What Is A Resonator
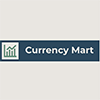
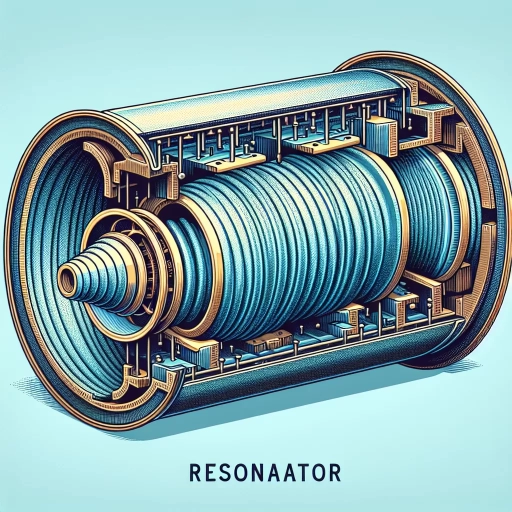
A resonator is a fundamental component in various fields, including physics, engineering, and technology, known for its ability to amplify or enhance specific frequencies. At its core, a resonator operates by storing energy in a way that maximizes the efficiency of energy transfer at particular frequencies. To fully grasp the concept of a resonator, it is essential to delve into its basic principles, understand how it functions, and explore its diverse applications. In this article, we will first **Understanding the Basics of a Resonator**, where we will define what a resonator is and the key characteristics that distinguish it. Next, we will examine **How Resonators Work**, detailing the mechanisms behind their operation and the physics that govern their behavior. Finally, we will discuss **Applications and Uses of Resonators**, highlighting their importance in fields such as electronics, acoustics, and medical technology. By understanding these aspects, readers will gain a comprehensive insight into the role and significance of resonators in modern technology. Let's begin by **Understanding the Basics of a Resonator**.
Understanding the Basics of a Resonator
Understanding the basics of a resonator is crucial for grasping its fundamental role in various technological and scientific applications. A resonator, at its core, is a device or system that vibrates or oscillates at specific frequencies, often used to amplify or filter signals. To delve into the intricacies of resonators, it is essential to explore three key aspects: their definition and purpose, the different types that exist, and their historical development. Firstly, understanding the definition and purpose of a resonator sets the foundation for appreciating its functionality. This involves recognizing how resonators are designed to enhance or select specific frequencies, making them indispensable in fields such as electronics, acoustics, and optics. Secondly, examining the various types of resonators reveals the diversity and adaptability of these devices. From mechanical resonators like pendulums and springs to electrical resonators such as LC circuits, each type has unique characteristics that suit different applications. Lastly, tracing the historical development of resonators provides insight into how these devices have evolved over time, influenced by technological advancements and scientific discoveries. By starting with a clear definition and purpose, we can better comprehend the multifaceted nature of resonators and their significance across various disciplines. Let us begin by exploring the definition and purpose of resonators in more detail.
Definition and Purpose
A resonator is a device or system that exhibits resonance, which is the tendency to oscillate at a specific frequency, often in response to an external force. The **definition** of a resonator can be understood as any object or structure that vibrates or oscillates at a particular frequency, typically when it is subjected to an external energy source. This frequency is known as the resonant frequency and is determined by the physical properties of the resonator, such as its mass, stiffness, and dimensions. The **purpose** of a resonator is multifaceted and varies depending on its application. In general, resonators are used to amplify or filter signals at specific frequencies. For instance, in electronics, resonant circuits (LC circuits) consisting of inductors and capacitors are used to tune into specific radio frequencies, allowing for precise signal reception and transmission. In mechanical systems, resonators can be used to enhance the efficiency of energy transfer; for example, in musical instruments like guitars and violins, the body of the instrument acts as a resonator to amplify sound waves produced by the strings. In engineering and physics, resonators play a crucial role in various fields such as telecommunications, medical imaging, and materials science. For example, in MRI machines, resonant coils are used to generate strong magnetic fields that resonate with hydrogen nuclei in the body, producing detailed images. Similarly, in materials science, resonant techniques like ultrasonic testing are employed to inspect the integrity of materials by detecting changes in their resonant frequencies. Understanding the basics of a resonator is essential for designing and optimizing systems that rely on resonance. By knowing how to calculate and adjust the resonant frequency of a system, engineers can create more efficient and effective devices. For instance, in the design of filters and antennas, precise control over resonant frequencies allows for better signal processing and transmission. Additionally, recognizing potential issues related to resonance, such as unwanted vibrations or resonance-induced failures, is critical for ensuring the reliability and safety of mechanical and electrical systems. In summary, resonators are fundamental components in many technological applications due to their ability to selectively amplify or filter signals at specific frequencies. Their purpose spans across various disciplines, from enhancing signal quality in electronics to improving diagnostic capabilities in medical imaging. Understanding the definition and purpose of resonators provides a solid foundation for exploring their diverse applications and optimizing their performance in different contexts.
Types of Resonators
Resonators are fundamental components in various fields such as electronics, mechanics, and acoustics, each designed to amplify or filter specific frequencies. There are several types of resonators, each with unique characteristics and applications. **Mechanical Resonators**: These operate based on the principle of mechanical vibration. Examples include tuning forks, pendulums, and spring-mass systems. Mechanical resonators are used in clocks, watches, and other timing devices due to their precise frequency response. **Electrical Resonators**: These are crucial in electronic circuits and can be categorized into two main types: LC (inductor-capacitor) resonators and crystal resonators. LC resonators consist of an inductor and a capacitor connected in series or parallel, creating a circuit that oscillates at a specific frequency. Crystal resonators use piezoelectric materials like quartz crystals to generate precise frequencies and are commonly used in electronic devices such as radios, computers, and mobile phones. **Acoustic Resonators**: These are designed to enhance sound waves at particular frequencies. Examples include musical instruments like guitars and violins, where the body of the instrument acts as a resonator to amplify sound. Another example is the Helmholtz resonator, which is used in soundproofing materials to absorb specific sound frequencies. **Optical Resonators**: These are used in laser technology and optical communication systems. Optical cavities, such as Fabry-Pérot cavities and ring resonators, confine light within a small space, allowing it to bounce back and forth and amplify or filter light at specific wavelengths. **Nuclear Resonators**: These involve the interaction of nuclear magnetic moments with external magnetic fields. Nuclear magnetic resonance (NMR) is a technique used in chemistry and medicine to analyze the structure of molecules and diagnose diseases. Each type of resonator has its own set of applications and benefits. Understanding the basics of these different types is essential for designing and utilizing resonators effectively in various technological and scientific contexts. By leveraging the unique properties of each type, engineers and scientists can create systems that operate with high precision and efficiency.
Historical Development
The historical development of resonators is a rich and multifaceted narrative that spans centuries, reflecting the evolution of scientific understanding and technological innovation. The concept of resonance itself dates back to ancient times, with early observations of vibrating strings and tuning forks. However, the modern understanding and application of resonators began to take shape during the Renaissance period. In the 17th century, scientists like Galileo Galilei and Christiaan Huygens laid foundational work on the principles of vibration and oscillation. Their studies on pendulums and springs provided the groundwork for later researchers. The 18th century saw significant contributions from Leonhard Euler, who mathematically described the behavior of vibrating systems, and Joseph-Louis Lagrange, who further developed these theories. The 19th century marked a pivotal era in the development of resonators. Hermann von Helmholtz's work on acoustics led to a deeper understanding of sound waves and their resonance properties. This period also saw the invention of the first practical resonators, such as the Helmholtz resonator, which is still used today in various applications including architectural acoustics and automotive design. The early 20th century witnessed the advent of electrical resonators with the development of radio technology. Guglielmo Marconi's experiments with radio waves relied heavily on tuned circuits that acted as resonators to filter and amplify signals. The invention of the quartz crystal resonator by Joseph W. Horton in 1920 revolutionized timekeeping and communication technologies by providing highly stable frequency sources. In the mid-20th century, advancements in materials science and electronics led to the creation of more sophisticated resonators. The development of piezoelectric materials enabled the construction of piezoelectric resonators, which are widely used today in devices ranging from ultrasonic cleaners to medical imaging equipment. Modern resonators continue to evolve with advancements in nanotechnology and microelectromechanical systems (MEMS). These innovations have enabled the miniaturization of resonators, making them integral components in modern electronics such as smartphones, GPS devices, and medical implants. The ongoing research into new materials and designs promises even more precise and versatile applications for resonators in the future. Understanding these historical developments is crucial for appreciating the fundamental principles behind how resonators work. From their early beginnings in mechanical systems to their current forms in advanced electronics, resonators have played a pivotal role in shaping our technological landscape. This historical context provides a solid foundation for delving into the basics of resonators and their diverse applications across various fields.
How Resonators Work
Resonators are fundamental components in various technological and scientific applications, from simple musical instruments to complex electronic circuits. Understanding how resonators work involves delving into three key concepts: the principle of resonance, energy transfer mechanisms, and frequency tuning and control. At the heart of any resonator is the principle of resonance, which dictates how the system vibrates or oscillates at specific frequencies. This principle is crucial because it explains why certain frequencies are amplified while others are dampened. Additionally, energy transfer mechanisms play a vital role in how resonators function, as they determine how energy is absorbed, stored, and released within the system. Finally, frequency tuning and control are essential for optimizing the performance of resonators, allowing them to operate efficiently at desired frequencies. By grasping these concepts, one can appreciate the intricate dynamics behind resonators and their widespread utility. Let's begin by exploring the principle of resonance, which forms the foundation of all resonator functionality.
Principle of Resonance
The Principle of Resonance is a fundamental concept that underpins the operation of resonators, which are devices designed to amplify or filter specific frequencies. Resonance occurs when a system vibrates or oscillates at a particular frequency, known as its resonant frequency, in response to an external force. This phenomenon is observed in various physical systems, including mechanical, electrical, and acoustic systems. In the context of resonators, resonance is harnessed to enhance the amplitude of vibrations or oscillations at the resonant frequency, while dampening other frequencies. For instance, in mechanical resonators like pendulums or springs, the system naturally oscillates at its resonant frequency due to the balance between the restoring force (such as gravity or spring tension) and the inertia of the moving mass. When an external force is applied at this frequency, the amplitude of oscillation increases significantly because the energy from the external force is efficiently transferred to the system. In electrical resonators, such as LC circuits (comprising inductors and capacitors), resonance occurs when the reactance of the inductor equals the reactance of the capacitor. At this point, the circuit's impedance is minimized, allowing maximum current flow and energy transfer. This principle is crucial in applications like radio tuning circuits where specific frequencies need to be selectively amplified. Acoustic resonators, such as musical instruments or cavities, also rely on the principle of resonance. For example, a guitar string vibrates at its resonant frequency when plucked, producing a clear tone. Similarly, a cavity like a pipe can resonate acoustically when sound waves of a particular frequency are introduced, leading to enhanced sound production. The key characteristics of resonance include selectivity and amplification. Selectivity refers to the ability of a resonator to respond strongly to a specific frequency while rejecting others. Amplification occurs because the energy input at the resonant frequency is efficiently stored and released by the system, leading to increased amplitude. Understanding and applying the Principle of Resonance is essential for designing and optimizing resonators across various fields. It allows engineers to create devices that can filter out unwanted frequencies, amplify desired signals, and improve overall system efficiency. Whether in mechanical engineering, electrical engineering, or acoustics, the principle of resonance remains a cornerstone for achieving precise frequency selection and amplification.
Energy Transfer Mechanisms
Energy transfer mechanisms are fundamental to understanding how resonators work, as they facilitate the efficient exchange of energy between different systems. In the context of resonators, energy transfer occurs through various mechanisms that leverage the principles of resonance to enhance efficiency and precision. 1. **Electromagnetic Coupling**: This mechanism involves the transfer of energy between two or more resonant circuits through electromagnetic fields. When two resonant circuits are brought close to each other, their magnetic fields interact, allowing energy to be transferred from one circuit to another. This is a key principle in wireless power transfer technologies and radio frequency (RF) communication systems. 2. **Mechanical Coupling**: In mechanical resonators, energy is transferred through physical contact or proximity. For example, in a mechanical watch, the balance wheel and spring system transfer energy mechanically to drive the watch's hands. Similarly, in acoustic resonators like musical instruments, mechanical vibrations are transferred through strings, membranes, or air columns. 3. **Thermal Energy Transfer**: This mechanism involves the transfer of thermal energy between systems at different temperatures. In thermal resonators, such as those used in thermoelectric devices, heat is transferred to generate electrical energy or vice versa. This process relies on the Seebeck effect or Peltier effect, where temperature differences drive electron flow. 4. **Quantum Mechanical Coupling**: At the quantum level, energy transfer can occur through mechanisms like quantum entanglement and tunneling. In quantum resonators, such as those used in quantum computing and quantum communication, energy is transferred between quantum states with high precision and speed. 5. **Piezoelectric Coupling**: This mechanism leverages the piezoelectric effect, where mechanical stress induces an electric charge in certain materials. In piezoelectric resonators, mechanical vibrations are converted into electrical signals and vice versa, making them crucial in applications like ultrasonic devices and sensors. 6. **Optical Coupling**: Optical resonators, such as lasers and optical cavities, transfer energy through light. Here, photons are confined within a cavity to enhance interaction with the gain medium, leading to coherent light emission. This mechanism is essential for high-precision spectroscopy and communication systems. Each of these energy transfer mechanisms exploits the resonant properties of systems to maximize efficiency and minimize losses. By understanding these mechanisms, engineers can design resonators that operate optimally across various domains, from electrical engineering to quantum physics, ensuring precise and efficient energy transfer in a wide range of applications.
Frequency Tuning and Control
Frequency tuning and control are crucial aspects of resonator operation, as they determine the precise frequency at which the resonator oscillates. A resonator, by definition, is a device that vibrates or oscillates at a specific frequency, often referred to as its resonant frequency. This frequency is inherently tied to the physical properties of the resonator, such as its mass, stiffness, and dimensions in mechanical systems, or capacitance and inductance in electrical circuits. To achieve precise frequency tuning, various methods can be employed. In mechanical resonators, adjustments can be made to the physical dimensions or material properties. For instance, in a guitar string, tightening or loosening the string changes its tension, thereby altering its resonant frequency. Similarly, in crystal oscillators used in electronic devices, slight variations in temperature can affect the crystal's dimensions and thus its resonant frequency, necessitating temperature compensation mechanisms. In electrical resonators, such as LC circuits (comprising inductors and capacitors), frequency tuning is typically achieved by adjusting either the capacitance or inductance. This can be done using variable capacitors or inductors, which allow for fine-tuning of the circuit's resonant frequency. For example, in radio transmitters and receivers, tuning dials adjust the capacitance of a variable capacitor to match the desired broadcast frequency. Control over the resonant frequency is also essential for maintaining stability and accuracy. Feedback mechanisms are often used to ensure that the resonator operates at its intended frequency despite external disturbances. In quartz crystal oscillators, for example, a feedback loop involving an amplifier and a crystal ensures that the oscillator maintains its precise frequency even under varying conditions. Moreover, modern resonators often incorporate advanced control systems to enhance their performance. Digital signal processing techniques can be used to monitor and adjust the resonant frequency in real-time, compensating for any drift due to environmental changes or aging of components. This level of control is particularly important in applications requiring high precision, such as GPS systems and atomic clocks. In summary, frequency tuning and control are vital components of resonator design and operation. By understanding and manipulating the physical and electrical properties of resonators, engineers can achieve precise and stable operation at specific frequencies, which is essential for a wide range of technological applications. This precision ensures that devices function reliably and accurately, whether in simple mechanical systems or complex electronic circuits.
Applications and Uses of Resonators
Resonators, devices that amplify or filter specific frequencies, play a crucial role in various technological fields. Their applications span across electrical and electronic systems, acoustic and mechanical systems, and advanced technologies and innovations. In electrical and electronic systems, resonators are essential for signal processing, filtering, and amplification, ensuring efficient communication and data transmission. They are also vital in acoustic and mechanical systems, where they enhance sound quality, stabilize vibrations, and optimize energy transfer. Furthermore, resonators drive advancements in cutting-edge technologies such as quantum computing, medical imaging, and aerospace engineering. By understanding the diverse applications of resonators, we can appreciate their significance in modern technology. This article will delve into these areas, starting with the critical role of resonators in electrical and electronic systems.
Electrical and Electronic Systems
Electrical and electronic systems are fundamental components in modern technology, playing a crucial role in various applications across different industries. These systems involve the design, development, and operation of circuits and devices that process, transmit, and utilize electrical energy. In the context of resonators, electrical and electronic systems are particularly significant because resonators are used to filter, amplify, or generate signals at specific frequencies. Resonators, which can be mechanical, electrical, or optical, are essential in many electrical and electronic systems due to their ability to enhance signal quality and efficiency. For instance, in radio communication systems, resonant circuits are used to tune into specific frequencies, allowing for clear reception of signals. Similarly, in power transmission lines, resonant circuits help in filtering out unwanted frequencies and ensuring stable power delivery. In electronic devices such as smartphones and computers, resonators are integral to the functioning of the circuitry. They are used in oscillators to generate clock signals that synchronize the operations of various components within the device. Additionally, resonant circuits in these devices help in noise reduction and signal conditioning, ensuring reliable data transmission and processing. The applications of resonators extend beyond consumer electronics to industrial and medical fields. In medical imaging technologies like MRI (Magnetic Resonance Imaging), resonant coils are used to generate strong magnetic fields that produce detailed images of the body. In industrial automation, resonant sensors are employed for precise measurement and control, enhancing the efficiency and accuracy of manufacturing processes. Moreover, resonators are crucial in energy storage and conversion systems. For example, in power supplies and inverters, resonant circuits are used to improve the efficiency of energy transfer and reduce losses. This is particularly important in renewable energy systems where maximizing efficiency is critical for sustainable power generation. In summary, electrical and electronic systems rely heavily on resonators to achieve optimal performance. Whether it is filtering signals in communication systems, generating clock signals in electronic devices, or enhancing medical imaging technologies, resonators play a vital role in ensuring the reliability and efficiency of these systems. Their ability to operate at specific frequencies makes them indispensable components in a wide range of applications, from consumer electronics to industrial and medical technologies.
Acoustic and Mechanical Systems
Acoustic and mechanical systems are integral components in the broader context of resonators, playing crucial roles in various applications across different fields. In acoustic systems, resonators are used to enhance or manipulate sound waves. For instance, musical instruments such as guitars and violins rely on resonant cavities to amplify and shape the sound produced by their strings. Similarly, in public address systems, resonant enclosures are employed to improve the efficiency and quality of sound reproduction. These acoustic resonators exploit the principle of resonance to amplify specific frequencies, thereby enhancing the overall auditory experience. In mechanical systems, resonators are utilized to optimize performance and efficiency. For example, in automotive engineering, resonators are used in exhaust systems to reduce noise levels and improve engine performance by tuning the exhaust system to resonate at frequencies that cancel out unwanted sounds. Additionally, mechanical resonators are essential in vibration analysis and control, where they help in identifying and mitigating harmful vibrations that could lead to structural failure or damage. This is particularly important in aerospace and civil engineering, where the integrity of structures under dynamic loads is critical. Moreover, mechanical resonators find applications in energy harvesting and conversion. Piezoelectric materials, which generate an electric charge in response to mechanical stress, are often used in resonant configurations to convert environmental vibrations into electrical energy. This technology has significant potential for powering small devices and sensors in remote or hard-to-reach locations. The synergy between acoustic and mechanical systems is also evident in advanced technologies like ultrasonic cleaning and medical imaging. Ultrasonic cleaners use resonant cavities to generate high-frequency sound waves that dislodge contaminants from surfaces, while medical imaging techniques such as ultrasound rely on mechanical resonators to produce and detect sound waves that provide detailed images of internal body structures. In summary, acoustic and mechanical systems leveraging resonators are pivotal in a wide range of applications, from enhancing sound quality and reducing noise levels to optimizing mechanical performance and enabling innovative technologies. These systems underscore the versatility and importance of resonators in modern engineering and technology.
Advanced Technologies and Innovations
Advanced technologies and innovations have significantly enhanced the applications and uses of resonators, transforming them into indispensable components across various industries. In the realm of telecommunications, resonators are crucial for filtering and tuning frequencies in wireless communication systems, ensuring clear and reliable signal transmission. For instance, quartz crystal resonators are widely used in mobile phones and satellite communications due to their high precision and stability. In the medical field, resonators play a pivotal role in diagnostic imaging technologies such as Magnetic Resonance Imaging (MRI) and ultrasound. Here, resonant cavities and coils are designed to produce precise magnetic fields or sound waves, enabling detailed imaging of internal body structures. This has revolutionized healthcare by providing non-invasive diagnostic tools that offer high-resolution images without the need for ionizing radiation. In aerospace engineering, resonators are integral to navigation systems, particularly in GPS technology. The precise frequency stability provided by these devices ensures accurate location and time synchronization, which is critical for navigation in both civilian and military applications. Furthermore, in the automotive sector, resonators are used in various safety features such as radar systems and collision avoidance technologies. These systems rely on resonant antennas to detect obstacles and measure distances accurately, enhancing vehicle safety and reducing the risk of accidents. Additionally, resonators find extensive use in energy storage and conversion technologies. For example, supercapacitors often employ resonant circuits to optimize charging and discharging cycles, improving efficiency and lifespan. Similarly, in renewable energy systems like solar and wind power, resonant converters help in achieving maximum power transfer from the source to the grid. In consumer electronics, resonators are essential for clocking circuits in computers and other digital devices. Here, they ensure that data processing occurs at precise intervals, maintaining system stability and performance. Moreover, in audio equipment like speakers and microphones, resonant cavities enhance sound quality by amplifying specific frequencies. The advancements in materials science have also led to the development of new types of resonators such as MEMS (Micro-Electro-Mechanical Systems) resonators and graphene-based resonators. These offer miniaturization, higher sensitivity, and improved durability, expanding the potential applications of resonators even further. Overall, the integration of advanced technologies has elevated the functionality and versatility of resonators, making them indispensable in a wide range of applications from telecommunications to healthcare and beyond. As innovation continues to push boundaries, it is likely that resonators will remain at the forefront of technological advancements across multiple disciplines.