What Is Gcms Notes
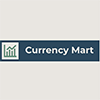
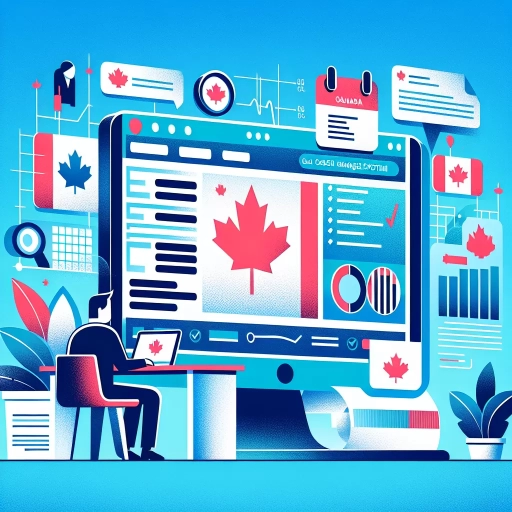
Gas Chromatography-Mass Spectrometry (GCMS) is a powerful analytical technique that has revolutionized various fields such as chemistry, biology, and environmental science. This sophisticated method combines the separation capabilities of gas chromatography with the mass analysis capabilities of mass spectrometry, allowing for the identification and quantification of complex mixtures. In this article, we will delve into the fundamentals of GCMS, exploring its technical aspects, applications, and benefits. First, we will **Understand the Basics of GCMS**, laying the groundwork for how this technique operates. Next, we will dive into **How GCMS Works: Technical Aspects**, detailing the intricate processes involved in separating and identifying compounds. Finally, we will examine **Applications and Benefits of GCMS**, highlighting its significance in various scientific and industrial contexts. By understanding these core components, readers will gain a comprehensive insight into the world of GCMS and its pivotal role in modern analytical science. Let's begin by **Understanding the Basics of GCMS**.
Understanding the Basics of GCMS
Understanding the basics of Gas Chromatography-Mass Spectrometry (GCMS) is crucial for anyone involved in analytical chemistry. This powerful analytical technique combines the separation capabilities of gas chromatography with the identification capabilities of mass spectrometry, making it a cornerstone in various scientific fields. To grasp GCMS fully, it is essential to delve into three key areas: the definition and purpose of GCMS, the key components that make up the system, and its historical development. Firstly, understanding the **definition and purpose of GCMS** lays the foundation for appreciating its role in modern science. GCMS is used to separate, identify, and quantify the components of a mixture, making it invaluable in fields such as forensic science, environmental monitoring, and pharmaceutical research. Secondly, knowing the **key components of GCMS** helps in understanding how the system operates. These components include the gas chromatograph, which separates the mixture into its individual components, and the mass spectrometer, which identifies these components based on their mass-to-charge ratio. Lastly, exploring the **historical development of GCMS** provides insight into how this technique has evolved over time. From its early beginnings in the mid-20th century to the sophisticated instruments available today, understanding this history highlights the advancements and innovations that have made GCMS such a powerful tool. By starting with the **definition and purpose of GCMS**, we can establish a clear understanding of why this technique is so important and how it is applied in various contexts. This foundational knowledge will then pave the way for a deeper exploration of its components and historical development.
Definition and Purpose of GCMS
**Understanding the Basics of GCMS: Definition and Purpose** Gas Chromatography-Mass Spectrometry (GCMS) is a powerful analytical technique that combines the separation capabilities of gas chromatography (GC) with the mass analysis capabilities of mass spectrometry (MS). The **definition** of GCMS revolves around its dual functionality: GC separates the components of a mixture based on their boiling points and affinity for the stationary phase, while MS identifies these components by measuring their mass-to-charge ratio. This synergy allows for the precise identification and quantification of individual compounds within complex mixtures. The **purpose** of GCMS is multifaceted. Primarily, it serves as a diagnostic tool in various fields such as environmental monitoring, pharmaceutical research, forensic science, and food safety. In environmental monitoring, GCMS helps detect and quantify pollutants like volatile organic compounds (VOCs) and pesticides in air, water, and soil samples. In pharmaceutical research, it aids in the identification of active pharmaceutical ingredients (APIs) and impurities, ensuring drug purity and efficacy. Forensic scientists use GCMS to analyze evidence such as blood alcohol levels, narcotics, and explosives. Additionally, in food safety, GCMS is employed to detect contaminants like heavy metals and residues of pesticides. GCMS also plays a crucial role in quality control processes across industries. For instance, it helps in the analysis of petroleum products to determine their composition and quality. In the field of biotechnology, GCMS is used to study metabolic pathways by analyzing volatile metabolites. Furthermore, its high sensitivity and specificity make it an invaluable tool for detecting trace amounts of substances, which is particularly important in fields where even small concentrations can have significant impacts. In summary, the definition of GCMS highlights its integrated approach to separating and identifying chemical compounds, while its purpose underscores its versatility and critical role in various scientific and industrial applications. By combining the strengths of both GC and MS, GCMS provides comprehensive insights into the composition of complex mixtures, making it an indispensable tool for researchers and analysts alike.
Key Components of GCMS
The key components of Gas Chromatography-Mass Spectrometry (GCMS) are crucial for understanding the functionality and applications of this analytical technique. At the heart of GCMS is the **Gas Chromatograph**, which separates the components of a mixture based on their boiling points and affinity for the stationary phase. This separation occurs within a **Column**, typically a narrow, coiled tube coated with a stationary phase that interacts differently with various compounds. The **Injector** introduces the sample into the column, where it is vaporized and carried by an inert gas such as helium or nitrogen. The **Oven** controls the temperature of the column, allowing for precise temperature programming to optimize separation. As the separated components exit the column, they enter the **Mass Spectrometer**, which identifies them based on their mass-to-charge ratio. The mass spectrometer consists of an **Ion Source**, where molecules are ionized; a **Mass Analyzer**, which separates ions according to their mass-to-charge ratio; and a **Detector**, which measures the abundance of each ion. The **Vacuum System** maintains the low-pressure environment necessary for the mass spectrometer to function accurately. **Data Acquisition and Processing Software** interprets the data from the detector, providing detailed spectra and chromatograms that help in identifying and quantifying the components of the sample. Additionally, **Detectors** such as Electron Ionization (EI) or Chemical Ionization (CI) are used to ionize the molecules, while **Mass Analyzers** like Quadrupole, Time-of-Flight (TOF), or Ion Trap further refine the separation and identification process. Understanding these components is essential for optimizing GCMS performance, ensuring accurate and reliable results in various fields such as forensic science, environmental monitoring, pharmaceutical analysis, and food safety testing. Each component plays a vital role in the overall process, from sample introduction to data interpretation, making GCMS a powerful tool for chemical analysis.
Historical Development of GCMS
The historical development of Gas Chromatography-Mass Spectrometry (GCMS) is a narrative of scientific innovation and technological advancement that spans several decades. The journey began in the early 20th century with the invention of gas chromatography (GC) by Russian scientist Mikhail Tsvet in 1903, who used it to separate plant pigments. However, it wasn't until the 1950s that GC gained widespread acceptance as a powerful analytical tool, particularly after the work of Martin and Synge in 1941, who laid the theoretical foundations for the technique. The integration of mass spectrometry (MS) with GC marked a significant milestone. Mass spectrometry itself has its roots in the early 20th century, with J.J. Thomson's discovery of isotopes in 1913 and the development of the first mass spectrometer by Dempster in 1918. The first practical GCMS system was developed in the late 1950s and early 1960s by scientists such as Holmes and Morrell, who successfully coupled a gas chromatograph to a mass spectrometer. The 1960s saw rapid improvements in GCMS technology, driven by advancements in electronics, computerization, and detector sensitivity. The introduction of quadrupole mass analyzers in the 1960s and 1970s further enhanced the capabilities of GCMS by providing higher resolution and sensitivity. This period also witnessed the development of more sophisticated data acquisition and processing systems, which enabled the analysis of complex mixtures and the identification of unknown compounds. The 1980s and 1990s brought about significant advancements in instrumentation, including the introduction of benchtop GCMS systems that were more affordable and accessible to a wider range of laboratories. This era also saw improvements in column technology, such as the development of capillary columns, which increased resolution and efficiency. Additionally, advances in software enabled more robust data analysis and interpretation. In recent years, GCMS has continued to evolve with advancements in high-resolution mass spectrometry (HRMS), such as time-of-flight (TOF) and Orbitrap analyzers, which offer unparalleled mass resolution and accuracy. These developments have expanded the applications of GCMS into fields such as environmental monitoring, pharmaceutical analysis, and forensic science. Modern GCMS systems are also equipped with advanced automation features, making them more user-friendly and efficient for routine analyses. Throughout its development, GCMS has become an indispensable tool in various scientific disciplines due to its ability to separate, identify, and quantify complex mixtures with high precision. Its evolution is a testament to the collaborative efforts of scientists and engineers across multiple fields, leading to a powerful analytical technique that continues to shape our understanding of the chemical world.
How GCMS Works: Technical Aspects
Gas Chromatography-Mass Spectrometry (GCMS) is a powerful analytical technique that combines the separation capabilities of gas chromatography with the identification and quantification abilities of mass spectrometry. To understand how GCMS works, it is essential to delve into its technical aspects. This article will explore three key components: the principle of gas chromatography, the role of mass spectrometry in GCMS, and the process of data analysis and interpretation. The principle of gas chromatography involves separating volatile compounds based on their boiling points and affinity for the stationary phase, allowing for precise identification of individual components within a mixture. Mass spectrometry then takes these separated compounds and breaks them down into ions, which are analyzed to determine their mass-to-charge ratio, providing detailed information about the molecular structure. Finally, data analysis and interpretation involve processing the spectral data to identify and quantify the compounds present. By understanding these technical aspects, one can appreciate the robustness and reliability of GCMS in various scientific and industrial applications. Let's begin by examining the principle of gas chromatography, which forms the foundational step in the GCMS process.
Principle of Gas Chromatography
Gas Chromatography (GC) is a powerful analytical technique that separates, identifies, and quantifies the components of a mixture based on their boiling points and affinity for the stationary phase. The principle of GC revolves around the concept of partitioning, where a sample is vaporized and carried through a column by an inert gas, known as the mobile phase. Inside the column, the vaporized components interact with a stationary phase, which can be either a solid or a liquid. This interaction causes different components to travel at different speeds through the column, leading to their separation. The process begins with the injection of a sample into the GC instrument. The sample is vaporized in the injection port and mixed with the carrier gas, typically helium or nitrogen. This gas mixture then enters the column, where the components of the sample partition between the mobile phase (carrier gas) and the stationary phase. The components that have a higher affinity for the stationary phase will spend more time interacting with it, thus moving more slowly through the column. Conversely, components with lower affinity will move more quickly. As each component exits the column, it is detected by a detector, which generates a signal proportional to the concentration of the component. Common detectors include the Flame Ionization Detector (FID) and the Electron Capture Detector (ECD). The signals from these detectors are then processed by a computer, which generates a chromatogram—a plot of signal intensity versus time. Each peak in the chromatogram corresponds to a specific component in the sample, allowing for identification and quantification based on retention time and peak area. In addition to its analytical capabilities, GC can be coupled with Mass Spectrometry (MS) to form Gas Chromatography-Mass Spectrometry (GC-MS), enhancing its ability to identify complex mixtures. In GC-MS, the separated components from the GC column are ionized and fragmented in the MS instrument, producing mass spectra that provide detailed structural information about each component. This combination significantly increases the sensitivity and specificity of the analysis, making it an indispensable tool in various fields such as environmental monitoring, pharmaceutical research, and forensic science. Overall, the principle of gas chromatography relies on precise control over temperature, flow rates, and column properties to achieve efficient separation and detection of sample components. Its versatility and high resolution make it a cornerstone technique in modern analytical chemistry.
Role of Mass Spectrometry in GCMS
Mass spectrometry (MS) plays a pivotal role in Gas Chromatography-Mass Spectrometry (GCMS) by providing detailed molecular information about the components of a sample. In GCMS, the gas chromatograph separates the volatile components of a mixture based on their boiling points and affinity for the stationary phase. Once these components are separated, they are introduced into the mass spectrometer, where they are ionized, typically using electron ionization (EI) or chemical ionization (CI). The ions are then accelerated and separated according to their mass-to-charge ratio in the mass analyzer, which can be a quadrupole, time-of-flight (TOF), or ion trap analyzer. This separation allows for the identification of each component based on its unique mass spectrum, which serves as a fingerprint for that molecule. The mass spectrum is compared against databases of known spectra to identify the compounds present in the sample. Additionally, MS can provide quantitative data by measuring the abundance of ions corresponding to specific masses, enabling precise determination of concentrations. The integration of MS with GC enhances the analytical power by combining the high resolution of GC with the detailed molecular information provided by MS, making GCMS an indispensable tool in fields such as forensic science, environmental monitoring, pharmaceutical analysis, and food safety testing. Overall, the role of mass spectrometry in GCMS is to deliver accurate and comprehensive identification and quantification of sample components, thereby facilitating detailed chemical analysis and characterization.
Data Analysis and Interpretation
Data analysis and interpretation are crucial steps in the process of understanding and leveraging data from Gas Chromatography-Mass Spectrometry (GCMS). Following the technical aspects of how GCMS works, data analysis involves transforming raw data into meaningful insights. Here’s a concise overview: **Data Analysis:** 1. **Peak Identification:** The GCMS software identifies peaks in the chromatogram, each corresponding to a specific compound. These peaks are characterized by their retention time and intensity. 2. **Mass Spectra Interpretation:** For each peak, the mass spectrometer generates a mass spectrum, which is a plot of ion abundance versus mass-to-charge ratio. This spectrum is compared against databases (like NIST) to identify the compound. 3. **Quantification:** By integrating the area under each peak, the software quantifies the amount of each compound present in the sample. 4. **Data Cleaning:** Raw data may contain noise or artifacts; thus, filtering and smoothing techniques are applied to ensure accuracy. **Interpretation:** 1. **Compound Identification:** By matching mass spectra with database entries, analysts can identify unknown compounds in the sample. 2. **Concentration Determination:** Quantified data helps in determining the concentration of each compound, which is critical for various applications such as environmental monitoring, pharmaceutical analysis, and forensic science. 3. **Pattern Recognition:** Analysts look for patterns or trends in the data that could indicate specific processes or conditions (e.g., degradation products in a chemical reaction). 4. **Validation:** Results are validated against known standards to ensure accuracy and reliability. **Tools and Techniques:** 1. **Software Tools:** Advanced software like Agilent MassHunter or Thermo Fisher Scientific’s TraceFinder facilitate peak detection, integration, and spectral matching. 2. **Statistical Methods:** Techniques such as principal component analysis (PCA) and partial least squares regression (PLS) can be used to analyze complex datasets and identify correlations. 3. **Database Comparison:** Libraries like NIST and Wiley are used for spectral matching to identify compounds accurately. **Applications:** 1. **Environmental Monitoring:** GCMS helps in detecting pollutants and contaminants in water, air, and soil samples. 2. **Pharmaceuticals:** It is used for drug development, purity testing, and detecting impurities. 3. **Forensic Science:** GCMS aids in identifying substances in criminal investigations, such as narcotics or explosives. 4. **Food Safety:** It ensures the quality of food products by detecting additives, contaminants, or adulterants. In summary, data analysis and interpretation in GCMS involve meticulous steps from peak identification to compound quantification and validation. These processes are supported by sophisticated software tools and statistical methods, enabling precise identification and quantification of compounds across various fields.
Applications and Benefits of GCMS
Gas Chromatography-Mass Spectrometry (GCMS) is a powerful analytical technique that has revolutionized various fields by providing precise and detailed chemical analysis. This article delves into the diverse applications and benefits of GCMS, highlighting its significance in three key areas: Industrial and Environmental Applications, Medical and Pharmaceutical Uses, and Forensic Science and Law Enforcement. In industrial settings, GCMS is crucial for quality control, process optimization, and environmental monitoring. It helps in identifying and quantifying volatile organic compounds (VOCs) in air and water samples, ensuring compliance with regulatory standards. In medical and pharmaceutical contexts, GCMS aids in drug development, toxicology studies, and the detection of biomarkers for diseases. Its role in forensic science is equally vital, as it facilitates the identification of substances in crime scenes and helps in solving criminal cases. By exploring these applications, this article aims to illustrate the comprehensive utility of GCMS across different sectors. Let's begin by examining its industrial and environmental applications, where GCMS plays a pivotal role in maintaining environmental health and industrial efficiency.
Industrial and Environmental Applications
In the realm of industrial and environmental applications, Gas Chromatography-Mass Spectrometry (GCMS) plays a pivotal role in ensuring safety, compliance, and sustainability. This powerful analytical technique is indispensable for identifying and quantifying complex mixtures of chemicals, which is crucial in various sectors. In industrial settings, GCMS is used to monitor the quality of raw materials and finished products, detect contaminants, and optimize manufacturing processes. For instance, in the petrochemical industry, GCMS helps in analyzing crude oil and petroleum products to determine their composition and purity, thereby enhancing refining efficiency and product quality. In environmental monitoring, GCMS is a key tool for detecting pollutants in air, water, and soil samples. It aids in the identification of volatile organic compounds (VOCs), pesticides, heavy metals, and other hazardous substances that can have detrimental effects on ecosystems and human health. Regulatory agencies rely on GCMS data to enforce environmental standards and ensure compliance with laws such as the Clean Air Act and the Safe Drinking Water Act. Additionally, GCMS supports research into climate change by analyzing greenhouse gases and other atmospheric pollutants. The technique also finds extensive use in forensic science for analyzing evidence in criminal investigations. For example, it can identify accelerants in arson cases or detect drugs and poisons in biological samples. In the food industry, GCMS helps in detecting adulterants, ensuring food safety by identifying harmful additives or contaminants that could pose health risks to consumers. Moreover, GCMS is vital in pharmaceuticals for drug development and quality control. It helps in the identification of active pharmaceutical ingredients (APIs) and impurities, ensuring that drugs meet stringent regulatory standards. The ability of GCMS to provide detailed chemical profiles makes it an essential tool for research and development, enabling scientists to understand the metabolic pathways of drugs and their interactions with biological systems. Overall, the applications of GCMS in industrial and environmental contexts are diverse and critical. Its precision, sensitivity, and versatility make it an indispensable tool for maintaining product quality, ensuring environmental safety, and advancing scientific research across multiple disciplines. By providing accurate and detailed chemical analysis, GCMS supports informed decision-making and contributes significantly to public health and safety.
Medical and Pharmaceutical Uses
In the realm of medical and pharmaceutical applications, Gas Chromatography-Mass Spectrometry (GCMS) plays a pivotal role in ensuring the safety, efficacy, and quality of drugs and medical products. GCMS is instrumental in the identification and quantification of active pharmaceutical ingredients (APIs), excipients, and impurities within drug formulations. This technique allows for the precise analysis of complex mixtures, which is crucial for pharmaceutical development, where even trace amounts of contaminants can have significant implications on drug safety and efficacy. GCMS is widely used in drug discovery to screen for potential candidates by analyzing their metabolic pathways and identifying metabolites. It helps in understanding how drugs are metabolized in the body, which is essential for predicting their pharmacokinetic profiles. During the drug development phase, GCMS aids in the validation of analytical methods to ensure compliance with regulatory standards such as those set by the FDA and ICH. In clinical settings, GCMS is employed for toxicology screening to detect and quantify drugs of abuse, therapeutic drugs, and their metabolites in biological samples like blood, urine, and tissue. This capability is vital for diagnosing drug overdose cases and monitoring patient compliance with prescribed medications. Additionally, GCMS is used in forensic medicine to analyze biological samples for legal purposes, helping in criminal investigations involving drug-related crimes. The technique also finds extensive use in the quality control of pharmaceuticals. It helps in detecting adulterants and counterfeit drugs by identifying unknown substances through mass spectral libraries. This ensures that only safe and authentic products reach the market, protecting public health. Moreover, GCMS supports research in pharmacogenomics by analyzing genetic variations that affect drug metabolism. By identifying genetic markers associated with different metabolic pathways, researchers can tailor drug treatments to individual patients' genetic profiles, enhancing personalized medicine. In summary, GCMS is a cornerstone in medical and pharmaceutical applications due to its unparalleled ability to detect, identify, and quantify a wide range of compounds with high sensitivity and specificity. Its role spans from drug discovery and development to clinical diagnostics and forensic analysis, making it an indispensable tool for ensuring the quality and safety of medical products.
Forensic Science and Law Enforcement
Forensic science and law enforcement are intricately linked, with the former providing critical tools and techniques to aid in the latter's pursuit of justice. One of the most powerful instruments in this synergy is Gas Chromatography-Mass Spectrometry (GCMS). GCMS is a sophisticated analytical technique that separates, identifies, and quantifies the components of a mixture. In the context of forensic science, GCMS plays a pivotal role in various applications that significantly benefit law enforcement. **Applications in Forensic Science:** 1. **Drug Analysis:** GCMS is widely used to identify and quantify illicit drugs, helping law enforcement agencies to determine the presence and concentration of substances in seized materials or biological samples. 2. **Toxicology:** It aids in detecting toxins and poisons in biological fluids, which is crucial for investigating poisoning cases or overdoses. 3. **Fire Debris Analysis:** GCMS helps in identifying accelerants used in arson cases, allowing investigators to determine whether a fire was accidental or intentional. 4. **Explosives Analysis:** The technique is used to analyze residues from explosive materials, aiding in the investigation of bombings and other explosive incidents. 5. **Environmental Forensics:** GCMS can identify pollutants and contaminants in environmental samples, helping to trace the source of pollution and hold perpetrators accountable. **Benefits to Law Enforcement:** 1. **Enhanced Evidence Analysis:** GCMS provides precise and reliable data, which is essential for building strong cases against suspects. 2. **Speed and Efficiency:** Modern GCMS systems are highly automated, allowing for rapid analysis of samples and quick turnaround times, which can be critical in time-sensitive investigations. 3. **Non-Destructive Testing:** Many GCMS methods are non-destructive, preserving evidence for further analysis if needed. 4. **Courtroom Admissibility:** The results from GCMS are highly regarded in legal proceedings due to their accuracy and reliability, making them a powerful tool in securing convictions. 5. **Training and Standardization:** The widespread use of GCMS in forensic labs ensures that analysts are well-trained and that methods are standardized, reducing variability and increasing confidence in results. In summary, GCMS is a cornerstone of modern forensic science, offering law enforcement agencies a robust tool for analyzing evidence across a wide range of criminal investigations. Its precision, speed, and reliability make it an indispensable asset in the pursuit of justice, enabling investigators to gather critical evidence that can stand up to legal scrutiny. As technology continues to evolve, the applications and benefits of GCMS will only expand, further solidifying its role as a vital component of forensic science and law enforcement.