolutionary adaptations, behavioral patterns, and survival strategies of various animals. Locomotion, the ability to move from one place to another, is a critical aspect of an organism's life, influencing its ability to find food, escape predators, and reproduce. Each species has evolved unique locomotor mechanisms tailored to its environment and lifestyle. For instance, the cheetah's slender body and powerful leg muscles enable it to achieve speeds of up to 70 miles per hour, making it the fastest land animal on Earth. In contrast, the octopus uses a complex system of muscles and hydrostatic pressure to change the shape of its body and move through water with remarkable agility. The study of locomotion also reveals intriguing differences in how various animals navigate their environments. While many animals can move in multiple directions, some exhibit specific limitations. For example, the kangaroo and the koala are among the few mammals that cannot walk backwards due to their specialized limb structures and muscle arrangements. Kangaroos rely on their powerful hind legs and tail for forward propulsion, while koalas have strong arms adapted for climbing and grasping but are not suited for backward movement. Understanding these unique locomotor abilities is essential for several reasons. Firstly, it helps in conserving species by identifying habitat requirements and potential threats. For example, knowing that certain species cannot move efficiently through fragmented habitats can inform conservation efforts aimed at preserving continuous corridors for movement. Secondly, studying locomotion can inspire technological innovations, such as robotics and prosthetics, by mimicking nature's solutions to movement challenges. Finally, it enhances our appreciation for the diversity and complexity of life on Earth, highlighting the remarkable adaptations that have evolved over millions of years to ensure the survival and success of different species. In conclusion, the importance of understanding locomotion in different species extends beyond mere curiosity; it underpins our understanding of ecological balance, informs conservation strategies, and drives technological advancements. By delving into the unique ways in which animals move, we gain a deeper appreciation for the intricate relationships between organisms and their environments, ultimately enriching our knowledge of the natural world. This understanding is crucial as we continue to explore and protect the diverse array of life on our planet.
Preview of the Main Animal in Focus
In the realm of animal locomotion, one creature stands out for its unique inability to walk backwards: the kangaroo. This marsupial, native to Australia, is renowned for its powerful hind legs and distinctive hopping gait. The kangaroo's locomotion is a marvel of evolutionary adaptation, optimized for speed and efficiency in its natural habitat. Unlike many other mammals that can move in various directions, the kangaroo's anatomy is specialized to the extent that it cannot walk backwards. This limitation is due to several key factors: its robust tail, which serves as a fifth limb for balance and propulsion; its elongated hind legs, designed for explosive jumping rather than reverse movement; and its unique foot structure, featuring large, elastic tendons that store energy for each bound but are not suited for backward motion. The kangaroo's inability to walk backwards is a trade-off for its extraordinary forward speed and agility. When threatened or during courtship displays, kangaroos can achieve remarkable velocities, often exceeding 30 miles per hour. This speed is crucial for survival in their environment, where predators like dingoes and eagles are ever-present threats. Despite the limitation of not being able to move in reverse, kangaroos have developed other strategies to cope with potential dangers. For instance, they can quickly change direction by using their powerful tail as a pivot point, allowing them to dodge predators with agility. Moreover, the kangaroo's unique locomotion has fascinated scientists and wildlife enthusiasts alike. Studies on kangaroo biomechanics have revealed intricate details about how these animals manage to cover such vast distances with minimal energy expenditure. The elastic properties of their tendons act like springs, enabling them to store and release energy efficiently with each hop. This mechanism not only enhances their speed but also reduces the metabolic cost of movement, making them highly efficient runners. In addition to their impressive physical abilities, kangaroos also exhibit complex social behaviors that are influenced by their locomotion. For example, dominant males engage in "boxing" matches where they stand upright on their hind legs and use their forepaws to spar for mating rights. This behavior highlights how their specialized locomotion extends beyond mere movement—it is integral to their social hierarchy and reproductive success. Understanding the kangaroo's unique locomotion provides valuable insights into evolutionary biology and biomechanics. It underscores how specific adaptations can lead to remarkable specializations that enhance an animal's survival and reproductive capabilities within its environment. As we delve deeper into the fascinating world of animal locomotion, the kangaroo serves as an exemplary model of how nature's ingenuity can result in extraordinary abilities that set certain species apart from others. By exploring these unique traits, we gain a deeper appreciation for the diversity and complexity of life on Earth.
The Anatomy and Physiology of the Animal in Question
Understanding the anatomy and physiology of an animal is crucial for appreciating its overall functionality and survival mechanisms. This intricate interplay between various bodily systems dictates how an animal moves, adapts, and thrives in its environment. The skeletal structure, for instance, provides the foundational framework that supports the animal's body and facilitates movement, but it also comes with inherent limitations that influence the animal's capabilities. Additionally, the muscular system, while essential for locomotion and other physical activities, is subject to specific constraints and adaptations that optimize performance within these limitations. Furthermore, the nervous system plays a pivotal role in coordinating movement, ensuring that the skeletal and muscular systems operate in harmony. By examining these components—skeletal structure limitations, muscular constraints and adaptations, and nervous system implications on movement—we can gain a comprehensive understanding of how an animal's anatomy and physiology work together to enable its unique behaviors and survival strategies. Let's begin by exploring the skeletal structure limitations that underpin these complex interactions.
Skeletal Structure Limitations
The skeletal structure of animals, while incredibly diverse and adaptable, is not without its limitations. One notable example of these limitations is seen in the anatomy of the horse. Horses, despite their impressive size and strength, are incapable of walking backwards due to the specific design of their skeletal system. This inability stems from the unique structure of their joints and the arrangement of their bones. In horses, the joints in their legs are designed for forward movement, with the knee and hock joints functioning similarly to hinges that allow for flexion and extension but not for backward motion. The equine knee, located above the hoof, is actually an equivalent to the human wrist, and it lacks the necessary flexibility to bend in a way that would allow the horse to move its legs backwards. Additionally, the horse's hock joint, which corresponds to the human ankle, is also restricted in its range of motion, further preventing any backward movement. Another critical factor is the horse's hoof anatomy. Hooves are made of a tough protein called keratin and are shaped to provide support and traction during forward locomotion. However, they do not offer the necessary grip or stability for backward movement, making it difficult for horses to generate the force needed to walk in reverse. Furthermore, the overall musculature and ligamentous support in a horse's legs are optimized for forward propulsion rather than backward movement. The powerful muscles in the hindquarters and the specialized ligaments that connect bones together are all geared towards efficient forward locomotion, contributing to the horse's inability to walk backwards. This limitation is not unique to horses; other quadruped mammals with similar skeletal adaptations also face similar constraints. For instance, cows and goats share some of these anatomical features that restrict their ability to move in reverse. However, each species has evolved specific traits that allow them to navigate their environments effectively within these constraints. Understanding these skeletal limitations provides valuable insights into the anatomy and physiology of animals like horses. It highlights how evolutionary pressures have shaped their bodies for specific functions, often at the expense of other capabilities. This knowledge is crucial for veterinarians, trainers, and anyone involved in the care and management of these animals, as it informs how they should be handled and exercised to avoid injury and ensure optimal health. In conclusion, the skeletal structure of horses and other similar quadruped mammals imposes significant limitations on their ability to walk backwards. These limitations are rooted in the specialized design of their joints, hooves, muscles, and ligaments, all of which are optimized for forward movement. Recognizing these limitations is essential for appreciating the intricate balance between form and function in animal anatomy and for providing appropriate care and management for these remarkable creatures.
Muscular Constraints and Adaptations
When examining the anatomy and physiology of animals, particularly those that cannot walk backwards, it is crucial to delve into the realm of muscular constraints and adaptations. These constraints often stem from evolutionary pressures that have shaped the musculoskeletal system to optimize specific functions, such as speed, agility, or stability. For instance, in the case of horses, their skeletal and muscular structures are highly specialized for forward movement. The horse's pelvis is designed to support powerful gluteal muscles that propel the animal forward, while the hindlimbs are structured to absorb shock and distribute force efficiently during locomotion. However, this specialization comes at a cost; the horse's hindlimbs lack the necessary flexibility and muscular arrangement to facilitate backward movement. The gastrocnemius and soleus muscles in the lower leg are primarily designed for extension and propulsion rather than flexion, making it difficult for horses to walk backwards without significant strain. Similarly, many quadruped mammals exhibit similar adaptations that limit their ability to move in reverse. In dogs, for example, the structure of their hip and knee joints, along with the arrangement of their muscles, is optimized for forward locomotion. The quadriceps and hamstrings work in tandem to extend and flex the knee joint efficiently during running or walking forward but are less effective when attempting to move backwards. This is further compounded by the dog's paw anatomy, which is better suited for gripping and pushing off rather than pulling back. Birds also present an interesting case study in muscular constraints and adaptations. Many bird species, such as chickens and turkeys, have powerful leg muscles that enable them to run swiftly forward but lack the necessary musculature to walk backwards. The arrangement of their legs and feet is optimized for forward propulsion and balance, with strong gastrocnemius muscles facilitating rapid extension of the ankle joint. However, these birds often rely on their wings for balance when moving in reverse, highlighting how different muscle groups can compensate for limitations in specific movements. In addition to these examples, understanding muscular constraints can provide insights into the evolutionary history of an animal. For instance, the kangaroo's unique hopping gait is supported by highly specialized leg muscles that allow for powerful extension but restrict backward movement. This adaptation is likely a result of selective pressures favoring speed and efficiency over versatility in locomotion. In conclusion, the inability of certain animals to walk backwards is a direct result of their muscular constraints and adaptations. These constraints are often a trade-off for enhanced performance in other areas, such as speed or agility, and reflect the animal's evolutionary history and environmental pressures. By examining these adaptations closely, we gain a deeper understanding of how anatomy and physiology interact to define an animal's capabilities and limitations. This knowledge not only enriches our appreciation of animal diversity but also informs fields such as veterinary medicine, wildlife conservation, and biomechanical engineering.
Nervous System Implications on Movement
The nervous system plays a pivotal role in the control and coordination of movement in animals, and its implications are particularly evident when examining species that exhibit unique locomotor abilities. For instance, the anatomy and physiology of the animal in question—such as the kangaroo—highlight how specialized nervous system structures enable or limit certain types of movement. Kangaroos, known for their powerful hind legs and distinctive hopping gait, are incapable of walking backwards due to several key nervous system adaptations. At the core of this limitation is the integration of sensory input, motor control, and reflex mechanisms. The kangaroo's nervous system is highly specialized to optimize forward movement through hopping. This involves precise coordination between the central nervous system (CNS), peripheral nervous system (PNS), and the musculoskeletal system. The CNS, comprising the brain and spinal cord, processes sensory information from proprioceptors (sensors in muscles and tendons) and mechanoreceptors (sensors in skin and joints) to adjust muscle tension and timing for efficient hopping. The PNS, which includes motor neurons and sensory neurons, transmits these signals to and from the CNS, ensuring synchronized muscle contractions. However, this specialization comes at a cost; the neural pathways and muscle groups are not adapted for reverse movement. The unique arrangement of muscles in a kangaroo's legs, particularly the strong gastrocnemius and soleus muscles in the hind legs, are optimized for forward propulsion rather than backward motion. Moreover, reflexes play a crucial role in maintaining balance and direction during hopping. These reflexes are programmed to respond to specific stimuli that occur during forward movement, such as the impact of landing on the feet or the stretch in the Achilles tendon. When attempting to move backwards, these reflexes would be inappropriate and could lead to instability or injury. Additionally, the brain's motor control centers have learned patterns of movement through experience and practice, which are deeply ingrained in the kangaroo's motor memory. These patterns are highly efficient for forward hopping but do not include the necessary neural pathways for backward walking. This lack of neural plasticity in this specific context means that even if a kangaroo were to attempt walking backwards, it would face significant challenges in coordinating its muscles effectively. In summary, the nervous system's implications on movement in animals like kangaroos are profound. The specialized neural mechanisms that enable their unique hopping ability also restrict their capacity for backward movement. This interplay between anatomy, physiology, and nervous system function underscores why certain animals are adapted for specific types of locomotion and highlights the intricate complexity of motor control in the natural world.
Behavioral and Evolutionary Reasons for Forward Movement
Forward movement is a fundamental aspect of life, driven by a complex interplay of behavioral and evolutionary factors. At its core, the ability to move forward is crucial for survival, efficiency, and adaptation. This article delves into three key reasons why forward movement has become a cornerstone of many species' survival strategies. First, **Survival and Predation Avoidance Strategies** highlight how the ability to move forward allows organisms to escape predators, find safe habitats, and secure vital resources. Second, **Efficiency in Foraging and Resource Gathering** explains how forward movement enhances the ability to locate and acquire food, water, and other essential resources with greater speed and precision. Lastly, **Evolutionary Pressures Shaping Locomotion Traits** explores how natural selection has shaped the development of locomotion traits over time, favoring those that can move efficiently and effectively. By understanding these drivers, we gain insight into why forward movement has evolved as a critical component of life. Let us begin by examining the critical role of **Survival and Predation Avoidance Strategies** in the evolution of forward movement.
Survival and Predation Avoidance Strategies
In the intricate dance of survival, animals have evolved a myriad of strategies to avoid predation, each tailored to their unique environments and physiological capabilities. For many species, forward movement is not just a mode of locomotion but a critical component of their survival toolkit. The inability to walk backwards, a trait shared by several animal groups, underscores the importance of forward movement in predation avoidance. One of the primary reasons animals cannot walk backwards is the structural and functional specialization of their limbs. For instance, horses and other ungulates have hooves that are designed for forward propulsion, providing stability and speed when fleeing from predators. Their skeletal system, particularly the arrangement of their joints and muscles, is optimized for forward motion, making backward movement inefficient and potentially hazardous. Similarly, many birds have powerful legs adapted for running or perching but lack the flexibility to move in reverse. Forward movement also plays a crucial role in sensory awareness and reaction time. Animals that are adept at moving forward often possess keen senses that allow them to detect potential threats early. For example, gazelles have exceptional vision and hearing, enabling them to spot predators from a distance and respond swiftly by running forward. This forward-oriented sensory capability is essential for their survival, as it allows them to react quickly to danger without the need to turn around. Moreover, behavioral adaptations further reinforce the necessity of forward movement. Many prey species exhibit "flight responses" where they rapidly move away from perceived threats. This immediate reaction is often more effective when executed in a forward direction, as it leverages the animal's natural speed and agility. For instance, rabbits use their powerful hind legs to burst into high-speed runs when threatened, a strategy that relies on their ability to move swiftly in one direction—forward. In addition to these physical and behavioral adaptations, evolutionary pressures have also shaped the importance of forward movement in predation avoidance. Over generations, animals that could move efficiently in one direction—forward—were more likely to survive and reproduce, passing on their advantageous traits to their offspring. This selective pressure has led to the development of specialized features such as streamlined bodies in fish and cheetahs' slender builds, both optimized for rapid forward movement. In conclusion, the inability of certain animals to walk backwards is a testament to the evolutionary significance of forward movement in survival and predation avoidance. From structural adaptations like hooves and powerful legs to behavioral responses such as flight reactions, these strategies highlight how forward motion has become an indispensable aspect of many species' survival kits. As we explore why some animals cannot walk backwards, we uncover a deeper narrative about how nature has honed specific traits to ensure survival in a world filled with predators and dangers lurking around every corner.
Efficiency in Foraging and Resource Gathering
Efficiency in foraging and resource gathering is a critical aspect of an animal's survival, particularly when considering the evolutionary pressures that shape their behaviors. For many species, the ability to optimize resource acquisition is directly linked to their fitness and reproductive success. In the context of animals that cannot walk backwards, such as kangaroos and certain species of birds, this efficiency is often achieved through specialized adaptations that enhance forward movement. For instance, kangaroos have evolved powerful hind legs and a unique hopping mechanism that allows them to cover vast distances with minimal energy expenditure. This locomotor strategy is highly efficient for foraging over large areas, enabling them to locate and exploit food sources quickly. Similarly, birds like penguins use their streamlined bodies and flippers to swim efficiently through water, allowing them to hunt fish and other aquatic prey with precision. These adaptations not only facilitate rapid movement but also reduce the metabolic costs associated with foraging, thereby increasing the net energy gain from each foraging bout. Moreover, animals that cannot walk backwards often develop keen senses and cognitive abilities to compensate for their limited mobility. For example, some species of birds have exceptional vision that helps them detect food from a distance, reducing the need for extensive searching. This visual acuity, combined with their ability to fly or move swiftly in one direction, makes them highly efficient foragers despite their inability to reverse direction on foot. Additionally, behavioral strategies play a significant role in enhancing foraging efficiency. Many animals that cannot walk backwards exhibit complex social behaviors that facilitate cooperative foraging. For example, some bird species engage in flocking behaviors where individuals take turns scouting for food while others rest or engage in other activities. This division of labor ensures that resources are located and exploited efficiently without overexerting any single individual. From an evolutionary perspective, the inability to walk backwards has driven the development of these specialized traits and behaviors. Natural selection favors individuals that can optimize their resource gathering activities within the constraints of their physical capabilities. Over time, this selective pressure has led to the evolution of highly efficient foraging strategies tailored to the specific ecological niches occupied by these animals. In summary, animals that cannot walk backwards have evolved remarkable adaptations and behaviors to ensure efficient foraging and resource gathering. These strategies, ranging from specialized locomotion and sensory capabilities to complex social behaviors, underscore the intricate relationship between behavioral traits and evolutionary pressures. By optimizing their forward movement and compensating for limitations in mobility, these animals have successfully adapted to their environments, highlighting the remarkable diversity and resilience of life on Earth.
Evolutionary Pressures Shaping Locomotion Traits
Evolutionary pressures have played a pivotal role in shaping locomotion traits across various species, influencing how animals move and interact with their environments. The ability to walk forward is a common trait among many animals, but there are notable exceptions where backward movement is either highly restricted or entirely absent. One such example is the kangaroo, which, despite its impressive jumping and forward locomotion capabilities, is incapable of walking backwards. This limitation is largely due to the unique anatomy of kangaroos; their powerful hind legs and specialized foot structure are optimized for forward propulsion and jumping, making backward movement biomechanically impractical. The evolutionary pressures driving this adaptation are multifaceted. In the case of kangaroos, the need for efficient long-distance travel and rapid escape from predators in their open, grassland habitats has favored the development of powerful hind limbs and a robust tail that aids in balance and propulsion. The energy efficiency of their gait, characterized by elastic energy storage in their tendons and muscles, allows them to cover vast distances with minimal energy expenditure. However, this specialization comes at the cost of flexibility in movement direction; the rigid structure of their legs and the way they distribute their body weight make backward walking an energetically costly and mechanically challenging task. Similar evolutionary trade-offs can be observed in other species. For instance, the giraffe's long neck and legs are adapted for reaching high branches and running at high speeds over long distances, respectively. While these traits confer significant advantages in terms of feeding and predator avoidance, they also limit the giraffe's ability to maneuver in tight spaces or move in reverse efficiently. The giraffe's body plan is optimized for forward movement, reflecting the selective pressures of its savannah and woodland habitats where speed and reach are crucial survival attributes. In addition to anatomical constraints, behavioral factors also influence the evolution of locomotion traits. Many animals that cannot walk backwards often exhibit compensatory behaviors that mitigate this limitation. For example, kangaroos use their strong tails to pivot and change direction quickly when faced with obstacles or threats from behind. This behavioral adaptation underscores how evolutionary pressures not only shape physical traits but also influence behavioral strategies that enhance survival and reproductive success. The study of evolutionary pressures shaping locomotion traits offers valuable insights into the intricate relationships between anatomy, behavior, and environment. By examining why certain species cannot walk backwards, we gain a deeper understanding of the selective forces that have molded their evolutionary histories. This knowledge highlights the remarkable diversity of locomotor strategies in the animal kingdom and underscores the importance of considering both anatomical and behavioral adaptations when exploring how animals navigate their environments. Ultimately, the inability of some animals to walk backwards serves as a compelling example of how evolutionary specialization can lead to remarkable efficiencies in certain contexts while imposing limitations in others.