What Does Ligase Do
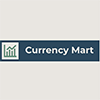
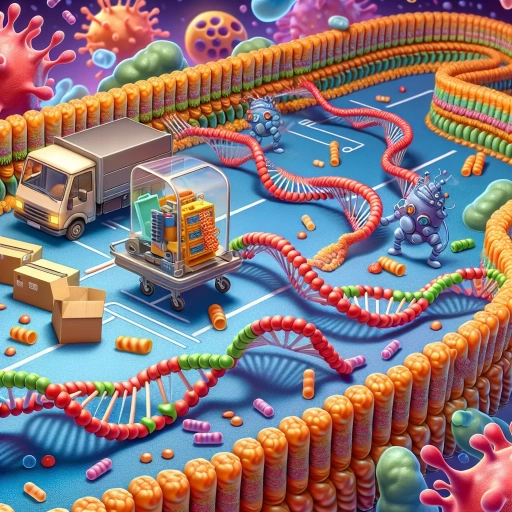
Role of Ligase in DNA Replication
DNA replication is a complex and highly regulated process essential for the survival and propagation of living organisms. At the heart of this process is the enzyme ligase, which plays a crucial role in ensuring the integrity and continuity of the genetic material. Ligase's functions are multifaceted, contributing significantly to several key aspects of DNA replication. Firstly, it seals gaps in the lagging strand, a critical step that prevents genetic material from being lost or damaged. Secondly, ligase ensures genome stability by maintaining the continuity of the DNA molecule, thereby safeguarding against mutations and genetic disorders. Lastly, it facilitates continuous DNA synthesis by joining Okazaki fragments together, allowing the replication process to proceed smoothly. Understanding these roles is vital for appreciating the precision and efficiency of DNA replication. In this article, we will delve into these aspects, starting with the critical function of sealing gaps in the lagging strand, which is essential for maintaining the integrity of the newly synthesized DNA.
Sealing Gaps in the Lagging Strand
During DNA replication, the process of synthesizing new DNA strands is not a continuous affair due to the antiparallel nature of DNA and the unidirectional activity of DNA polymerase. This leads to the formation of short, discontinuous segments known as Okazaki fragments on the lagging strand. These fragments are synthesized in the 5' to 3' direction, but they are not directly linked together. Instead, they leave small gaps between them, which must be sealed to ensure the integrity and continuity of the newly synthesized DNA strand. Here, the enzyme DNA ligase plays a crucial role. DNA ligase is responsible for forming a phosphodiester bond between the 3'-hydroxyl group of one Okazaki fragment and the 5'-phosphate group of the adjacent fragment. This enzymatic reaction effectively seals the gaps, creating a continuous strand of DNA. The process involves several key steps: first, DNA ligase recognizes the nick (gap) between two Okazaki fragments; then, it forms an enzyme-adenylate intermediate, which is followed by the transfer of the adenylate group to the 5'-phosphate end of the nick. Finally, the enzyme catalyzes the attack of the 3'-hydroxyl group on this adenylated phosphate, resulting in the formation of a phosphodiester bond and the release of AMP. The sealing of gaps by DNA ligase is essential for maintaining genomic stability and ensuring accurate DNA replication. Without this enzyme, the newly synthesized strands would remain fragmented, leading to potential genetic mutations and instability. Moreover, DNA ligase is highly specific and efficient, allowing it to recognize and repair nicks in both prokaryotic and eukaryotic cells with precision. In eukaryotes, there are multiple forms of DNA ligase that participate in different aspects of DNA metabolism, including replication, repair, and recombination. In summary, the role of DNA ligase in sealing gaps in the lagging strand during DNA replication is pivotal. By forming phosphodiester bonds between Okazaki fragments, ligase ensures that the newly synthesized DNA is continuous and intact, thereby safeguarding genomic integrity and facilitating accurate genetic transmission from one generation to the next. This enzymatic function underscores the intricate and highly coordinated nature of DNA replication, highlighting how specialized enzymes like DNA ligase contribute to the overall fidelity of this critical biological process.
Ensuring Genome Stability
Ensuring genome stability is a critical process that involves multiple mechanisms to maintain the integrity of DNA. At the heart of this process is the role of DNA ligase, an enzyme that plays a pivotal part in DNA replication and repair. During DNA replication, the genetic material is duplicated to ensure that each daughter cell receives an identical copy of the genome. However, this process is not without its challenges; the replication machinery often encounters gaps and breaks in the DNA strand. Here, DNA ligase steps in to seal these gaps by forming a phosphodiester bond between the 5' phosphate end of one nucleotide and the 3' hydroxyl end of another, thereby restoring the continuity of the DNA strand. The importance of genome stability cannot be overstated. Mutations and errors in DNA replication can lead to genetic disorders, cancer, and other diseases. DNA ligase helps mitigate these risks by ensuring that the newly synthesized DNA strands are accurately joined together. This enzyme is particularly crucial during the lagging strand synthesis phase of DNA replication, where short segments of DNA known as Okazaki fragments are synthesized. Each Okazaki fragment must be joined to its neighbor, a task that DNA ligase accomplishes with precision. In addition to its role in DNA replication, DNA ligase is also essential for DNA repair mechanisms. When DNA damage occurs due to environmental factors or errors during replication, cells employ various repair pathways to correct these defects. For instance, in the context of base excision repair (BER), DNA ligase seals the nick in the DNA backbone after the damaged base has been removed and replaced. Similarly, in non-homologous end joining (NHEJ), a pathway used to repair double-strand breaks, DNA ligase is responsible for sealing the final gap after the broken ends have been processed. The efficiency and accuracy of DNA ligase are vital for maintaining genome stability. Deficiencies in DNA ligase activity have been linked to several genetic disorders, including Bloom syndrome and Fanconi anemia, which are characterized by increased genomic instability and a heightened risk of cancer. Furthermore, the fidelity of DNA ligase ensures that the genetic code is preserved across generations, allowing organisms to maintain their phenotypic traits and adapt to changing environments. In summary, ensuring genome stability is a multifaceted process that relies heavily on the precise action of enzymes like DNA ligase. By sealing gaps and breaks in DNA strands during replication and repair, DNA ligase safeguards the integrity of the genome, preventing mutations and errors that could have deleterious consequences. Its role underscores the intricate and highly regulated nature of DNA metabolism, highlighting the critical importance of enzymatic functions in maintaining cellular health and genetic continuity.
Facilitating Continuous DNA Synthesis
Facilitating continuous DNA synthesis is a critical aspect of DNA replication, and it involves several key enzymes and processes. At the heart of this mechanism is the enzyme DNA ligase, which plays a pivotal role in ensuring that the newly synthesized DNA strands are seamlessly joined together. During DNA replication, the leading strand is synthesized continuously in the 5' to 3' direction by DNA polymerase. However, the lagging strand is synthesized in short, discontinuous segments known as Okazaki fragments due to the antiparallel nature of DNA and the unidirectional activity of DNA polymerase. Each Okazaki fragment is approximately 1000-2000 nucleotides long and is initiated by an RNA primer. Once an Okazaki fragment is completed, the RNA primer must be removed and replaced with DNA, a process carried out by DNA polymerase and an enzyme called RNase H. The gaps between these fragments are then filled with nucleotides by DNA polymerase, leaving only a single phosphodiester bond to be formed between the 3' end of one fragment and the 5' end of the next. This is where DNA ligase steps in, catalyzing the formation of this final phosphodiester bond. By sealing these gaps, ligase ensures that the lagging strand is converted into a continuous, intact DNA strand. This process is essential for maintaining genomic integrity and preventing breaks in the DNA that could lead to mutations or genetic instability. In essence, DNA ligase acts as a molecular "glue," bridging the gaps between Okazaki fragments and facilitating continuous DNA synthesis on the lagging strand. The efficiency and accuracy of this process are crucial for successful DNA replication. Without ligase, the newly synthesized DNA would remain fragmented, compromising the structural integrity and functional viability of the genome. Moreover, defects in DNA ligase activity have been associated with various genetic disorders and increased susceptibility to certain diseases, highlighting its importance in cellular processes. In summary, facilitating continuous DNA synthesis relies heavily on the coordinated action of multiple enzymes, with DNA ligase being a key player. By sealing the gaps between Okazaki fragments on the lagging strand, ligase ensures that DNA replication proceeds accurately and efficiently, safeguarding genomic stability and facilitating the transmission of genetic information from one generation of cells to the next. This underscores the vital role of ligase in maintaining the integrity of DNA and underscores its significance in cellular biology.
Ligase Function in DNA Repair
Ligase, a crucial enzyme in the DNA repair process, plays a pivotal role in maintaining genomic stability and integrity. This enzyme is essential for sealing the gaps in DNA strands, thereby ensuring that genetic information remains intact. The function of ligase can be understood through three key aspects: repairing single-strand breaks, maintaining chromosomal integrity, and correcting mutations and damage. When DNA undergoes single-strand breaks, ligase is vital for rejoining the fragmented strands, preventing further damage and ensuring the continuity of genetic material. Additionally, ligase helps maintain chromosomal integrity by sealing gaps that could otherwise lead to chromosomal instability and potential genetic disorders. Furthermore, it corrects mutations and damage by facilitating the repair of errors introduced during DNA replication and repair processes. Understanding these functions highlights the importance of ligase in safeguarding the genome. Let's delve deeper into the first of these critical roles: repairing single-strand breaks.
Repairing Single-Strand Breaks
Repairing single-strand breaks is a critical process in maintaining the integrity of DNA, and it is here that the enzyme ligase plays a pivotal role. Single-strand breaks occur when one of the two strands of the DNA double helix is severed, often due to oxidative stress, exposure to ionizing radiation, or errors during DNA replication. These breaks can be particularly problematic because they can lead to genetic instability and mutations if not promptly repaired. The repair mechanism involves several key steps, with ligase being the final and crucial enzyme in this process. The repair of single-strand breaks typically begins with the recognition of the damage by specific proteins that bind to the broken DNA. Once recognized, an enzyme called DNA polymerase is activated to fill in any gaps by synthesizing new nucleotides that match the complementary strand. However, this synthesis leaves a small gap where the phosphodiester backbone is broken. This is where DNA ligase comes into play. DNA ligase, also known as DNA joinase, is an enzyme that seals this gap by forming a phosphodiester bond between the 3'-hydroxyl group of one nucleotide and the 5'-phosphate group of another. This reaction is essential for restoring the continuity of the DNA strand. There are different types of DNA ligases, including DNA ligase I and DNA ligase III in eukaryotes, each with specific roles in different repair pathways. In the context of single-strand break repair, DNA ligase III is particularly important. It works in conjunction with other repair proteins such as XRCC1 (X-ray cross-complementing group 1) and PARP1 (poly(ADP-ribose) polymerase 1) to ensure efficient repair. The XRCC1-PARP1 complex helps in recruiting and positioning DNA ligase III at the site of the break, facilitating the ligation reaction. The efficiency and accuracy of this repair process are crucial for maintaining genomic stability. Without functional DNA ligase, single-strand breaks would accumulate, leading to increased genetic mutations and potentially harmful outcomes such as cancer or cell death. Furthermore, defects in DNA ligase have been associated with certain genetic disorders, highlighting the enzyme's importance in overall cellular health. In summary, repairing single-strand breaks is a complex but highly coordinated process that relies on the precise action of DNA ligase. By sealing the gaps in the phosphodiester backbone, ligase ensures that DNA integrity is maintained, thereby protecting against genetic instability and promoting cellular survival. This underscores the vital function of ligase in DNA repair mechanisms and its broader significance in maintaining genomic health.
Maintaining Chromosomal Integrity
Maintaining chromosomal integrity is a critical process that ensures the stability and proper functioning of genetic material within cells. This intricate mechanism involves several key enzymes and pathways, with DNA ligase playing a pivotal role. DNA ligase is an essential enzyme in the DNA repair machinery, responsible for sealing the gaps between Okazaki fragments during DNA replication and repairing single-strand breaks in DNA. In the context of chromosomal integrity, DNA ligase functions by forming a phosphodiester bond between the 5' phosphate end of one nucleotide and the 3' hydroxyl end of another, effectively joining two DNA strands together. This process is crucial for maintaining genome stability, as it prevents the accumulation of DNA breaks that could lead to genetic mutations, chromosomal rearrangements, or even cell death. During DNA replication, the lagging strand is synthesized discontinuously in short segments known as Okazaki fragments. Here, DNA ligase seals these fragments together to form a continuous strand, ensuring that the genetic information is accurately replicated. In addition to replication, DNA ligase is also involved in various DNA repair pathways such as base excision repair (BER) and nucleotide excision repair (NER), where it helps to restore the integrity of DNA damaged by environmental factors or errors during replication. The importance of maintaining chromosomal integrity cannot be overstated; any failure in this process can lead to severe consequences including genetic disorders, cancer, and aging. For instance, defects in DNA ligase IV, a specific isoform of DNA ligase involved in non-homologous end joining (NHEJ), have been associated with immunodeficiency and increased susceptibility to cancer due to impaired ability to repair double-strand breaks. Furthermore, the precision and efficiency of DNA ligase are tightly regulated to prevent aberrant joining of DNA strands, which could result in chromosomal instability. This regulation involves a complex interplay of other proteins and enzymes that work in concert with DNA ligase to ensure accurate repair and replication processes. In summary, maintaining chromosomal integrity relies heavily on the precise function of DNA ligase among other enzymes. By sealing gaps and repairing breaks in DNA, ligase ensures that genetic material remains stable and intact, thereby safeguarding against mutations and chromosomal abnormalities that could have detrimental effects on cellular function and overall health. The critical role of ligase underscores its importance as a cornerstone in the cellular machinery dedicated to preserving genome stability.
Correcting Mutations and Damage
Correcting mutations and damage is a critical process in maintaining the integrity of DNA, and it involves several key enzymes, including ligase. DNA is constantly exposed to various forms of damage, such as ultraviolet light, chemical mutagens, and errors during replication. These damages can lead to mutations that disrupt normal cellular functions and potentially cause diseases like cancer. The cell's response to such damage is mediated by a complex network of DNA repair pathways, each tailored to address specific types of lesions. One of the essential steps in many DNA repair mechanisms is the sealing of gaps in the DNA backbone. This is where ligase plays its crucial role. Ligase, specifically DNA ligase, is an enzyme responsible for forming a phosphodiester bond between the 5' phosphate end of one nucleotide and the 3' hydroxyl end of another, effectively joining two DNA fragments together. This process is vital in both base excision repair (BER) and nucleotide excision repair (NER), two major pathways that correct different types of DNA damage. In BER, ligase seals the gap created after the removal of a damaged base and its sugar-phosphate backbone. This pathway is particularly important for repairing oxidative damage and alkylation lesions. After the damaged base is excised by a DNA glycosylase, an apurinic/apyrimidinic endonuclease (APE1) cleaves the phosphodiester backbone, and a DNA polymerase fills in the resulting gap. Finally, DNA ligase seals this gap, restoring the continuity of the DNA strand. Similarly, in NER, ligase is involved after the excision of a larger segment of DNA containing a lesion. NER is capable of repairing bulky adducts caused by UV radiation, such as cyclobutane pyrimidine dimers. The process involves the recognition of the lesion by UvrA and UvrB proteins, followed by the excision of a 24-32 nucleotide segment containing the damage. DNA polymerase then synthesizes new DNA to replace this segment, and once again, DNA ligase seals the final gap. The precision and efficiency of ligase in forming these phosphodiester bonds are crucial for maintaining genome stability. Without proper ligation, DNA fragments would remain disjointed, leading to genetic instability and potentially harmful mutations. Moreover, defects in DNA ligase function have been associated with increased susceptibility to cancer and other genetic disorders. In summary, correcting mutations and damage in DNA is a multifaceted process that relies heavily on the accurate and efficient sealing of DNA gaps by ligase. This enzyme's role in both BER and NER underscores its importance in ensuring the integrity of genetic material, thereby protecting cells from the deleterious effects of DNA damage. The precise action of ligase in forming phosphodiester bonds is a cornerstone of cellular defense mechanisms against genetic alterations, highlighting its indispensable function in DNA repair processes.
Applications of Ligase in Biotechnology
Ligase, an enzyme crucial for forming covalent bonds between DNA fragments, has revolutionized various fields within biotechnology. Its applications are multifaceted and highly impactful, contributing significantly to advancements in cloning and gene expression, PCR and DNA sequencing, and genetic engineering and gene therapy. In the realm of **Cloning and Gene Expression**, ligase is essential for assembling recombinant DNA molecules, allowing researchers to study gene function and produce therapeutic proteins. This enzyme facilitates the precise joining of DNA fragments, enabling the creation of genetically modified organisms and the expression of foreign genes in host cells. Additionally, **PCR and DNA Sequencing** rely on ligase for the construction of cloning vectors and the preparation of DNA libraries, which are critical for amplifying and analyzing genetic material. Furthermore, **Genetic Engineering and Gene Therapy** utilize ligase to introduce specific genetic modifications, aiming to treat or prevent diseases by correcting or replacing defective genes. By understanding the role of ligase in these processes, we can appreciate its central importance in biotechnological research and development. Transitioning to the specifics of **Cloning and Gene Expression**, it becomes clear how ligase's precision and efficiency are pivotal in these applications.
Cloning and Gene Expression
Cloning and gene expression are pivotal processes in biotechnology, leveraging the enzyme ligase to facilitate the manipulation of genetic material. Cloning involves the creation of multiple copies of a DNA sequence, which is crucial for various biotechnological applications. This process begins with the isolation of the target DNA fragment, often achieved through restriction enzymes that cut the DNA at specific sites. The resulting fragments are then joined to a vector, such as a plasmid, using DNA ligase. Ligase seals the gaps between the DNA fragments by forming phosphodiester bonds, effectively linking the target sequence to the vector. Once the recombinant DNA molecule is formed, it is introduced into a host organism, typically bacteria, where it can replicate and produce multiple copies of the cloned DNA. Gene expression, on the other hand, involves the translation of genetic information encoded in DNA into proteins. This process is essential for understanding how genes function and for producing therapeutic proteins. In biotechnology, gene expression can be controlled and optimized using various techniques, including the use of promoters and enhancers that regulate transcription. The cloned DNA, now part of the host's genome, can be induced to express the desired protein under specific conditions. For instance, in recombinant protein production, bacteria or yeast can be engineered to produce human insulin or other therapeutic proteins by expressing the corresponding genes. The applications of these technologies are vast and diverse. In medicine, cloning and gene expression enable the production of vaccines, hormones, and other therapeutic proteins. For example, human insulin produced through recombinant DNA technology has revolutionized the treatment of diabetes. In agriculture, genetically modified organisms (GMOs) can be engineered to resist pests, tolerate environmental stresses, or enhance nutritional content. Additionally, cloning techniques are used in forensic science for DNA fingerprinting and in basic research to study gene function and regulation. The precision and efficiency of these processes rely heavily on the activity of DNA ligase. By ensuring that DNA fragments are accurately joined together, ligase plays a critical role in maintaining the integrity of the genetic material. This is particularly important in applications where the fidelity of the cloned sequence is paramount, such as in the development of gene therapies or the creation of genetically modified crops. In summary, cloning and gene expression are fundamental tools in biotechnology, and their success is significantly dependent on the enzymatic activity of ligase, which facilitates the precise manipulation and replication of genetic material.
PCR and DNA Sequencing
Polymerase Chain Reaction (PCR) and DNA sequencing are two pivotal techniques in biotechnology that have revolutionized the field of molecular biology. PCR, developed by Kary Mullis in 1983, is a method that allows for the exponential amplification of specific DNA sequences. This process involves denaturing the DNA, annealing primers to the target sequence, and extending these primers using a thermostable DNA polymerase, such as Taq polymerase. By repeating these cycles, PCR can generate millions of copies of the target DNA sequence from a small initial sample, making it an indispensable tool for genetic research, forensic analysis, and clinical diagnostics. DNA sequencing, on the other hand, is the process of determining the precise order of nucleotides (adenine, guanine, cytosine, and thymine) in a DNA molecule. The most commonly used method is Sanger sequencing, developed by Frederick Sanger and his colleagues in 1977. This technique involves synthesizing a complementary strand to the target DNA by incorporating dideoxynucleotides that terminate the reaction at specific points, allowing for the identification of each nucleotide base. Next-generation sequencing (NGS) technologies have further advanced this field by enabling high-throughput sequencing of entire genomes in a single run, significantly reducing costs and increasing speed. The synergy between PCR and DNA sequencing is crucial for various biotechnological applications. For instance, in genetic engineering, PCR can be used to amplify specific genes or sequences before they are cloned into vectors for further manipulation. Subsequent DNA sequencing ensures that the cloned sequences are accurate and free from mutations. In forensic science, PCR amplifies trace amounts of DNA evidence, which is then sequenced to identify individuals or solve crimes. In clinical settings, these techniques are used for diagnosing genetic disorders by amplifying and sequencing specific genes associated with diseases. Moreover, the integration of PCR with DNA sequencing has facilitated the development of advanced diagnostic tools such as real-time PCR (qPCR), which allows for the quantification of gene expression levels and the detection of pathogens. This combination also underpins genome editing technologies like CRISPR-Cas9, where precise sequences are amplified and sequenced to validate the efficacy of gene editing. In the context of ligase applications in biotechnology, PCR and DNA sequencing play complementary roles. Ligases are enzymes that form covalent bonds between DNA fragments, a critical step in cloning and gene assembly. After ligating DNA fragments together, PCR can be used to amplify the resulting constructs for further analysis or manipulation. Subsequent DNA sequencing verifies the integrity and accuracy of these constructs, ensuring that they are correctly assembled without any unwanted mutations or insertions. In summary, PCR and DNA sequencing are fundamental tools in biotechnology that work in tandem to facilitate a wide range of applications from genetic research to clinical diagnostics. Their integration with ligase-mediated reactions enhances the precision and efficiency of genetic engineering processes, underscoring their indispensable role in advancing biotechnological innovations.
Genetic Engineering and Gene Therapy
Genetic engineering and gene therapy are revolutionary fields that leverage advanced biotechnological tools, including the enzyme ligase, to manipulate and repair genetic material. Genetic engineering involves the deliberate alteration of an organism's DNA to introduce new traits or enhance existing ones. This process often relies on ligase, an enzyme that seals gaps in DNA strands by forming phosphodiester bonds, thereby completing the integration of foreign DNA into a host genome. For instance, in recombinant DNA technology, ligase is crucial for joining the cut ends of DNA fragments generated by restriction enzymes, allowing scientists to create hybrid DNA molecules that can be inserted into vectors for cloning or expression in host cells. Gene therapy, a subset of genetic engineering, focuses specifically on treating or preventing diseases by correcting or replacing genes responsible for genetic disorders. Here, ligase plays a pivotal role in ensuring the precise integration of therapeutic genes into the patient's genome. In ex vivo gene therapy, cells are removed from the patient, genetically modified using techniques that involve ligase-mediated DNA repair, and then reintroduced into the body. For example, in the treatment of severe combined immunodeficiency (SCID), also known as "bubble boy disease," gene therapy involves using ligase to integrate a functional copy of the defective gene into hematopoietic stem cells, which are then reinfused into the patient to restore immune function. The precision and efficiency of ligase in these processes are paramount. High-fidelity ligases, such as DNA ligase I and DNA ligase IV, are essential for maintaining genomic integrity during gene editing and therapy. These enzymes ensure that DNA repair occurs accurately, minimizing the risk of mutations that could lead to unintended consequences. Furthermore, advancements in ligase technology have enabled the development of more sophisticated gene editing tools like CRISPR-Cas9, where ligase is used in conjunction with other enzymes to facilitate precise genome modifications. The applications of genetic engineering and gene therapy extend across various fields, including agriculture, pharmaceuticals, and medicine. In agriculture, genetic engineering allows for the creation of crops with enhanced nutritional content, pest resistance, and drought tolerance. In pharmaceuticals, genetically engineered organisms can produce therapeutic proteins and vaccines more efficiently. In medicine, gene therapy holds promise for treating a wide range of genetic disorders, from inherited diseases like cystic fibrosis to acquired conditions such as cancer. In summary, ligase is a cornerstone enzyme in genetic engineering and gene therapy, facilitating the precise manipulation and repair of DNA. Its role in forming stable phosphodiester bonds ensures that genetic modifications are integrated accurately into the genome, making it an indispensable tool in biotechnology. As research continues to advance, the applications of ligase in these fields are likely to expand, offering new avenues for treating diseases and improving human health.