What Is Soeed
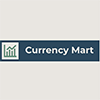
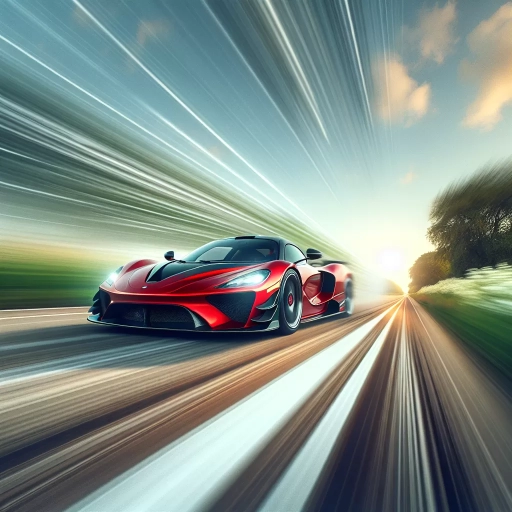
Speed, a fundamental concept in physics, is a measure of how fast an object moves or covers a distance over time. It is a critical aspect of our daily lives, influencing everything from transportation and technology to sports and everyday activities. Understanding speed is not just about recognizing its numerical value; it involves delving into its underlying principles, scientific mechanisms, and practical applications. This article will explore the multifaceted nature of speed by first examining the concept itself, defining what speed is and how it is measured. We will then delve into the science behind speed, discussing the laws of motion and the forces that govern it. Finally, we will look at the various applications and implications of speed across different fields, highlighting its impact on modern society. By grasping these dimensions, readers will gain a comprehensive understanding of speed and its significance. Let us begin by understanding the concept of speed in its most basic form.
Understanding the Concept of Speed
Understanding the concept of speed is a fundamental aspect of physics and engineering, with far-reaching implications in various fields such as transportation, sports, and technology. To grasp this concept fully, it is essential to delve into three key areas: the definition and measurement of speed, the different types of speed, and the historical development of speed measurement. Firstly, defining and measuring speed provides the foundational knowledge necessary for any further exploration. This involves understanding the basic units of measurement, such as meters per second or miles per hour, and the methods used to calculate these values. Secondly, recognizing the various types of speed—average, instantaneous, and relative—allows for a more nuanced understanding of how speed can be applied in different contexts. For instance, average speed is crucial for long-distance travel, while instantaneous speed is vital in real-time applications. Lastly, examining the historical development of speed measurement highlights the evolution of scientific tools and methodologies over time. From ancient civilizations to modern-day technologies, this historical perspective underscores the continuous quest for precision and accuracy in measuring speed. By exploring these facets, we can gain a comprehensive understanding of speed that is both informative and engaging. Let us begin by examining the definition and measurement of speed, which forms the bedrock of our understanding.
Definition and Measurement
**Definition and Measurement** To grasp the concept of speed, it is crucial to understand its definition and how it is measured. Speed, in its simplest form, is a scalar quantity that represents the rate of change of an object's position with respect to time. Unlike velocity, which is a vector quantity that includes both magnitude and direction, speed focuses solely on the magnitude of the rate of change. This distinction is vital because it allows us to analyze motion without considering the direction in which an object is moving. The measurement of speed involves calculating the distance traveled by an object over a specific period. Mathematically, speed (\(v\)) is defined as the ratio of distance (\(d\)) to time (\(t\)), expressed as \(v = \frac{d}{t}\). This formula underscores that speed is directly proportional to the distance covered and inversely proportional to the time taken. For instance, if a car travels 100 kilometers in 2 hours, its average speed would be 50 kilometers per hour. In practical terms, measuring speed can be done using various methods depending on the context. For everyday applications, such as driving or cycling, speedometers are commonly used. These devices measure the rotational speed of wheels and convert it into linear speed. In scientific and engineering contexts, more precise instruments like radar guns or GPS trackers may be employed to measure speed accurately. Understanding the concept of speed also involves recognizing different types of speeds. Instantaneous speed refers to the speed at a specific moment in time, while average speed is the total distance divided by the total time taken over a given interval. This distinction is important because instantaneous speed can vary significantly over time due to factors like acceleration or deceleration. Moreover, the unit of measurement for speed varies depending on the system being used. In the International System of Units (SI), speed is typically measured in meters per second (m/s), while in everyday applications, kilometers per hour (km/h) or miles per hour (mph) are more common. The choice of unit often depends on the context and the precision required. In conclusion, understanding the definition and measurement of speed is foundational to comprehending its role in various fields such as physics, engineering, and even daily life. By recognizing how speed is calculated and the different types of speeds that exist, one can better analyze and interpret motion in various scenarios. This foundational knowledge not only enhances our understanding of speed but also provides a robust framework for exploring more complex concepts related to motion and dynamics.
Types of Speed (Average, Instantaneous, Relative)
Understanding the concept of speed is crucial in various fields, including physics, engineering, and everyday life. Within this broader context, there are several types of speed that provide different insights into how objects move. **Average Speed** is a measure of the total distance traveled divided by the total time taken. It gives an overall picture of an object's journey but does not account for variations in speed during the trip. For instance, if a car travels 200 miles in 4 hours, its average speed is 50 miles per hour, regardless of whether it was moving at a constant speed or had periods of acceleration and deceleration. **Instantaneous Speed**, on the other hand, is the speed of an object at a specific moment in time. It reflects the exact rate at which an object is moving at that instant and can be determined using tools like speedometers in vehicles or radar guns in traffic enforcement. Unlike average speed, instantaneous speed captures the dynamic nature of motion, providing real-time data that can be critical in situations requiring precise control or measurement. **Relative Speed** introduces the concept of motion relative to another frame of reference. This type of speed is essential when considering the interaction between two or more moving objects. For example, when two cars are approaching each other, their relative speed is the sum of their individual speeds if they are moving directly towards each other. In aviation, relative speed is crucial for pilots to understand their velocity relative to air currents or other aircraft to ensure safe navigation. Each type of speed serves a distinct purpose and offers unique information about an object's motion. Average speed provides a general overview suitable for long-term analysis or planning, while instantaneous speed offers immediate feedback necessary for real-time adjustments. Relative speed, meanwhile, helps in understanding complex interactions between multiple moving entities. By grasping these different types of speed, one can better comprehend and analyze various aspects of motion, whether in scientific research, engineering applications, or everyday activities like driving or flying. This nuanced understanding enhances our ability to predict, control, and optimize movement in diverse contexts.
Historical Development of Speed Measurement
The historical development of speed measurement is a fascinating journey that spans centuries, reflecting human ingenuity and the relentless pursuit of precision. The concept of speed, though seemingly straightforward, has evolved significantly over time, influenced by technological advancements, scientific discoveries, and practical needs. In ancient civilizations, such as Greece and Rome, speed was often measured through simple observations and comparisons. For instance, the speed of a chariot or a runner could be estimated relative to the time it took to cover a known distance. The Greek philosopher Aristotle (384-322 BCE) discussed the concept of speed in his works, laying some of the foundational philosophical groundwork for later scientific inquiry. The Middle Ages saw little significant progress in speed measurement due to the lack of sophisticated tools. However, with the advent of the Renaissance and the Scientific Revolution in the 16th and 17th centuries, new methods began to emerge. Galileo Galilei (1564-1642) is credited with one of the earliest systematic studies on motion and speed. He used inclined planes to measure the acceleration of rolling balls, thereby introducing quantitative methods into the study of motion. The invention of mechanical clocks in the 17th century provided a reliable means to measure time accurately, which was crucial for calculating speed. Sir Isaac Newton (1643-1727) built upon Galileo's work and formulated his laws of motion, which included the concept of velocity as a vector quantity. These laws laid the foundation for modern physics and paved the way for more precise measurements. In the 19th century, the development of railroads and steam engines created a pressing need for accurate speed measurements. This led to the invention of various mechanical devices such as odometers and tachometers. The tachometer, which measures rotational speed, became particularly important in industrial applications. The 20th century saw a significant leap forward with the advent of electronic and digital technologies. Radar guns, introduced in the mid-20th century, allowed law enforcement to measure vehicle speeds accurately using Doppler radar principles. Later advancements included GPS technology, which enables precise speed measurements by tracking changes in position over time. Today, speed measurement is more sophisticated than ever before. Modern vehicles are equipped with electronic speedometers that provide real-time data on velocity. In sports and athletics, high-speed cameras and timing systems allow for precise measurements down to fractions of a second. In scientific research, advanced sensors and data acquisition systems enable researchers to measure speeds in various contexts, from fluid dynamics to particle physics. Throughout its historical development, the measurement of speed has been driven by both practical needs and scientific curiosity. From ancient observations to modern technological marvels, our ability to quantify speed has become increasingly precise and widespread. This evolution not only reflects our growing understanding of the physical world but also underscores the importance of speed in various aspects of human life—from transportation and sports to scientific inquiry and technological innovation.
The Science Behind Speed
The science behind speed is a multifaceted field that delves into the intricate mechanisms governing how objects move. At its core, understanding speed requires a deep dive into the physics of motion and velocity, which lays the foundational principles for comprehending how objects change their position over time. However, speed is not isolated from external influences; factors such as friction, gravity, and aerodynamics play crucial roles in determining an object's velocity. Additionally, the relationship between energy and work is pivotal in understanding the dynamics of speed, as it explains how forces applied to an object translate into its motion. By examining these three key aspects—physics of motion and velocity, factors influencing speed, and energy and work in relation to speed—we can gain a comprehensive understanding of what drives and hinders the movement of objects. Let's begin by exploring the fundamental principles of physics that underpin our understanding of motion and velocity.
Physics of Motion and Velocity
**The Physics of Motion and Velocity** Understanding the physics of motion and velocity is crucial for grasping the fundamental principles behind speed. Motion, in its simplest form, is the change in position of an object with respect to time. This concept is deeply intertwined with velocity, which quantifies both the speed and direction of an object's motion. Velocity is a vector quantity, meaning it has both magnitude (speed) and direction. For instance, if a car travels at 60 miles per hour northward, its speed is 60 miles per hour, but its velocity includes both this speed and the direction north. The study of motion and velocity falls under kinematics, a branch of classical mechanics that describes the motion of objects without considering the forces that cause this motion. Kinematic equations, such as \(v = v_0 + at\) and \(s = v_0t + \frac{1}{2}at^2\), where \(v\) is final velocity, \(v_0\) is initial velocity, \(a\) is acceleration, \(t\) is time, and \(s\) is displacement, provide mathematical tools to analyze and predict the motion of objects. These equations are essential in understanding how velocity changes over time under constant acceleration. Acceleration itself is the rate of change of velocity and is also a vector quantity. It can be positive (increasing velocity), negative (decreasing velocity), or zero (constant velocity). For example, when a car accelerates from rest to a certain speed, its acceleration is positive; when it decelerates to come to a stop, its acceleration is negative. In real-world applications, understanding motion and velocity is vital. In automotive engineering, for instance, knowing how a vehicle accelerates and decelerates is crucial for designing safe braking systems and optimizing performance. Similarly, in aerospace engineering, precise calculations of velocity and acceleration are necessary for navigating spacecraft through complex trajectories. Moreover, the concept of relative motion plays a significant role in understanding velocity. Relative motion refers to the motion of one object as observed from another moving frame of reference. This concept is particularly important in high-speed phenomena like jet travel or space exploration where relative velocities between different frames of reference must be accurately calculated to ensure safe and efficient travel. In conclusion, the physics of motion and velocity forms the backbone of understanding speed. By delving into kinematics and the principles governing acceleration and relative motion, we gain a deeper insight into how objects move and interact within our universe. This knowledge not only enhances our comprehension of natural phenomena but also drives technological advancements across various fields, making it an indispensable part of scientific inquiry and innovation.
Factors Influencing Speed (Friction, Gravity, Aerodynamics)
When delving into the science behind speed, it is crucial to understand the various factors that influence it. Among these, friction, gravity, and aerodynamics play pivotal roles in determining how fast an object can move. **Friction** is a force that opposes motion between any surfaces that are in contact. There are different types of friction—static, kinetic, and rolling—but all of them act to slow down or resist the movement of an object. For instance, in automotive engineering, reducing friction between tires and the road surface can significantly enhance speed by minimizing energy loss. Similarly, in high-speed applications like Formula 1 racing, specialized tires and lubricants are used to minimize frictional forces. **Gravity** is another fundamental force that affects speed. On Earth's surface, gravity pulls objects downward with a constant acceleration of approximately 9.81 meters per second squared. This gravitational force limits the maximum speed achievable by any object moving on or near the Earth's surface. However, gravity also plays a role in downhill motion; for example, skiers and cyclists can achieve high speeds by leveraging gravitational potential energy as they descend slopes. **Aerodynamics**, the study of air and its interaction with solid objects in motion, is particularly significant for high-speed phenomena. Air resistance or drag acts as a braking force that slows down moving objects. The shape and design of an object can greatly influence its aerodynamic efficiency; streamlined shapes reduce drag by allowing air to flow smoothly around them. In aviation and automotive industries, aerodynamic design is critical for achieving optimal speeds. For example, aircraft are designed with sleek profiles to cut through air efficiently, while race cars use spoilers and air dams to manage airflow and reduce drag. The interplay between these factors—friction, gravity, and aerodynamics—determines the ultimate speed an object can attain. In high-performance applications such as space exploration or professional sports, understanding and mitigating these forces are essential for achieving record-breaking speeds. For instance, spacecraft must overcome Earth's gravitational pull to reach orbit or escape velocity, while athletes in sports like cycling or skiing must optimize their equipment and techniques to minimize friction and maximize aerodynamic efficiency. In conclusion, the science behind speed is deeply intertwined with the dynamics of friction, gravity, and aerodynamics. By understanding how these forces interact with moving objects, engineers and scientists can design more efficient systems that push the boundaries of speed in various fields. Whether it's developing faster cars or more agile aircraft, mastering these fundamental principles is key to unlocking new levels of performance and innovation.
Energy and Work in Relation to Speed
**Energy and Work in Relation to Speed** The intricate relationship between energy, work, and speed is a fundamental aspect of understanding the science behind speed. At its core, speed is a measure of how quickly an object moves over a certain distance. However, to achieve and maintain speed, an object must expend energy. This energy expenditure is directly linked to the concept of work, which is defined as the force applied to an object multiplied by the distance over which that force is applied. When an object accelerates from rest to a higher speed, it requires a significant amount of energy to overcome its initial inertia. This energy can come from various sources such as chemical reactions in engines, electrical power, or even human muscle power. The work done on the object translates into kinetic energy—the energy of motion—which increases as the object gains speed. According to the principle of conservation of energy, the total energy of an isolated system remains constant; thus, any increase in kinetic energy must be balanced by a corresponding decrease in another form of energy, such as potential energy or thermal energy. The efficiency with which work is converted into kinetic energy plays a crucial role in determining an object's speed. For instance, in a car engine, only a fraction of the chemical energy stored in gasoline is converted into kinetic energy; the rest is lost as heat and friction. Similarly, in human locomotion, muscles convert chemical energy from food into mechanical work, but some of this energy is dissipated as heat due to inefficiencies in muscle function. Moreover, the relationship between energy and speed is not linear; it follows a quadratic relationship. According to Newton's second law of motion and the equation for kinetic energy (\(KE = \frac{1}{2}mv^2\)), where \(m\) is mass and \(v\) is velocity, doubling an object's speed requires four times the kinetic energy. This explains why achieving high speeds requires significantly more energy than achieving moderate speeds. In real-world applications, understanding this relationship is critical for optimizing performance. For example, in aerospace engineering, minimizing mass while maximizing power output is essential for achieving high speeds efficiently. In sports science, athletes and coaches focus on improving muscle efficiency and technique to convert more of their energy into forward motion rather than heat. In conclusion, the interplay between energy, work, and speed is a complex yet fascinating area of study. By grasping these principles, we can better appreciate how various systems—from simple mechanical devices to complex biological organisms—generate and utilize energy to achieve motion at different speeds. This knowledge not only enhances our understanding of the natural world but also informs technological innovations and performance optimizations across diverse fields.
Applications and Implications of Speed
The concept of speed has revolutionized various aspects of modern life, from the way we travel to the pace at which economies grow. In this article, we delve into the multifaceted applications and implications of speed, exploring three critical dimensions: Transportation and Technology Advancements, Economic and Social Impact of Speed, and Safety Considerations and Speed Limits. Firstly, advancements in transportation technology have significantly increased travel speeds, transforming how we move people and goods. High-speed trains, advanced aircraft, and even the development of electric vehicles have not only reduced travel times but also enhanced efficiency and comfort. These innovations have been pivotal in connecting global communities and fostering economic growth. Secondly, the economic and social impact of speed is profound. Faster communication networks and data transfer rates have enabled real-time global interactions, boosting international trade and collaboration. However, this increased pace also brings challenges such as stress and the need for continuous adaptation. Lastly, safety considerations and speed limits are crucial in balancing the benefits of speed with public safety. While higher speeds can lead to increased productivity, they also raise the risk of accidents. Therefore, understanding the optimal speed limits for different environments is essential to mitigate these risks. Transitioning to our first supporting section, we will examine **Transportation and Technology Advancements** in greater detail, highlighting how these innovations have reshaped our world and what future developments might hold.
Transportation and Technology Advancements
The intersection of transportation and technology has revolutionized the way we travel, significantly enhancing speed, efficiency, and safety. One of the most profound advancements is the development of high-speed rail networks. Countries like Japan, China, and France have implemented bullet trains that can reach speeds of over 300 kilometers per hour, drastically reducing travel times between cities. For instance, the Shanghai Maglev Train in China can travel from Shanghai Pudong International Airport to the city center in just 7 minutes and 20 seconds, covering a distance of 30 kilometers. This technology not only saves time but also reduces carbon emissions compared to air travel. Another significant area of innovation is in the realm of autonomous vehicles. Self-driving cars and trucks, equipped with advanced sensors, GPS, and artificial intelligence, are being tested and implemented globally. These vehicles promise to enhance road safety by minimizing human error, which is responsible for the majority of accidents. Companies like Waymo and Tesla are at the forefront of this technology, with Waymo already offering autonomous taxi services in select areas. Autonomous vehicles also have the potential to optimize traffic flow, reducing congestion and increasing the overall speed of transportation. The integration of smart traffic management systems is another key advancement. These systems use real-time data analytics and IoT sensors to monitor and manage traffic flow dynamically. For example, intelligent traffic lights can adjust their timing based on current traffic conditions, reducing wait times and improving the flow of vehicles. Cities like Singapore and London have implemented such systems, resulting in noticeable reductions in traffic congestion and travel times. In addition, advancements in air travel technology are pushing the boundaries of speed. Supersonic flight, which was last seen with the Concorde in the early 2000s, is being revisited with new designs that aim to reduce noise pollution and increase efficiency. Companies like Boom Supersonic are working on aircraft that could travel at speeds up to Mach 2.2 (around 2,700 km/h), potentially cutting travel times between continents by more than half. Furthermore, the rise of electric vehicles (EVs) is transforming personal transportation. EVs offer faster acceleration and smoother performance compared to traditional gasoline-powered cars. Brands like Tesla have made EVs mainstream, with models like the Tesla Model S capable of going from 0 to 100 km/h in under 3 seconds. This rapid acceleration enhances the driving experience while also contributing to a cleaner environment. Lastly, the development of hyperloop systems promises to revolutionize long-distance travel. Proposed by Elon Musk, hyperloop technology involves transporting passengers in vacuum-sealed tubes at speeds of up to 1,200 km/h. While still in its experimental phase, this technology has the potential to make travel between cities faster than flying, with significantly lower energy consumption. In summary, the convergence of transportation and technology has led to unprecedented advancements in speed, efficiency, and sustainability. From high-speed rail networks and autonomous vehicles to smart traffic management systems and innovative air travel technologies, these developments are reshaping how we move around the world. As these technologies continue to evolve, they will have profound implications for our daily lives, economic productivity, and environmental sustainability.
Economic and Social Impact of Speed
The economic and social impact of speed is multifaceted and profound, influencing various aspects of modern life. Economically, speed enhances productivity and efficiency across industries. In manufacturing, faster production lines and logistics systems reduce costs and increase output, allowing businesses to meet demand more effectively and maintain competitiveness. This ripple effect extends to global trade, where quicker transportation methods such as high-speed rail and air freight facilitate the rapid movement of goods, fostering international commerce and economic growth. Additionally, the advent of high-speed internet has revolutionized the service sector by enabling real-time communication, remote work, and e-commerce, thereby expanding market reach and customer accessibility. Socially, speed has transformed the way people live and interact. High-speed transportation networks like highways and bullet trains have reduced travel times, making it easier for people to commute to work, visit family, or explore new destinations. This increased mobility has contributed to urbanization and the development of megacities, where diverse populations converge to seek better opportunities. Furthermore, the speed of digital communication through social media and messaging apps has bridged geographical gaps, enabling instant connections and fostering global communities. However, this rapid pace of life also comes with challenges such as increased stress levels, decreased attention spans, and potential social isolation despite increased connectivity. Moreover, the pursuit of speed has driven technological innovation, leading to breakthroughs in fields like materials science, aerodynamics, and computer engineering. For instance, advancements in high-speed computing have enabled complex simulations that aid in medical research, weather forecasting, and financial modeling. These technological advancements not only drive economic growth but also improve the quality of life by providing better healthcare outcomes, more accurate weather predictions, and more stable financial systems. However, there are also negative consequences associated with the relentless pursuit of speed. Environmental concerns arise from the increased energy consumption and pollution generated by high-speed transportation and industrial processes. Additionally, the emphasis on speed can lead to safety compromises, as seen in accidents involving high-speed vehicles or the neglect of safety protocols in fast-paced work environments. Balancing the benefits of speed with these risks is crucial for sustainable development. In conclusion, the economic and social impact of speed is a double-edged sword. While it drives economic growth, enhances productivity, and connects people globally, it also poses significant challenges related to environmental sustainability, safety, and social well-being. As society continues to value speed, it is essential to implement measures that mitigate its negative effects while maximizing its benefits. This balanced approach will ensure that the pursuit of speed contributes positively to both economic prosperity and social harmony.
Safety Considerations and Speed Limits
When discussing the applications and implications of speed, it is crucial to delve into the safety considerations and speed limits that govern various environments. Speed, while a fundamental aspect of modern life, must be balanced with safety to prevent accidents and ensure the well-being of individuals. In transportation, for instance, speed limits are meticulously set based on road conditions, traffic volume, and the presence of pedestrians or other vulnerable users. These limits are not arbitrary; they are derived from extensive research and data analysis to minimize the risk of collisions. For example, urban areas typically have lower speed limits due to the higher density of pedestrians, cyclists, and intersections, whereas highways have higher limits due to their design for faster travel with fewer obstacles. In addition to road safety, speed considerations extend to other domains such as aviation and maritime transport. In aviation, strict speed regulations are enforced during takeoff, landing, and cruising phases to ensure safe distances between aircraft and to manage air traffic efficiently. Similarly, in maritime transport, speed limits are imposed in certain zones to protect marine life and prevent accidents in congested waterways. These regulations are often enforced through a combination of technological monitoring systems and human oversight. The enforcement of speed limits also involves technological advancements such as radar guns, speed cameras, and intelligent transportation systems (ITS). These tools not only monitor compliance but also provide real-time data that can be used to optimize traffic flow and reduce congestion. Furthermore, public awareness campaigns play a significant role in promoting safe driving practices by educating drivers about the dangers of excessive speed and the importance of adhering to speed limits. Beyond enforcement mechanisms, there is a growing emphasis on designing infrastructure that inherently promotes safe speeds. This includes features like roundabouts, speed bumps, and narrowed traffic lanes which physically limit the speed at which vehicles can travel. Such design elements are particularly effective in urban settings where pedestrian safety is paramount. Moreover, vehicle manufacturers are increasingly incorporating safety features into their designs that help manage speed. Advanced driver-assistance systems (ADAS) such as adaptive cruise control and automatic emergency braking can adjust vehicle speed based on road conditions and traffic patterns. These technologies not only enhance safety but also contribute to a smoother driving experience by reducing the need for constant manual adjustments. In conclusion, the interplay between speed and safety is complex and multifaceted. Effective speed management requires a holistic approach that includes stringent regulations, advanced technology, public education, and thoughtful infrastructure design. By understanding these safety considerations and adhering to established speed limits, we can significantly reduce the risk of accidents and create safer environments for all users of our transportation systems. This balanced approach ensures that the benefits of speed are realized without compromising on safety, thereby enhancing overall quality of life.