What Is Pure Substance
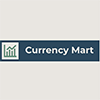
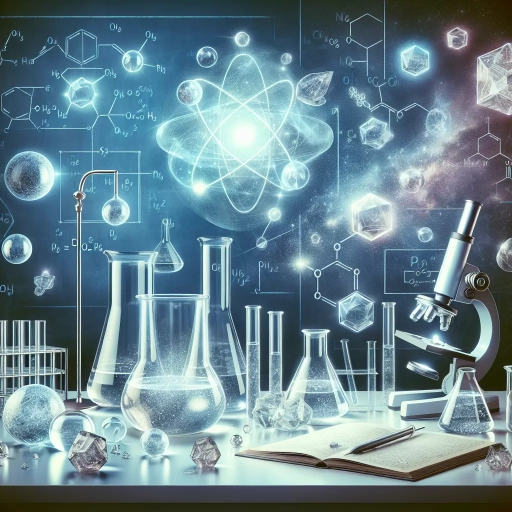
In the realm of chemistry, understanding the concept of pure substances is fundamental for both theoretical and practical applications. A pure substance is a material that consists of only one type of matter, whether it be an element or a compound, and it exhibits consistent properties throughout. This article delves into the intricacies of pure substances, starting with their definition and characteristics, which are crucial for distinguishing them from mixtures. We will explore the various types of pure substances, including elements and compounds, highlighting their unique properties and roles in different scientific contexts. Additionally, we will discuss the methods used for identifying and purifying these substances, which are essential in ensuring their purity and reliability in scientific experiments. By grasping these concepts, readers will gain a comprehensive understanding of what constitutes a pure substance. Let us begin by examining the definition and characteristics of pure substances, which form the cornerstone of this topic.
Definition and Characteristics of Pure Substances
Pure substances are fundamental in chemistry, characterized by their uniform composition and consistent properties. Understanding these substances involves delving into three key aspects: their chemical composition, physical properties, and how they distinguish from mixtures. The chemical composition of a pure substance is its defining feature, as it consists of only one type of molecule or atom. This uniformity is crucial for identifying and classifying substances. Additionally, the physical properties of pure substances, such as melting and boiling points, are consistent and predictable. These properties serve as benchmarks for identifying and distinguishing substances. Lastly, distinguishing pure substances from mixtures is essential, as mixtures can vary in composition and properties. By examining these three facets, we can gain a comprehensive understanding of what constitutes a pure substance. Let's begin by exploring the chemical composition of pure substances in more detail.
Chemical Composition
Chemical composition is a fundamental concept in understanding pure substances, as it refers to the specific proportion of elements that make up a compound. A pure substance, by definition, has a fixed and consistent chemical composition. This means that every sample of the same pure substance will contain the same elements in the same ratio, regardless of its source or method of preparation. For instance, water (H₂O) is a pure substance because it always consists of two hydrogen atoms and one oxygen atom per molecule. This consistent chemical composition is what distinguishes pure substances from mixtures, which can vary in their composition. The chemical composition of a pure substance can be expressed through its chemical formula, which provides a concise representation of the types and numbers of atoms present in a molecule. For example, the chemical formula for carbon dioxide is CO₂, indicating that one molecule of carbon dioxide contains one carbon atom and two oxygen atoms. This specificity in chemical composition ensures that the physical and chemical properties of a pure substance remain constant, such as its melting point, boiling point, and reactivity. Understanding the chemical composition of pure substances is crucial in various fields like chemistry, biology, and materials science. It allows scientists to predict and explain the behavior of substances under different conditions. For example, knowing the chemical composition of a substance helps in determining its solubility, density, and reactivity with other substances. This knowledge is essential for synthesizing new compounds, developing pharmaceuticals, and designing materials with specific properties. Moreover, the fixed chemical composition of pure substances enables precise analytical techniques such as spectroscopy and chromatography to identify and quantify the components of a sample. These methods rely on the unique spectral signatures or separation characteristics that are inherent to each element or compound, allowing for accurate identification and quantification. In summary, the chemical composition of a pure substance is its defining characteristic, ensuring uniformity in its properties and behavior. This concept is central to understanding the definition and characteristics of pure substances, providing a foundation for various scientific and practical applications.
Physical Properties
Physical properties are intrinsic characteristics of a substance that can be observed or measured without altering its chemical identity. These properties are crucial in defining and distinguishing pure substances from one another. Key physical properties include **melting and boiling points**, which are specific temperatures at which a substance changes state from solid to liquid and from liquid to gas, respectively. **Density**, the mass per unit volume of a substance, is another important physical property that helps in identifying substances. **Solubility**, which describes how well a substance dissolves in a solvent, is also significant. Additionally, **color**, **odor**, and **texture** provide immediate visual and sensory clues about the nature of a substance. Other critical physical properties include **viscosity**, which measures the resistance of a fluid to flow, and **surface tension**, which is the force acting along the surface of a liquid that causes it to behave as if it has an "elastic skin" at its surface. The **refractive index**, which indicates how much light bends when passing from one medium to another, is also a valuable property for identification. Furthermore, **electrical conductivity** and **thermal conductivity** describe how well a substance can conduct electricity and heat, respectively. These properties collectively form a unique fingerprint for each pure substance, allowing scientists to identify and distinguish them accurately. Understanding these physical properties is essential in various fields such as chemistry, physics, and engineering. For instance, knowing the melting and boiling points of a substance is vital in processes like purification and synthesis. Similarly, understanding solubility helps in selecting appropriate solvents for reactions or extractions. The precise measurement of these properties ensures that substances are pure and free from contaminants, which is critical in scientific research and industrial applications. In summary, physical properties are fundamental in defining pure substances by providing a set of measurable characteristics that are unique to each substance. These properties not only help in identification but also play a crucial role in various scientific and industrial processes, ensuring the purity and integrity of the substances involved.
Distinguishing from Mixtures
Distinguishing pure substances from mixtures is a fundamental concept in chemistry, crucial for understanding the properties and behaviors of different materials. A pure substance, such as an element or a compound, has a fixed composition and consistent properties throughout its entirety. In contrast, a mixture can be separated into its component parts by physical means and does not have a fixed composition. To distinguish between the two, several key characteristics can be observed. **Homogeneity** is a primary indicator; pure substances are homogeneous, meaning they have the same properties throughout, whereas mixtures can be either homogeneous (like solutions) or heterogeneous (like suspensions). For instance, water is a homogeneous pure substance because it has uniform properties everywhere, while air is a homogeneous mixture of gases. Another distinguishing factor is **separability**. Pure substances cannot be separated into simpler components by physical methods such as filtration, distillation, or magnetic separation. On the other hand, mixtures can often be separated using these methods. For example, a mixture of sand and water can be separated by filtration, but water itself cannot be separated into simpler components through physical means. **Chemical Composition** also plays a significant role. Pure substances have a definite chemical composition that can be expressed by a chemical formula. For example, the chemical formula for water is H₂O, indicating that it always consists of two hydrogen atoms and one oxygen atom per molecule. Mixtures do not have a fixed chemical composition; their components can vary in proportion. Additionally, **Physical Properties** such as melting and boiling points are consistent for pure substances but vary for mixtures. Pure substances exhibit sharp melting and boiling points because all molecules are identical and change state at the same temperature. Mixtures typically exhibit a range of melting and boiling points due to the presence of different components. Finally, **Reproducibility** of properties is another key differentiator. The properties of a pure substance are reproducible regardless of its source or method of preparation. This is not true for mixtures, where properties can vary significantly depending on the proportions of the components. In summary, distinguishing between pure substances and mixtures involves examining their homogeneity, separability, chemical composition, physical properties like melting and boiling points, and reproducibility of properties. These criteria help chemists identify whether a material is a uniform entity with consistent characteristics or a blend of different substances with variable properties. Understanding these distinctions is essential for accurately defining and characterizing pure substances in various scientific contexts.
Types of Pure Substances
Pure substances are fundamental in chemistry, characterized by their uniform composition and properties. Understanding these substances is crucial for various scientific and industrial applications. This article delves into the different types of pure substances, focusing on three key categories: Elements, Compounds, and Isotopes and Isomers. Elements are the simplest form of pure substances, consisting of only one type of atom. Compounds, on the other hand, are formed when elements combine in specific ratios, resulting in new substances with distinct properties. Isotopes and Isomers introduce complexity by showing how variations within elements and compounds can lead to different physical and chemical behaviors. By exploring these categories, we gain a comprehensive understanding of the diversity and intricacies within pure substances. Let's begin by examining the foundational building blocks: Elements.
Elements
Elements are the fundamental building blocks of matter, consisting of atoms that all have the same number of protons in their atomic nuclei. This uniformity in atomic structure defines an element and distinguishes it from other elements. Each element has a unique set of properties, such as atomic mass, electron configuration, and chemical reactivity, which are determined by the number of protons (atomic number) and electrons in its atoms. For instance, hydrogen (H) with one proton and one electron behaves differently from helium (He) with two protons and two electrons. Elements can exist in various forms: as gases (like oxygen and nitrogen), liquids (such as mercury), or solids (like carbon and iron). They can also be classified into different categories based on their properties, such as metals (e.g., copper and gold), nonmetals (e.g., carbon and oxygen), and metalloids (e.g., silicon and germanium). The periodic table of elements organizes these substances systematically, allowing for easy identification and comparison of their characteristics. Understanding elements is crucial because they form the basis of all matter and are essential for comprehending chemical reactions and the composition of compounds. In the context of pure substances, elements represent one of the two primary types, the other being compounds, which are formed by the chemical bonding of two or more different elements. The study of elements provides a foundational understanding of chemistry and is pivotal in various scientific and industrial applications.
Compounds
Compounds are a fundamental type of pure substance, characterized by their composition of two or more different elements chemically bonded together in a fixed ratio. Unlike mixtures, where the components can be separated by physical means, compounds cannot be broken down into their constituent elements without undergoing a chemical reaction. This distinction is crucial because it highlights the stability and uniformity of compounds. In a compound, the atoms of different elements are held together by strong chemical bonds, such as covalent, ionic, or metallic bonds. For instance, water (H₂O) is a compound composed of hydrogen and oxygen atoms in a 2:1 ratio. Similarly, sodium chloride (NaCl), commonly known as table salt, consists of sodium and chlorine atoms in a 1:1 ratio. These fixed ratios are a hallmark of compounds and distinguish them from mixtures, where the proportions of the components can vary. The formation of compounds often involves the sharing or transfer of electrons between atoms. In covalent compounds like water and methane (CH₄), atoms share pairs of electrons to form stable molecules. In ionic compounds like sodium chloride, electrons are transferred from one atom to another, resulting in the formation of ions that are electrostatically attracted to each other. Compounds exhibit properties that are distinct from those of their individual component elements. For example, the boiling point of water is 100°C at standard atmospheric pressure, whereas the boiling points of hydrogen and oxygen gases are -252.88°C and -182.96°C, respectively. This difference underscores the unique characteristics that arise from the chemical bonding in compounds. Moreover, compounds can be classified into various types based on their chemical structure and properties. Molecular compounds, such as carbon dioxide (CO₂) and ammonia (NH₃), consist of discrete molecules held together by intermolecular forces. Ionic compounds, like calcium carbonate (CaCO₃) and potassium nitrate (KNO₃), are composed of ions arranged in a crystalline lattice structure. The study of compounds is essential in various fields, including chemistry, biology, and materials science. Understanding the properties and behaviors of compounds is crucial for developing new materials, pharmaceuticals, and technologies. For instance, the discovery of new compounds with specific properties has led to advancements in fields such as medicine, where targeted therapies rely on the unique chemical properties of certain compounds. In summary, compounds are pure substances that consist of two or more elements chemically bonded together in fixed ratios. Their formation involves the sharing or transfer of electrons, resulting in unique properties that distinguish them from their component elements. The classification and study of compounds are vital for advancing our understanding of chemistry and its applications across various disciplines.
Isotopes and Isomers
Isotopes and isomers are fundamental concepts in chemistry that help us understand the diversity within pure substances. **Isotopes** are atoms of the same chemical element that have the same number of protons but differ in the number of neutrons in their atomic nuclei. This variation in neutron number leads to different atomic masses for these isotopes, yet they retain the same chemical properties due to their identical proton count. For example, carbon-12, carbon-13, and carbon-14 are isotopes of carbon, each with 6 protons but varying numbers of neutrons (6, 7, and 8 respectively). Isotopes can be stable or radioactive; stable isotopes do not undergo radioactive decay, while radioactive isotopes do. **Isomers**, on the other hand, are molecules that have the same molecular formula but differ in their structural arrangement of atoms. This difference can manifest in two main ways: **structural isomers** and **stereoisomers**. Structural isomers have different bond connections between their atoms, leading to distinct physical and chemical properties. For instance, ethanol (CH₃CH₂OH) and dimethyl ether (CH₃OCH₃) are structural isomers with the same molecular formula (C₂H₆O) but different structures. Stereisomers, including enantiomers and diastereomers, differ in the spatial arrangement of their atoms without altering the bond connections. Enantiomers are mirror images that cannot be superimposed on each other, much like left and right hands. Diastereomers are stereoisomers that are not mirror images of each other. Understanding isotopes and isomers is crucial for various fields such as chemistry, biology, medicine, and environmental science. In chemistry, recognizing these variations helps in synthesizing specific compounds with desired properties. In biology, isomers play a significant role in the structure and function of biomolecules like proteins and carbohydrates. In medicine, isotopes are used in diagnostic imaging techniques such as positron emission tomography (PET scans), while isomers are important in drug development where slight structural changes can significantly affect efficacy and safety. In summary, isotopes and isomers represent different levels of diversity within pure substances. Isotopes highlight variations at the atomic level due to differences in neutron numbers, while isomers reveal variations at the molecular level due to differences in atomic arrangement. These concepts are essential for a comprehensive understanding of pure substances and their applications across various scientific disciplines.
Identification and Purification Methods
In the realm of scientific research and industrial applications, the accurate identification and purification of substances are crucial steps that underpin a wide range of disciplines. These processes rely on a variety of sophisticated methods, each with its unique strengths and applications. This article delves into three key areas: Chemical Analysis Techniques, Physical Separation Methods, and Chromatography and Spectroscopy. Chemical Analysis Techniques involve the use of chemical reactions to identify and quantify the components of a sample, providing detailed information about its composition. Physical Separation Methods, on the other hand, exploit differences in physical properties such as density, size, and solubility to isolate substances. Lastly, Chromatography and Spectroscopy offer powerful tools for separating and identifying complex mixtures based on interactions between the sample and a stationary phase or electromagnetic radiation. By understanding these methodologies, scientists can ensure the purity and accuracy of their samples, which is essential for reliable research outcomes. This article begins by exploring Chemical Analysis Techniques, which form the foundation of many identification and purification processes.
Chemical Analysis Techniques
Chemical analysis techniques are pivotal in the identification and purification of substances, ensuring the accuracy and reliability of scientific findings. These methods involve various sophisticated tools and procedures designed to determine the composition, structure, and properties of chemical substances. **Spectroscopy**, for instance, encompasses a range of techniques such as Infrared (IR) spectroscopy, Nuclear Magnetic Resonance (NMR) spectroscopy, and Mass Spectrometry (MS), which provide detailed information about molecular structure and bonding. IR spectroscopy helps identify functional groups within a molecule by analyzing the absorption of infrared radiation, while NMR spectroscopy offers insights into the molecular environment of atoms, particularly hydrogen and carbon. Mass spectrometry, on the other hand, measures the mass-to-charge ratio of ions, aiding in the identification of molecular weight and fragmentation patterns. **Chromatography** is another essential technique used for both identification and purification. High-Performance Liquid Chromatography (HPLC) and Gas Chromatography (GC) are commonly employed to separate, identify, and quantify the components of a mixture. These methods leverage differences in physical and chemical properties such as boiling point, polarity, and affinity for a stationary phase to isolate pure substances from complex mixtures. **Titration**, a quantitative analytical technique, involves the reaction of a known amount of a reagent with the substance being analyzed to determine its concentration. This method is particularly useful for determining the purity of substances by measuring the amount of impurities present. **X-ray Crystallography** and **Electron Microscopy** provide structural information at the atomic and molecular level. X-ray crystallography involves scattering X-rays off the atoms within a crystalline solid to determine the three-dimensional arrangement of atoms within the crystal lattice. Electron microscopy, including Transmission Electron Microscopy (TEM) and Scanning Electron Microscopy (SEM), uses electron beams to produce high-resolution images of the surface morphology and internal structure of materials. **Thermal Analysis** techniques like Differential Scanning Calorimetry (DSC) and Thermogravimetric Analysis (TGA) are used to study the thermal properties of substances. DSC measures the heat flow associated with phase transitions such as melting or crystallization, while TGA quantifies changes in mass as a function of temperature, helping to identify decomposition points and purity levels. In addition to these advanced techniques, **Classical Wet Chemistry** methods such as precipitation reactions, acid-base titrations, and gravimetric analysis remain fundamental tools for identifying and purifying substances. These traditional methods often serve as preliminary steps before more sophisticated analyses are conducted. Overall, the integration of these chemical analysis techniques ensures that substances can be accurately identified and purified, which is crucial in various fields including pharmaceuticals, environmental science, and materials engineering. By combining multiple analytical approaches, scientists can achieve a comprehensive understanding of the chemical composition and properties of substances, thereby ensuring the highest standards of purity and reliability in their research and applications.
Physical Separation Methods
Physical separation methods are crucial techniques used in the identification and purification of substances, ensuring the isolation of pure components from mixtures. These methods leverage physical properties such as density, size, and magnetic properties to separate substances without altering their chemical composition. One of the most common physical separation methods is **filtration**, which involves passing a mixture through a porous material to separate solid particles from liquids or gases. This technique is widely used in laboratories and industrial settings to remove impurities and obtain a clearer solution. Another significant method is **distillation**, which exploits differences in boiling points to separate components of a mixture. This process involves heating the mixture until the components vaporize and then condensing them back into liquids at different temperatures, allowing for the collection of each component separately. Distillation is particularly useful for separating volatile substances and is a key process in industries such as petroleum refining and alcohol production. **Chromatography** is another powerful tool for physical separation, involving the distribution of substances between two phases: a stationary phase and a mobile phase. The mobile phase carries the mixture through the stationary phase, and different components interact differently with each phase, leading to their separation based on their affinities. Various types of chromatography, including paper chromatography, thin-layer chromatography, and gas chromatography, are employed depending on the nature of the substances being separated. **Centrifugation** and **sedimentation** are methods that utilize differences in density to separate particles. Centrifugation involves spinning a mixture at high speeds to force denser particles to the bottom of a container, while sedimentation relies on gravity to achieve the same effect over a longer period. These techniques are essential in biological research for separating cells, proteins, and other biological molecules. **Magnetic separation** takes advantage of the magnetic properties of certain materials to isolate them from non-magnetic components. This method is particularly useful in mineral processing and recycling industries where magnetic materials need to be separated from non-magnetic ones. **Crystallization** is a technique that involves dissolving a substance in a solvent and then allowing it to crystallize out as the solution cools or evaporates. This method is highly effective for purifying solids because crystals formed during this process tend to be free from impurities, making it a cornerstone in the production of pharmaceuticals and other high-purity chemicals. In summary, physical separation methods are indispensable in the identification and purification of substances by exploiting their physical properties. These techniques not only ensure the isolation of pure components but also play critical roles in various scientific and industrial applications, underscoring their importance in maintaining purity and quality in diverse fields.
Chromatography and Spectroscopy
Chromatography and spectroscopy are pivotal techniques in the identification and purification of substances, ensuring the isolation of pure compounds from complex mixtures. **Chromatography** involves the separation of components based on their differential distribution between two phases: a stationary phase and a mobile phase. This method can be categorized into various types, such as gas chromatography (GC), liquid chromatography (LC), and paper chromatography, each tailored to specific types of samples. For instance, GC is ideal for volatile compounds, while LC is more versatile and can handle a wide range of substances. Chromatography not only separates but also quantifies the components present in a mixture, making it an indispensable tool in analytical chemistry. **Spectroscopy**, on the other hand, is a method that relies on the interaction between matter and electromagnetic radiation to identify and quantify substances. Key spectroscopic techniques include infrared (IR) spectroscopy, nuclear magnetic resonance (NMR) spectroscopy, mass spectrometry (MS), and ultraviolet-visible (UV-Vis) spectroscopy. IR spectroscopy helps in identifying functional groups within molecules by analyzing the absorption of infrared radiation. NMR spectroscopy provides detailed information about the molecular structure by measuring the interaction of nuclear spins with magnetic fields. MS determines the mass-to-charge ratio of ions, allowing for precise molecular weight determination and structural elucidation. UV-Vis spectroscopy measures the absorption of light in the ultraviolet and visible regions, which is useful for identifying conjugated systems and quantifying concentrations. The synergy between chromatography and spectroscopy enhances their analytical power. For example, combining GC or LC with MS (GC-MS or LC-MS) allows for both separation and identification of components in a single run, significantly improving the accuracy and efficiency of analysis. These combined techniques are crucial in various fields such as pharmaceuticals, environmental monitoring, and forensic science, where the purity and identity of substances are paramount. By leveraging these advanced methods, scientists can ensure that substances are not only purified but also accurately identified, which is essential for maintaining high standards in research and industry. In summary, chromatography and spectroscopy form the backbone of modern analytical chemistry, enabling precise identification and purification of substances to achieve the highest levels of purity and accuracy.