What Color Is Pluto
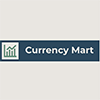
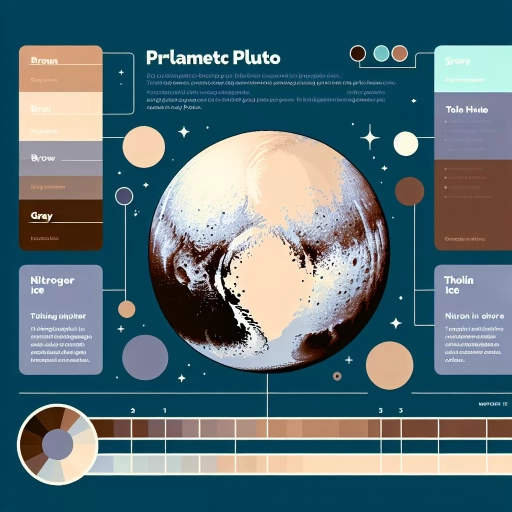
Understanding Pluto's Composition
Understanding Pluto's composition is a complex and multifaceted endeavor that involves several key aspects. At the heart of this inquiry lies the analysis of surface materials and their reflective properties, which provide crucial insights into the dwarf planet's geological history and current state. Additionally, the influence of Pluto's atmosphere on color perception plays a significant role in how we interpret its surface features, as atmospheric conditions can significantly alter the observed colors and textures. Furthermore, a comparative analysis with other celestial bodies, such as Neptune's moon Triton and other Kuiper Belt Objects (KBOs), helps contextualize Pluto's unique characteristics within the broader solar system. By examining these three interrelated factors, we can gain a more comprehensive understanding of Pluto's composition. Let us begin by delving into the surface materials and their reflective properties, which serve as the foundational layer for our exploration of this enigmatic world.
Surface Materials and Their Reflective Properties
When delving into the mysteries of Pluto's composition, understanding the reflective properties of surface materials becomes crucial. The reflectivity of a surface, often quantified by its albedo, is a key factor in determining how much sunlight is absorbed versus reflected. On Pluto, this is particularly important due to its distant and cold environment, where solar energy is scarce. The surface of Pluto is composed of various materials, each with distinct reflective properties. Water ice, for instance, has a high albedo and reflects a significant amount of sunlight, contributing to Pluto's overall brightness. Methane ice, another prevalent component, has a lower albedo compared to water ice but still plays a significant role in the planet's reflectivity. The presence of tholins—complex organic molecules formed through the interaction of methane and ultraviolet radiation—adds a darker, more absorptive layer to Pluto's surface, reducing its overall reflectivity. The combination and distribution of these materials across Pluto's surface influence its observed color and brightness. For example, regions like Sputnik Planum, a vast ice plain, appear bright due to the high concentration of water ice. In contrast, areas with higher tholin content, such as the Cthulhu Macula, appear darker and more reddish due to the absorptive nature of these organic compounds. Understanding these reflective properties not only helps in visualizing Pluto's surface but also provides insights into its geological history and ongoing processes. The New Horizons mission, which flew by Pluto in 2015, provided invaluable data on these surface materials and their reflective properties, enabling scientists to map Pluto's surface with unprecedented detail. By analyzing the reflectivity patterns across different regions, researchers can infer information about Pluto's composition, atmospheric interactions, and even its potential for hosting subsurface oceans or other geological features. This detailed understanding of surface materials and their reflective properties is essential for a comprehensive grasp of Pluto's composition and its place within our solar system.
Atmospheric Influence on Color Perception
The perception of color is significantly influenced by the atmospheric conditions of the environment in which an object is observed. This principle is crucial when attempting to understand the composition of distant celestial bodies like Pluto. The Earth's atmosphere, for instance, scatters shorter wavelengths of light, such as blue and violet, more than longer wavelengths like red and orange, a phenomenon known as Rayleigh scattering. This scattering effect is why our sky appears blue during the day. However, when observing objects in space, the absence of a substantial atmosphere around Pluto means that light is not scattered in the same way. Instead, the colors we observe are more direct reflections of the object's surface composition. When NASA's New Horizons spacecraft flew by Pluto in 2015, it captured detailed images that revealed a diverse range of colors on the dwarf planet's surface. The varying hues were indicative of different surface materials and processes. For example, the heart-shaped region known as Tombaugh Regio appears bright and white due to the presence of nitrogen ice, while other areas display darker, reddish tones likely resulting from tholins—complex organic molecules formed when methane and other simple organic compounds are exposed to ultraviolet radiation. These color variations provide valuable insights into Pluto's surface chemistry and geological history. The atmospheric influence on color perception also extends to how we interpret data from spacecraft. Since Pluto has a very thin atmosphere, known as an exosphere, it does not significantly alter the light reflected from its surface. This allows scientists to make more accurate assessments of Pluto's composition based on the reflected light spectra. In contrast, if Pluto had a thicker atmosphere similar to Earth's, the scattered light would complicate the analysis, making it harder to discern the true colors and compositions of its surface. Furthermore, understanding the atmospheric influence on color perception helps scientists correct for any biases introduced by Earth's own atmosphere when observing distant objects. By accounting for these effects, researchers can more accurately determine the intrinsic colors of celestial bodies like Pluto, thereby gaining a clearer picture of their composition and evolution. This meticulous approach ensures that the data collected from space missions are interpreted correctly, providing a reliable foundation for scientific conclusions about the nature of our solar system's outer reaches. In summary, the atmospheric influence on color perception plays a pivotal role in understanding Pluto's composition. The absence of a substantial atmosphere around Pluto allows for a more direct observation of its surface colors, which in turn reveals valuable information about its surface materials and geological processes. By considering these factors, scientists can make precise determinations about Pluto's composition, contributing significantly to our broader understanding of the solar system's diverse and fascinating worlds.
Comparative Analysis with Other Celestial Bodies
In the quest to understand Pluto's composition, a comparative analysis with other celestial bodies in our solar system provides invaluable insights. By examining the characteristics of similar objects, such as Neptune's moon Triton and the Kuiper Belt Objects (KBOs), we can better grasp the unique and shared features that define Pluto. Triton, for instance, shares a striking resemblance with Pluto in terms of its icy surface and possible subsurface ocean. Both bodies exhibit cryovolcanic activity, where water and other volatiles play a crucial role in their geological processes. This similarity suggests that Pluto might also harbor a subsurface ocean, which could have significant implications for its internal structure and potential habitability. The Kuiper Belt, a region of icy bodies beyond Neptune, is another key area for comparison. Pluto is one of the largest KBOs, and studying other members of this group helps in understanding the commonalities and differences within this population. For example, Eris, another large KBO, has a surface composed primarily of methane ice, which contrasts with Pluto's more diverse surface featuring methane, nitrogen, and carbon monoxide ices. This diversity highlights the varied chemical compositions within the Kuiper Belt and underscores the importance of detailed spectroscopic studies to determine the exact makeup of these distant worlds. Furthermore, comparing Pluto to other dwarf planets like Ceres in the asteroid belt and Haumea in the Kuiper Belt offers a broader perspective on the formation and evolution of these small, icy worlds. Ceres, with its water-rich composition and possible subsurface ocean, presents a different evolutionary path compared to Pluto's more volatile-rich surface. Haumea, known for its elongated shape and rapid rotation, provides an example of how gravitational forces can shape the morphology of these bodies. These comparisons not only enrich our understanding of Pluto but also contribute to a more comprehensive picture of the solar system's outer reaches. Additionally, the study of comets, which are thought to originate from the same region as KBOs, offers further clues about Pluto's composition. Comets like 67P/Churyumov-Gerasimenko, studied by the Rosetta mission, have revealed complex organic chemistry and water ice mixtures that are likely present in KBOs as well. This suggests that Pluto may also contain similar organic materials, which could have been delivered to Earth via cometary impacts. In conclusion, a comparative analysis with other celestial bodies is essential for a deeper understanding of Pluto's composition. By examining the similarities and differences between Pluto and other icy worlds, we gain a more nuanced view of its place within the solar system and the processes that have shaped it over billions of years. This approach not only enhances our knowledge of Pluto but also contributes to a broader understanding of the diverse and complex nature of our cosmic neighborhood.
Observational Evidence and Data
Observational evidence and data have been pivotal in shaping our understanding of the universe, particularly when it comes to distant and enigmatic celestial bodies like Pluto. This article delves into the multifaceted nature of observational evidence, highlighting three key areas that have significantly contributed to our current knowledge. First, we explore **Historical Observations and Early Theories**, which trace the evolution of human understanding from initial discoveries to early speculative theories. Next, we examine the groundbreaking findings from the **New Horizons Mission**, which provided unprecedented close-up data on Pluto's surface and atmosphere. Finally, we discuss the **Spectral Analysis of Pluto's Surface**, revealing insights into the composition and geological history of this dwarf planet. By combining these perspectives, we gain a comprehensive view of how observational evidence has transformed our understanding of Pluto from a mysterious point in the sky to a well-studied celestial neighbor. Let us begin by looking back at the historical roots of Pluto's discovery and the early theories that shaped our initial perceptions.
Historical Observations and Early Theories
Historical observations and early theories about Pluto have been a fascinating and evolving narrative, reflecting the advancements in astronomical technology and our understanding of the solar system. When Pluto was first discovered in 1930 by Clyde Tombaugh, it was initially considered to be the ninth planet in our solar system. The discovery was a significant event, as it seemed to complete the set of planets known at that time. However, early observations were limited by the technology available, and much of what was known about Pluto came from indirect methods such as orbital calculations and spectroscopic analysis. One of the earliest theories about Pluto's nature was that it was a large, icy body similar to Neptune and Uranus. This theory was supported by observations of its orbital pattern, which suggested that Pluto might be part of a larger population of similar objects beyond Neptune. The 1950s saw the development of more sophisticated telescopes and observational techniques, allowing astronomers to gather more detailed data on Pluto's size, mass, and composition. These observations led to the realization that Pluto was much smaller than initially thought and had a highly eccentric orbit that overlapped with the Kuiper Belt, a region of icy bodies and other small celestial objects beyond Neptune. The 1970s and 1980s brought significant advancements in space exploration technology, including the launch of spacecraft like Voyager 1 and 2, which provided new insights into the outer reaches of the solar system. Although these missions did not directly observe Pluto, they helped scientists better understand the environment in which Pluto existed. The discovery of other Kuiper Belt Objects (KBOs) in the 1990s further challenged the status of Pluto as a planet. One notable KBO, Eris, discovered in 2005, was found to be slightly larger than Pluto, prompting a reevaluation of what constitutes a planet. In 2006, the International Astronomical Union (IAU) redefined the term "planet," categorizing Pluto as a dwarf planet—a new class of objects created to describe Pluto and other similar bodies in our solar system. This decision was based on extensive observational evidence and data collected over decades. The New Horizons mission launched in 2006 provided the most detailed observations yet when it flew by Pluto in 2015. High-resolution images and detailed spectroscopic data revealed a complex geology with evidence of recent tectonic activity, suggesting that Pluto is more dynamic than previously thought. In summary, historical observations and early theories about Pluto have evolved significantly from its initial discovery as a potential ninth planet to its current classification as a dwarf planet. Each step in this journey has been driven by advances in technology and our ability to collect and analyze observational evidence. Today, we continue to refine our understanding of Pluto through ongoing research and missions, ensuring that our knowledge of this enigmatic world remains as vibrant and engaging as ever. This rich history underscores the importance of continuous observation and data collection in shaping our understanding of celestial bodies like Pluto.
New Horizons Mission Findings
The New Horizons mission, launched in 2006, has provided unprecedented insights into the Pluto system, revolutionizing our understanding of this distant and enigmatic world. As part of the Observational Evidence and Data, the findings from New Horizons are pivotal in answering long-standing questions about Pluto's composition, atmosphere, and geological activity. Upon its flyby in July 2015, New Horizons captured stunning images and gathered extensive data that revealed Pluto's surface to be far more dynamic than previously imagined. One of the most striking discoveries was the presence of a heart-shaped region known as Tombaugh Regio, which is composed primarily of nitrogen ice mixed with darker organic material. This region is surrounded by mountains of water ice, some reaching heights of over 11,000 feet, indicating recent geological activity that has reshaped Pluto's surface over millions of years. The mission also uncovered evidence of a thin atmosphere, which freezes and thaws as Pluto moves closer to and further from the Sun. This process creates a hazy atmosphere that scatters sunlight in a way that gives Pluto its reddish hue. The coloration is due to tholins, complex organic molecules formed when methane and other simple organic compounds are exposed to ultraviolet radiation. These tholins are responsible for the reddish-brown color observed on Pluto's surface. Furthermore, New Horizons revealed Pluto's moons in greater detail. Charon, the largest moon, was found to have its own unique features, including canyons and evidence of past volcanic activity. Other moons like Nix, Hydra, Kerberos, and Styx were also studied, providing insights into their orbits and possible origins. The data collected by New Horizons has significantly expanded our knowledge of the Kuiper Belt, a region of icy bodies beyond Neptune. Pluto's surface features suggest it may have experienced a period of rapid cooling early in its history, leading to the formation of its current icy landscape. Additionally, the mission's observations of Pluto's interaction with the solar wind have provided valuable information about how small, icy worlds interact with their environment. In summary, the New Horizons mission has transformed our understanding of Pluto from a mere point of light in the sky to a complex, dynamic world with its own unique geology, atmosphere, and moons. The observational evidence and data gathered during this mission have not only answered many questions but also opened up new avenues for research into the outer reaches of our solar system.
Spectral Analysis of Pluto's Surface
Spectral analysis of Pluto's surface has been a crucial tool in understanding the composition and characteristics of this distant dwarf planet. Through various observational missions, particularly the New Horizons flyby in 2015, scientists have gathered extensive data that shed light on Pluto's surface properties. Spectroscopy, which involves analyzing the light reflected or emitted by Pluto, allows researchers to identify the chemical makeup of its surface materials. The New Horizons spacecraft was equipped with instruments such as the Ralph spectrometer and the Linear Etalon Imaging Spectral Array (LEISA), which provided high-resolution spectral data across different wavelengths. These data revealed a diverse and complex surface composition. Methane and nitrogen ices are prevalent, with methane ice forming distinct tholins—complex organic molecules that result from the interaction of methane with ultraviolet radiation. Tholins are responsible for the reddish hue observed in some regions of Pluto's surface. Additionally, water ice and other frozen volatiles like carbon monoxide and ethane have been identified, indicating a dynamic geological history involving cryovolcanism and possible recent surface activity. The spectral signatures also suggest variations in surface texture and age. For instance, the Sputnik Planum ice plain shows a relatively young and smooth surface, indicative of recent resurfacing processes, while other areas exhibit more rugged terrains with older surfaces. These findings are supported by the observation of different albedo (reflectivity) patterns across Pluto's surface, which correlate with the presence of various ices and tholins. Furthermore, the spectral analysis has provided insights into Pluto's atmospheric composition. The presence of methane and nitrogen in the atmosphere affects the spectral lines observed, allowing scientists to study atmospheric escape and interaction with the solar wind. This information is crucial for understanding Pluto's climatic conditions and how they influence its surface evolution over time. In summary, spectral analysis of Pluto's surface has significantly enhanced our understanding of its composition, geological history, and atmospheric properties. By deciphering the spectral signatures from observational data, researchers can reconstruct a detailed picture of this enigmatic world, offering valuable insights into its place within our solar system. This evidence underscores the importance of continued exploration and study of Pluto and other Kuiper Belt Objects to unravel the mysteries of the outer solar system.
Scientific Interpretation and Theories
Scientific interpretation and theories play a crucial role in understanding the mysteries of our universe, particularly when it comes to distant celestial bodies like Pluto. The study of Pluto, once considered the ninth planet in our solar system, has unveiled a wealth of information that sheds light on its unique characteristics. This article delves into three key aspects that contribute to our understanding of Pluto: Tholins and Their Role in Pluto's Coloration, the Impact of Solar Radiation on Surface Chemistry, and Geological Processes Shaping Pluto's Appearance. Each of these elements provides a distinct lens through which we can analyze Pluto's composition and evolution. By examining the formation and effects of tholins, we gain insight into the chemical processes that influence Pluto's coloration. The impact of solar radiation on surface chemistry reveals how external forces shape the planet's surface over time. Meanwhile, geological processes highlight the dynamic nature of Pluto's landscape. These interconnected themes collectively enrich our scientific interpretation of Pluto, starting with the intriguing role that tholins play in its coloration.
Tholins and Their Role in Pluto's Coloration
Tholins, complex organic molecules formed through the interaction of ultraviolet radiation with simple organic compounds, play a pivotal role in the coloration of Pluto. These substances are generated when methane and other simple organic molecules present in Pluto's atmosphere are exposed to UV light from the Sun. The resulting tholins are rich in carbon, nitrogen, and oxygen, and they exhibit a range of colors from red to brown, which are responsible for the distinct hue observed on Pluto's surface. Scientific observations and theories suggest that tholins are not only limited to Pluto but are also found on other bodies in the outer Solar System, such as Neptune's moon Triton and Saturn's moon Titan. However, Pluto's unique environment makes it an ideal laboratory for studying these compounds. The New Horizons spacecraft, which flew by Pluto in 2015, provided invaluable data that helped scientists understand the distribution and impact of tholins on Pluto's surface. High-resolution images revealed extensive deposits of tholin-rich material, particularly in regions like the Sputnik Planum ice plain and the Norgay Montes mountain range. The formation of tholins is closely tied to Pluto's atmospheric chemistry. Methane and other volatile compounds freeze and thaw as Pluto orbits the Sun, creating a dynamic cycle that influences the production and deposition of tholins. These molecules can settle on the surface, contributing to the reddish-brown coloration observed. Additionally, tholins can be incorporated into Pluto's ices, affecting their optical properties and further enhancing the planet's color. Theoretical models and laboratory experiments support the idea that tholins are key to understanding Pluto's color. By simulating the conditions found on Pluto, scientists have been able to replicate the formation of tholins and study their optical properties. These studies indicate that tholins can absorb light in the blue and green parts of the spectrum while reflecting longer wavelengths, such as red and orange, which explains the observed coloration. Moreover, the presence of tholins on Pluto has broader implications for our understanding of the early Solar System. Tholins are thought to be precursors to more complex organic materials that could have played a role in the origins of life on Earth. Studying tholins on Pluto provides insights into the chemical processes that occurred in the early Solar System and how they might have influenced the development of life elsewhere. In summary, tholins are crucial for understanding Pluto's coloration due to their ability to absorb certain wavelengths of light and reflect others, resulting in the planet's distinctive red-brown hue. The study of tholins on Pluto not only enhances our knowledge of this distant world but also offers valuable insights into the chemistry and evolution of the outer Solar System, making it a fascinating area of ongoing scientific research and interpretation.
Impact of Solar Radiation on Surface Chemistry
The impact of solar radiation on surface chemistry is a pivotal aspect of understanding the dynamic interactions between celestial bodies and their environments. Solar radiation, comprising a broad spectrum of electromagnetic waves including ultraviolet (UV), visible light, and infrared (IR) radiation, plays a crucial role in shaping the chemical composition and physical properties of surfaces. On planetary bodies like Pluto, which is now classified as a dwarf planet, solar radiation influences the surface chemistry in several key ways. Firstly, UV radiation from the Sun can initiate photochemical reactions that alter the molecular structure of surface materials. For instance, methane and other volatile compounds present on Pluto's surface can undergo photolysis, breaking down into simpler molecules or forming new compounds. This process contributes to the formation of tholins, complex organic molecules that are responsible for the reddish hue observed on Pluto's surface. These tholins are not only aesthetically significant but also provide valuable insights into the chemical history and evolution of the dwarf planet. Secondly, solar radiation affects the temperature and atmospheric conditions of Pluto. The absorption of solar energy by surface materials leads to temperature fluctuations, which in turn influence the sublimation and condensation cycles of volatile compounds like nitrogen and methane. This dynamic process shapes the surface topography and composition, creating features such as cryovolcanoes and ice plains that are characteristic of Pluto's landscape. Furthermore, the interaction between solar radiation and Pluto's atmosphere is crucial for understanding its surface chemistry. The atmosphere, though tenuous, interacts with solar photons through processes like photodissociation and ionization. These interactions can lead to the formation of reactive species that further modify the surface chemistry by reacting with existing molecules or depositing new ones. In addition to these direct effects, solar radiation also has an indirect impact on Pluto's surface chemistry through its influence on the dwarf planet's orbital dynamics. Pluto's highly eccentric orbit means that it experiences significant variations in solar irradiance over its orbital period. These variations can lead to seasonal changes in surface temperature and atmospheric pressure, which in turn affect the chemical processes occurring on the surface. In conclusion, the impact of solar radiation on Pluto's surface chemistry is multifaceted and profound. It drives photochemical reactions, influences temperature and atmospheric conditions, shapes the surface topography, and affects orbital dynamics. Understanding these interactions is essential for interpreting the scientific data collected from missions like New Horizons and for developing comprehensive theories about the evolution and current state of Pluto's surface chemistry. This knowledge not only enhances our understanding of Pluto but also provides valuable insights into the broader context of solar system chemistry and the potential for life beyond Earth.
Geological Processes Shaping Pluto's Appearance
Geological processes have significantly shaped Pluto's appearance, transforming it into the complex and intriguing world we observe today. One of the primary forces behind Pluto's geological evolution is cryovolcanism, a process where volatile compounds such as water, ammonia, and methane freeze and thaw, leading to volcanic-like activity. This phenomenon is evident in features like Wright Mons and Piccard Mons, which are thought to be cryovolcanoes that have erupted with icy materials rather than molten lava. These structures suggest that Pluto has experienced periods of internal heat, possibly due to tidal heating caused by its orbital interactions with its moon Charon. Another key process is the formation of tholins, complex organic molecules that result from the interaction of methane and other simple organic compounds with ultraviolet radiation. These tholins are responsible for Pluto's reddish hue and are found in its atmosphere and on its surface. The distribution of tholins varies across different regions, indicating varying levels of exposure to solar radiation and atmospheric conditions. Pluto's surface also exhibits extensive evidence of glacial activity. The Sputnik Planum ice plain, for instance, is a vast, nitrogen-ice glacier that shows signs of recent flow and resurfacing. This region is remarkably young and smooth, suggesting that it has been geologically active in the recent past. The presence of these ice plains and their dynamic nature highlight Pluto's ongoing geological processes, which are driven by the freezing and thawing of volatile ices. Impact cratering has also played a crucial role in shaping Pluto's landscape. The absence of large impact craters in certain areas, such as the Sputnik Planum, indicates that these regions have been resurfaced relatively recently. In contrast, other areas like the Norgay Montes mountain range show numerous craters, suggesting older surfaces that have been preserved over time. This variability in crater density provides valuable insights into Pluto's geological history and the timing of its surface processes. Additionally, Pluto's unique orbital characteristics contribute to its geological activity. Its highly eccentric orbit takes it as close as 29.7 astronomical units (AU) from the Sun and as far as 49.3 AU, leading to significant variations in temperature and solar radiation. These changes likely influence the freezing and thawing cycles of its surface ices, driving seasonal geological processes that shape its appearance over time. In summary, Pluto's appearance is a result of a combination of cryovolcanic activity, tholin formation, glacial processes, impact cratering, and orbital variations. These geological processes not only provide a window into Pluto's past but also highlight its dynamic and evolving nature, making it a fascinating subject for scientific study and interpretation. Understanding these processes helps us better appreciate the complex interplay of factors that have shaped this distant, icy world into the intriguing entity we see today.