What Is Energy Measured In
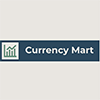
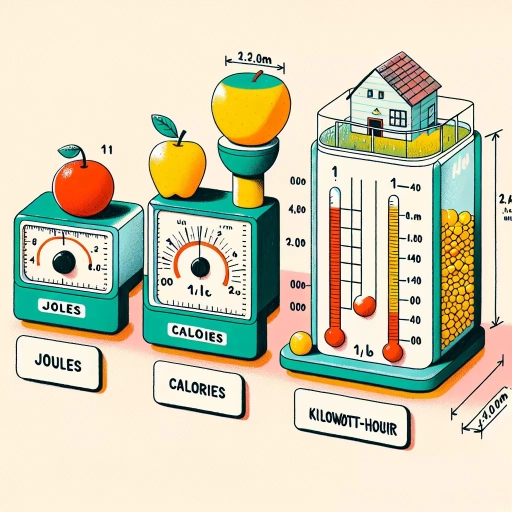
Understanding the Basics of Energy Measurement
Understanding the basics of energy measurement provides a crucial foundation for comprehending the engines of life and global processes that drive our world. The concept of energy, its quantities, and calculations, though is intangible, influences every aspect of our lives and the universe. The depth of this understanding can be enriched by delving into three primary facets of energy measurement - the definition and importance of energy units, the historical development of energy measurement, and the common energy units encountered in everyday life. Recognizing the critical role energy units play in quantifying and comparing various forms of energy is pivotal to various scientific, industrial, and daily life applications. The exploration of the historical trajectory of energy measurement provides intriguing insights into our progress and environmental awareness. Additionally, familiarizing ourselves with common energy units can render more tangible and relatable the otherwise abstract concept of energy. Thus, the exploration journey begins with the first step toward understanding this complex realm - the definition and importance of energy units. Overall, each supporting paragraph will serve as a stepping-stone in our journey to unravel the complexities of energy measurement.
Definition and Importance of Energy Units
Understanding the subtle complexities of the energy spectrum warrants a thorough investigation into the definition and importance of energy units. These fundamental building blocks play a critical role in our ability to measure, monitor, and manage the various forms of energy we constantly interact with in our daily lives. Analogous to the units we use for metrics such as mass or distance, energy units provide us with a universal language to quantify energy in a way that is understood and recognized across different cultures, industries, and scientific disciplines. Energy is a basic element of the universe, driving everything from the expansion of galaxies to the spin of electrons in atoms. Our Sun alone emits over 400 septillion Joules of energy every second, an almost incomprehensible amount that is easier to grasp when broken down into smaller, more manageable units. From kilowatt-hours used to measure electricity consumption in our homes, to British Thermal Units for heating systems, calories for food energy or electron volts filling quantum physics textbooks, the energy units we use reflect the diverse nature of the forms and scales of energy. The importance of these units extends beyond their linguistic convenience. Without the ability to measure energy, we would be essentially blind to the dynamics of the world around us. The structural integrity of buildings, the efficiency of engines, and the sustainability of our resources all hinge on our ability to measure and thus understand energy. Moreover, our ability to convert energy units based on context-specific requirements integrates seamlessly into economic, political, and environmental decision-making processes. For example, government policies on renewable energy heavily rely on energy unit conversions to assess and project resource availability and consumption rates. Furthermore, energy units are integral to advancements in science and technology, fostering innovation. Energy units guide engineers to design energy-efficient appliances, help astrophysicists determine the lifespan of stars, and aid health professionals in understanding how our body processes food energy. In fact, even our comprehension of climate change is heavily influenced by how we quantify and track energy in the form of greenhouse gas emissions. In conclusion, our understanding of energy, the driving force of the universe, is inherently tied to units of energy. These definitions not only facilitate unified communication across varied sectors but also help monitor and strategize energy management and conservation. Without these fundamental units, our comprehension and control of the world around us would be significantly undermined. Therefore, appreciating and understanding the definition and importance of energy units is paramount in crafting a better, more efficient, and sustainable world.
Historical Development of Energy Measurement
Energy measurement development has a rich historical context that dates back centuries. The concept of energy, as we understand it now, was not always widely accepted or defined. The initial understanding of energy evolved from the Greek concept of 'energeia', which roughly translated to operation or activity. It was first proposed by Aristotle in his work "Metaphysics". The concept was left relatively untouched until the 17th century, when the studies of scientist Galileo Galilei gave birth to kinematics, thereby laying the foundation for what we now understand as the concept of energy. Progressing into the 18th century, energy transitioned from a philosophical concept and became a fundamental characteristic within the physical world. It was during this time that Benjamin Thompson and Nicholas Carnot proposed the theories of heat as a form of energy. This effectively linked mechanical work and heat, thereby introducing the concept of energy conservation. This understanding of energy was later refined in the 19th century by the German physicist Hermann von Helmholtz, who formulated the principle of conservation of energy, stating all energy in a closed system remains constant. It was in the late 19th and early 20th century, when the term 'energy' finally entered common parlance. Scientists such as James Prescott Joule and Rudolf Clausius further developed the concept of 'energy' by introducing the idea of 'joule' as a unit of measurement. Joule contributed to the development of a system to measure mechanical work in terms of heat, thereby lending his name to the unit of energy in the International System of Units (SI). Clausius’s theory on heat, on the other hand, introduced the concept of entropy – as a measure of energy dispersal at a specific temperature. Into the 20th century, Albert Einstein gave the world the famous equation E=mc², indicating that energy (E) equals mass (m) times light (c) squared. This revolutionary concept firmly established that energy and mass were interchangeable, laying down the groundwork for nuclear energy. In the midst of the 21st century, measuring energy has taken a significant role in daily life, from the electricity we consume, to the calories we intake and the gasoline our cars use. It is not only an essential element for scientific research but also for economic development, environmental protection and even for managing our daily activities. From a popularly disregarded Greek idea to an indispensable scientific and mathematical concept that has shaped numerous breakthroughs in technology and revolutionized our understanding of the universe, energy, and its measurement has come a long way. This historical evolution of the concept and its measurement instances the ever-evolving nature of science that continuously builds on the knowledge of the forerunners, leading to more precise understandings and broader applications.
Common Energy Units in Everyday Life
In the simplest terms, energy is the ability to do work, and it is pivotal to almost every process on Earth, including human activities. It is central to such crucial sectors like industrial processes, transportation, heating, and environmental conditions, making understanding how it is measured a practical necessity. More so, the usage of dominant units of measurement such as Joules, Watts, and calories in everyday life is indispensable. One of the most common energy units is the Joule – named after James Prescott Joule, a physicist who significantly contributed to the development of the concept of energy. It's the unit for the amount of energy transferred when one Newton force moves one meter in the direction of the force. Uses are numerous – from quantifying heat energy in scientific contexts to measuring the energy savings advertised by light bulb manufacturers. Watt, conversely, is a common unit of power, which is the rate at which energy is processed or produced. Essentially, a Watt equals one Joule per second, and it is commonly used in electrical contexts. We use Watts every day when interacting with electrical devices. For instance, light bulbs are rated in watts to signify their power usage, while power companies use kilowatts, which are 1000 watts, or megawatts, which are a million watts, to quantify the power supplied to homes or industries. Lastly, calories, while technically units of heat energy, are used predominantly to measure the energy provided by food. One calorie (or actually, kilocalorie in scientific terms) is the amount of heat needed to raise the temperature of a kilogram of water by one degree Celsius. This metric is particularly important for diet monitoring and health-conscious individuals. Regardless of the unit used, each constitutes a vital lens for interpreting rows of numbers into meaningful, actionable insights about energy usage and conservation. Thus, understanding these common energy units better equips us to navigate and participate in the ongoing dialogue about energy efficiency, sustainability, and climate change.
Standard Units for Measuring Energy
Energy is the capacity to do work, a fundamental concept that underpins our understanding of the universe. To quantify and compare various types of energy, standard units of measurement are needed. Defining energy in absolute numerical values allows for accurate calculations, which are paramount in fields such as physics, engineering, nutrition, and environmental studies. The most common units used to measure energy include Joules in the International System of Units (SI), Calories, and British Thermal Units (BTUs). Each of these units holds its unique importance and is used across different arenas of science and industry. The first unit we'll explore is Joules, a pivotal fixture within the International System of Units. As we examine these units, we'll elucidate upon their definitions, conversions, and applications, underscoring the essential role they play in our understanding and manipulation of energy.
Joules and the International System of Units (SI)
The International System of Units (SI) is globally accepted as the unwavering basis for expressing quantities of every scientific and technical nature. It encompasses several units, each with its function and domain; however, our interest here lies within the sphere of energy measurement. Energy, a fundamental concept in physics, is usually measured in a unit called a Joule. The Joule, abbreviated as 'J', is recognized worldwide and became part of the SI units in 1971. A Joule is precisely described as a unit of work done when a one-newton force moves an object through a one meter distance in the direction of the force. The joule has also found wide acceptance in the measurement of different forms of energy, including kinetic, potential, electrical, and thermal energy. This comprehensive utility of joules underlines its versatility and inherent value in capturing standardized measures across variable contexts. For instance, in the realm of electrical energy, a joule is the energy transferred when a one Ampere current is passed through a resistance of one Ohm for one second. In practical examples like the calorie content of food, the joule's utility shines brightly as it provides a scientific counter to the commonplace, enabling a better understanding of energy exchanges in everyday life. Crucially, the adoption of joule as the standard unit for energy measurement brings an unprecedented uniformity and comparison ease between different types of energy transfers and transformations. It has been pivotal in facilitating advancements in technology and science by providing a standardized and universally accepted system for quantifying energy. The standardization eliminates confusion among scientific communities and fosters precise communication of scientific findings and technological developments across global borders. In essence, joules and the adoption of the comprehensive International System of Units (SI) signify a leap in our understanding and expression of scientific phenomena, particularly energy. Through its standardization and wide acceptance, it has not only shaped our conceptual understanding of energy but also provided a reliable tool for measurement across diverse fields of study and applications. The influence of joules in the world of energy extends far beyond mere numbers, enabling a deep and meaningful understanding of the world and the energy processes that enable and sustain life as we know it. Such is the power and relevance of the humble joule in the majestic realm of energy.
Calories and Their Application in Different Fields
While we mostly associate calories with nutrition and physical activities, it’s important to know that they are, in essence, units for measuring energy. A calorie, as a basic unit, is defined as the amount of energy required to raise the temperature of one gram of water by one degree Celsius. It's universal in nature and finds its applications in different fields beyond just dietetics and personal health. In the realm of biology and biochemistry, calories are integral in understanding the energy produced, consumed, and stored by an organism. Plants, for instance, convert sunlight into energy through photosynthesis, which is measured in calories. When these plants are eaten by animals or humans, these calories are further converted into energy necessary for survival and performing activities. Scientists in these fields often use the kilocalorie (Kcal), or large calorie, as the standard unit for measuring the energy within biological systems, highlighting the pivotal role calories play in life-energy dynamics. Moreover, in physics and chemistry, calories measure the energy involved in heating, cooling, or changing the state of matter. The process of combustion, for instance, is fundamentally a reaction that liberates energy, measured in calories, explaining why it's extensively used in heat engines or within our households. In the food industry, understanding the caloric content of foodstuff is crucial for nutritional labelling, dietary regulations, and promoting consumer awareness. From an industrial point of view, calories are deployed to estimate the energy output and efficiency of various machinery and engines. For instance, evaluating the performance of fuels where the potential energy content, measured in calories, can decide the power output of the combustion engine. Similarly, in sports science and physiology, calories are used to calculate and manage the energy expenditure of athletes during physical activities. This knowledge empowers the creation of specific diet plans and training regimens to optimize performance while ensuring overall well-being. Also, in environmental science, the calorific value of waste products can be used to recover energy through incineration, thus converting waste into a renewable energy source. This innovative approach is increasingly getting recognized for its potential in sustainable waste management. From the microscopic cells within our bodies to the grand cosmic phenomena, the interplay of energy, as measured in calories, remains a constant. Whether it's understanding our dietary needs, improving performances, or designing efficient systems, knowing the caloric value provides us a tool for rational assessments, planning, and decision-making. Therefore, calories prove to be one of the most versatile and universal standard units for measuring energy across different fields.
British Thermal Units (BTUs) and Their Usage
British Thermal Units (BTUs) are pivotal to understanding energy measurement, particularly within the United States and the United Kingdom. Traditionally, a BTU, a unit of heat energy in the Imperial system of measurement, is the amount of heat required to increase the temperature of one pound of water by one degree Fahrenheit. In simpler terms, one BTU is equivalent to roughly 1055 joules in the International System of Units. Moreover, a BTU manifests its significance in various arenas such as in the realm of thermodynamics, in air conditioning systems, in various household appliances, and in the natural gas industry. Fundamentally in thermodynamics, BTUs find their utility as a measure for heat energy or the energy content within fuel. It is a game-changer that facilitates a comprehensive comparison between different types of energy. In air conditioning systems, BTUs signify the cooling or heating capacity. An air conditioner with a higher BTU rating implies that the system can cool a larger area or a higher volume of air within a given time frame. Similarly, household appliances, particularly heating systems and cooking appliances use BTUs as a standard indicator for energy consumption. Products with higher BTU ratings indicate more energy usage thereby making BTUs a crucial factor in determining the appliance’s operational cost. Natural gas industry offers another classic example of BTU’s extensive usage. Natural gas is often sold in terms of its heat energy content that is expressed in BTUs. This implies that the price mentioned corresponds to one million BTUs (MBTU). Hence, to comprehend the parity in natural gas prices, understanding BTUs is indispensable. BTUs, therefore, underscores the energy efficiency of a product and aids in rational decision making based on the energy requirement. In retrospect, BTUs emerge as a standard unit for measuring energy that facilitates a coherent understanding and comparison across different spheres. The extensive usage of BTUs from thermodynamics to the natural gas industry further underscores its irreplaceable significance. Therefore, BTUs feature as one of the key calorie-based units that contributes to a more nuanced understanding when navigating the broad spectrum of energy measurement.
Specialized Energy Units Across Various Disciplines
Energy, synonymous with the power to perform work, remains a crucial aspect across numerous disciplines, each adopting specific units of measurement. The techniques and metrics used in varying fields emphasize the diversity and complexity of energy as a concept. The ensuing discourse will delve into three particular disciplines, elucidating the unique energy units they employ. Principally, we will explore how electrical energy utilizes Kilowatt-Hours (kWh) and Volts-Amps (VA), then proceed to understand the application of British Thermal Units (BTUs) and Calories in thermal energy, specifically in scientific research. Finally, we will navigate through the intricacies of chemical energy, shedding light on the use of Moles and Electronvolts (eV) in chemistry. Even though these energy units are specific to their respective fields of study, they all contribute to our broader comprehension of energy, its conversion, and its transference. To commence, we will focus our attention on the realm of electrical energy. In this domain, knowing how to gauge power consumption and generation by employing Kilowatt-hours (kWh) and the rate at which electricity works using Volts-amps (VA) is fundamental for engineers and technicians.
Electrical Energy: Kilowatt-Hours (kWh) and Volts-Amps (VA)
Specialized units often play a crucial role in specific disciplines, particularly in the field of electrical energy. Two units of measurement, Kilowatt-Hours (kWh) and Volts-Amps (VA), are indispensable when it comes to understanding the complexities of electrical energy. The Kilowatt-Hour (kWh) is a common unit that is primarily used to measure electrical energy, especially for residential and commercial applications. In essence, a Kilowatt-Hour is the amount of energy consumed over time, gauging the electrical power over an hour. If an electrical device rated at 1 kilowatt (1000 watts) operates for one hour, it will consume 1 kWh of energy. Interestingly, the price of electricity is usually based on kWh, making it a key term in energy economics as well as discussions about energy efficiency and renewable technologies. On the other hand, the Volt-Amp (VA) is a unit used to express apparent power in an electrical circuit. It is a slightly more complex unit, mainly used in power engineering. The apparent power (measured in VA) is the total power in an AC circuit, both used power (also known as real power) and reactive power. The real power is what does the actual work (lighting a bulb or heating a room), while the reactive power is the power that oscillates back and forth, contributing to the magnetic and electric fields in the circuit. What makes the Volt-Amp distinct is its capacity to measure the real power as well as the reactive power, portraying a more comprehensive picture of energy flow in an electrical circuit. Hence, the VA finds its utility not only in understanding the theoretical aspects of power but also in analyzing and troubleshooting the performance of electrical utilities and devices. To understand these units' practical implications, consider that any electrical circuit, from a small domestic utility to a massive power station, experiences a combination of real power and reactive power. Therefore, by understanding units like kWh and VA, we not only get a clear measurement of electrical power and energy but also grasp the operational efficiency and technical parameters of our electric-dependent world. Identifying and effectively measuring these units thus plays a critical role in properly managing and optimizing our electrical energy consumption. This use of specialized units across various disciplines, particularly electrical energy, indicates the immense importance of precise and contextual energy measurement in human life.
Thermal Energy: BTUs and Calories in Scientific Research
In scientific research, thermal energy is often measured in either British Thermal Units (BTUs) or calories. Both units provide crucial insights in energy-related studies, however, they are utilized differently across varying disciplines. The BTU, a traditional unit of heat, is used predominantly in the English-speaking world, specifically within industries and residential settings concerned with heating and cooling systems. Basically, a BTU signifies the quantity of thermal energy required to increase the temperature of 1 pound of water by 1 degree Fahrenheit. This unit is imperative to evaluate the performance of heat-producing appliances like furnaces, heaters, and air-conditioners; moreover, it is heavily utilized in energy conversion research making it instrumental in fields studying energy efficiency or renewable energy technologies. On the other hand, the calorie (cal) is extensively used in the scientific community, particularly in the realms of physics, chemistry, and biology. A calorie is defined as the amount of heat energy needed to raise the temperature of 1 gram of water by 1 degree Celsius. Notably, biologists and nutritionists often use this unit predominantly to measure the energy content of food, thus, calories you usually see in nutritional labels are actually kilocalories (Kcal), given that 1 Kcal is equal to 1,000 calories. Meanwhile, in physics and chemistry research, the small calorie facilitates an understanding of molecular, atomic, and nuclear interactions. Nonetheless, both BTUs and calories are significantly utilized in thermodynamics, the study of heat energy exchange involving work done, heat transfer, and changes in system volumes. Their usage supports critical computations in predicting energy changes, studying thermal power generation, or implicating thermal technology principles. Truly, the BTU and calorie, though measured differently, share the same essential role in quantifying heat or thermal energy, providing a consistent tool in fostering our comprehension of the world's energy dynamics. Understanding BTUs and calories, and their interplay across diverse scientific fields, not only equips us with a deeper knowledge of energy behavior but also fuels further advancements and breakthroughs in scientific research and technological applications.
Chemical Energy: Moles and Electronvolts (eV) in Chemistry
Chemical energy, one of many types of specialized energy used across various disciplines, hinges largely on the concepts of moles and electronvolts (eV) in Chemistry. A mole is a unit used in Chemistry that represents 6.02214076 × 10²³ particles, which can be atoms, molecules, ions, or electrons. Understanding moles and their relationship to chemical reactions is fundamental to comprehending Chemical energy. Chemical energy relies on the arrangement of atoms in a substance. During a chemical reaction, bonds between atoms are broken and formed, setting off an exchange of energy. The energy withdrawn or invested in chemical reactions is frequently stated in kilojoules per mole (kJ/mol). Hence, the amount of energy or work done in a chemical reaction often involves moles. A high energy per mole signifies a large amount of work realized in the chemical process, pointing to a highly reactive substance. On the other hand, electronvolts (eV) tie in the concepts of energy and electric charge. An electronvolt is an incredibly tiny unit of energy used mainly in atomic and nuclear physics and relates to the energy gained by a single electron when it accelerates through an electric potential difference of one volt. In context, it's used to express the kinetic energy of particles or the energy levels of atoms. In a popular energy unit conversion, 1 electronvolt equals approximately 96.5 kilojoules per mole. Therefore, eV and kJ/mol can interchangeably describe the energy difference between different atomic or molecular states. In the realm of Chemistry, moles and electronvolts (eV) have a central role in formulating the energy dynamics at a molecular level. A deeper grasp of these concepts enhances the understanding of energy transformation, as they relay how micro units like atoms and electrons interact to drive broader chemical responses. Thus, in the real-world scientific explorations, chemical energy and its intricate relation with moles and electronvolts (eV) strengthen the comprehensive view of energy quantity, distribution, and transformation across diverse disciplines and systems.