What Does An Atom Look Like
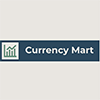
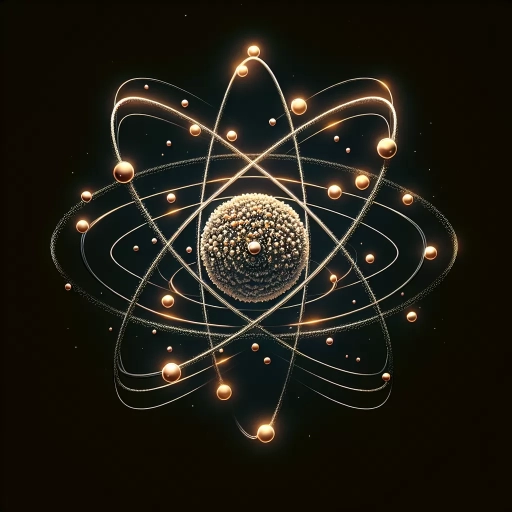
Understanding the Basic Structure of an Atom
Understanding the basic structure of an atom is like diving into the heart of the universe's building blocks. This complex yet fascinating world interweaves physics, chemistry, and the wonders of scientific exploration. The atom, seemingly simple in its definition, is composed of three essential components. Namely, these are the protons, neutrons, and electrons, each with their unique characteristics and tasks. This article will guide you on a microscopic journey to the core of an atomic structure, explicating the multifaceted nature of protons, neutrons, and electrons—the heartbeat of every atom. Subsequently, we will navigate towards the atomic nucleus—a packed bundle of protons and neutrons that dictate the atom's identity. This dense core symbolizes the heart of an atom, a potent force holding everything together. Beyond the nucleus, a vast space known as the electron cloud comes into perspective. Here, electrons exist, dancing around in an area that governs the atom's interactions with the rest of the universe. As we transition to the core components of an atom, we begin to see how these three elements—protons, neutrons, and electrons—interlock in a delicate balance to form the foundation of all matter. Let's begin this atomic adventure.
The Core Components of an Atom: Protons, Neutrons, and Electrons
Understanding the fundamental structure of an atom, it is essential to focus on its core components: protons, neutrons, and electrons. These tiny subatomic particles form the building blocks of matter and govern the properties and behavior of atoms. Protons carry a positive charge and reside in the atom's nucleus; their positive charge dictates the atomic number of an atom. For example, Hydrogen has one proton, awarding it the first place on the Periodic Table. Consequently, the number of protons in an atom also defines its identity. Therefore, if you change the number of protons in an atom, you are fundamentally forming a new atom. Neutrons, also housed in the nucleus, possess no charge at all, hence the name 'neutron'. They play a crucial role in the atom's mass, as a combination of both protons and neutrons contribute to an atom's atomic mass. Neutrons also possess another critical function: they aid in the atom's overall stability. This is because the presences of neutrons between protons in the nucleus help to overcome the vehement repulsion generated due to the same charges of the protons. Finally, in the surrounding area of the nucleus, there are negatively charged particles, known as electrons. They help balance the charge of the protons, rendering the atoms themselves generally neutral. Beyond just neutralizing charge, electrons are also vital because they participate in chemical reactions and formulate atomic bonds. In essence, they are the workers of the atom, interacting and exchanging with other atoms, facilitating every chemical phenomenon we know. In summary, while protons, neutrons, and electrons may be unimaginably minuscule, their existence and behaviour manifest in virtually everything around us, from the air we breathe to the stars we gawk at in the night sky. Understanding them is like possessing the keys to unlock the secrets of matter and how our universe works.
The Atomic Nucleus: The Center of an Atom
The Atomic Nucleus: The Center of an Atom The atomic nucleus stands at the heart of an atom, bearing a significant level of influence over the atom's behavior, characteristics, and properties. Although minuscule - existing as a one-hundred-thousandth the size of the atom itself - the atomic nucleus wields considerable sway over atom's identity. At its core, the atomic nucleus is a dense region composed primarily of two types of subatomic particles: protons and neutrons. The number of protons within the nucleus, also known as the atomic number, plays a pivotal role in deciding the type of atom: an oxygen atom encompasses 8 protons, whereas a carbon atom consists of 6. This fundamental information defines the atom's place in the periodic table, bestowing upon it unique attributes and behaviors. Neutrons, on the other hand, provides balance within the nucleus, as they lack any electrical charge and serve to stabilize the often volatile environment within the nucleus. The protons, bearing a positive charge, would naturally repel each other; neutron’s presence mitigates this conflict, preventing the protons from ejecting each other out of the nucleus and thereby maintaining atomic stability. The importance of the atomic nucleus goes beyond basic atomic structure. It’s the depository of virtually all the atom's mass. Despite its tiny size, the nucleus houses more than 99.9% of an atom’s total mass. This is because protons and neutrons, densely packed within the nucleus, are significantly heavier than electrons, which orbit ironically at a distance. This is a fascinating aspect of nuclear physics, and the study of the atomic nucleus offers crucial insights into the nature of matter at its most basic level. The behavior of the atomic nucleus also holds sway over nuclear reactions such as fusion and fission, which power our sun and nuclear power plants, respectively. When under specific conditions, the nucleus undergoes alterations that release a tremendous amount of energy - intrinsically linked to the principles underlying atomic bombs and nuclear energy. Therefore, the atomic nucleus, central to the atom, holds the key to atomic identity, stability, energy production, and scientific exploration, making it an enchanting topic in the journey of understanding basic atomic structure.
The Electron Cloud: The Surrounding Space of an Atom
Delving deeper into the structure of the atom, let’s explore an essential component known as the ‘Electron Cloud.’ This intricate component surrounds the nucleus of an atom, playing a pivotal role in various atomic interactions. Imagine a bustling city enclosed within a protective dome where all the action takes place - that's the electron cloud of an atom. This cloud, far from being a solid structure, ebbs and flows like an ocean, teeming with near-light-speed particles dancing to the rhythm of quantum physics. The spectacular dance of electrons within this space is indeed a marvel. Not fixed in orbits, as previous models suggested, electrons zip around the nucleus in a kind of probability cloud, an area where they are likely but not certain to be at any given moment. Hence, the electron cloud gives insight into the potential locations of these negativons, building a dynamic portrait of their whereabouts. Understanding the electron cloud is not just about appreciating the subatomic choreography. It provides profound insights into the chemical behavior of atoms. The outer layers of these clouds are elements' social interfaces where atoms can mingle, forming bonds to construct molecules - the cornerstone of every substance in the universe. The different cloud structures explain why certain atoms form specific bonds and how their reactions ignite chemistry's spark. Leveraging the concept of energy levels, the electron cloud portrays a captivating image of matter's fundamental particles. Inner electrons whirl close to the nucleus on lower energy levels, while outer electrons occupy higher levels. The nature of these energy levels is intimately linked with the atom's unique properties, dictating its identity in the periodic table and defining its role in chemical reactions. Wrapping up our exploration of the electron cloud, we must appreciate its contribution to the atom's overall stability. The instinctive negative charge of the cloud combined with the positive charge of the nucleus results in a harmonized balance. This balanced state defines the atom's fundamental stability, ensuring it doesn't spontaneously break down. Overall, the exploration of the electron cloud enhances our grasp of the atomic world, making clear how this vast nebulous space provides the stage on which the elemental dance of creation occurs. So, next time when you wonder what an atom looks like, imagine the electron cloud - the invisible symphony of particles whirling, twirling and etching their paths in the fabric of everything we know.
The Electron Cloud and Its Implications in Visualizing the Atom
The framework of the atom, as presented by quantum mechanics, is surprisingly complex and rich, marked profoundly by the concept of the electron cloud. The electron cloud, in essence, represents the regions around the nucleus where electrons are likely to exist and is radically transforming how we visualize the atom. This new understanding invites us to delve into three crucial aspects: the electron probability distribution, atomic orbitals, and the uncertainty principle. First, the electron probability distribution unfolds a revolutionary way of visualizing the atom, illustrating how electron locations aren't definite, but probabilistic. Next, the concept of atomic orbitals brings a fresh understanding to the paths electrons take, spurring a departure from the simple, neat orbits we've learned on school benches. These orbitals offer a profound glimpse into the bizarre and fascinating world of quantum mechanics. Lastly, the uncertainty principle underlines the challenges scientists face in pinpointing the exact location and momentum of electrons simultaneously. Each of these aspects intensifies the depth of our perspective on atomic structure and particle behavior, leading us to appreciate the intricate beauty of the minuscule components of our universe. We start our journey of exploration into these facets of atomic theory - by first understanding the electron probability distribution, a remarkable new way of envisioning the atom.
Electron Probability Distribution: A New Way of Visualizing the Atom
When delving deeper into atomic structure, one encounters the fascinating concept of electron probability distribution - a revolutionary perspective that is revolutionizing the way we visualize an atom. This model is an essential component in understanding the otherwise invisible phenomena happening at atomic scales. It dismisses the idea of electrons orbiting the nucleus in a planet-like manner which we often visualize in simplified atomic models. Instead, it presents a probabilistic explanation where electrons reside in regions termed as 'clouds' or 'orbitals'. The electron probability distribution model succinctly tackles the question of an electron's whereabouts at any given time in a dynamic cloud of probabilities rather than in an exact orbit. This implies that the electron can be located anywhere within the orbital, but there are specific regions where it is more likely to be found. These regions depict a probability density - areas of high density hint at a higher probability of locating an electron, and vice versa. This new visual paradigm of the atom is largely owed to the principles of quantum mechanics, particularly the Heisenberg Uncertainty Principle. This principle dictates it's impossible to simultaneously predict both an electron's momentum and position with absolute certainty. Thus, the electron cloud symbolizes an area where an electron is most likely to exist rather than establishing a definitive pathway. Through digital representations, the complexity of this 'cloud' can be appreciated as a beautiful, three-dimensional model. These models vividly and dynamically illustrate the various electron cloud shapes for different atoms, greatly aiding our understanding. They depict a reality of the atomic world that is both abstract and breathtakingly intricate. Understanding the electron probability distribution and its implications in visualizing the atom enhances not only our basic understanding of atomic structure but also impacts advanced fields like quantum physics and chemistry. By refining our atomic visualization, we can better understand chemical reactions, quantum state transitions, and even the behavior of complex molecules, where electron positioning and behavior play a pivotal role. In conclusion, electron probability distribution offers an alternative perspective that unveils the inherent uncertainty and beauty hidden within atom's structure. This understanding revolutionizes our visualization of atoms, taking us a leap forward in comprehending the wondrous microcosm that is the foundation of our Universe. This novel approach of interpreting the unseen fosters a deeper understanding and appreciation for the intricate workings of atomic components in our macroscopic world.
Understanding Atomic Orbitals: The Paths of Electrons
Understanding Atomic Orbitals: The Paths of Electrons When diving into the fascinating world of atomic structures, we invariably encounter the concept of atomic orbitals. These orbitals are crucial in comprehending how electrons behave within an atom. As we venture beyond the macroscopic world of visible matter into the realm of the subatomic, we can visualize atomic orbitals as fuzzy clouds enveloping the nucleus, serving as the paths in which electrons reside. Atomic orbitals are defined by the energy states of the electrons the atom possesses, and are known to occupy specific quantum states that have distinct energy levels. Importantly, these energy levels are not continuous. Rather, they are quantized, indicating that electrons can inhabit only certain energy states. An atomic orbital can hold up to two electrons, both spinning in opposite directions due to a quantum mechanical property referred to as "spin." The four types of orbitals - s, p, d, and f - have unique shapes and orientations, contributing to the complexity of atomic structure. The s-orbital, the simplest, is spherical and located closest to the nucleus, while the p-orbital is dumbbell-shaped and resides further out. The d and f orbitals, increasingly complex, add vital layers to the atomic structure, thereby enabling the existence of a diverse array of elements. These atomic orbitals grant electrons a degree of freedom within the confined space of an atom. While it's virtually impossible to pinpoint the exact location of an electron within an orbital at any given instant, we can determine the probability distribution of an electron's position - a crucial factor in the electron cloud model. This model represents a paradigm shift from the earlier 'planetary model' of electrons circling the nucleus along defined pathways. It underscores the statistical nature of quantum mechanics, positioning atomic orbitals as key to understanding electron behavior. In the grand context of visualizing the atom, atomic orbitals play a vital role, contributing to the depiction of the electron cloud. They facilitate a more nuanced understanding of the atom, allowing us to explore the paths of electrons in spatial dimensions Previously inaccessible using classical physics. Hence, understanding atomic orbitals creates pathways to comprehend complex theoretical constructs and accurately captures the fascinating realm within an atom.
The Uncertainty Principle and The Challenges in Pinpointing Electrons
In the mysterious realm of quantum mechanics and atomic structures, the Uncertainty Principle plays a pivotal role in elucidating the complex and elusive nature of electrons and their positioning within an atom. This principle, famously introduced by Werner Heisenberg, suggests that it's fundamentally impossible to simultaneously confirm an electron's precise location and momentum. This revolutionary concept can be likened to trying to pinpoint a hummingbird in the midst of a swift, erratic flight, indicating the challenges in effectively locating electrons. The Uncertainty Principle forces us to visualize atoms not as neat little solar systems with electrons orbiting the nucleus like planets around a sun, but as "electron clouds," in which the precise position of an electron is replaced with a range of probable locations. This is the crux of one of the most insightful, yet perplexing, models of an atom – the Electron Cloud Model. Unlike the Bohr’s model, where electrons are depicted on definite orbits, the Electron Cloud Model embodies a probabilistic approach. The challenges in pinpointing the electrons emanate from their dual nature. Electrons display traits synonymous with both particles and waves. This wave-particle duality, akin to trying to capture the fluid motion of a flamenco dancer, further aggrandizes the intricacy of the electron's position. It’s noteworthy, this complex dance in the world of quantum physics isn't a product of technological limitations but rather stems from nature’s fundamental laws. Understanding the Uncertainty Principle and the hurdles in locating electrons is not just an esoteric exercise in quantum mechanics but it carries profound implications in understanding the atom and thus the material universe. The electron cloud shapes our understanding of the atom's structure, atomic dynamics, chemical bonding, and reactivity, marking the intersection between the invisible quantum world and the tangible reality we experience every day. It's through this lens of uncertainty and probability that we can begin to comprehend the paradoxical atomic world with its subatomic components. As challenging as it may be, this conceptual shift, prompted by the Uncertainty Principle, furnishes us with an enhanced, albeit probabilistic, understanding of the atom's internal world - the Electron Cloud. This, tied with the principles of quantum mechanics, becomes a cornerstone in our continuous journey to unravel the quintessential mysteries of atoms and matter. Thus, the Quantum Uncertainty Principle and the ensuing challenges in pinpointing electrons illuminate the vibrant frontiers of the atomic world, meticulously sowing the seeds of wonderment and curiosity.
The Evolution of The Atomic Model: From Democritus to Quantum Mechanics
The journey of understanding the atom, that fundamental cornerstone of matter, has been a fascinating evolution of scientific thought and insight. From the earliest notions of the atom proposed by ancient philosophers like Democritus, to the modern complexities of quantum mechanics, our understanding of the atomic world has shifted dramatically. This article will delve into three major epochs of this journey. We start with the ancient atom as it was conceived by Democritus, who presented the concept of indivisible particles, a bold idea that marked the beginning of atomic theory. We then segue to a period that saw a significant leap in atomic understanding with the contributions of Rutherford and Bohr, creators of the planetary model. This model revolutionized the atomic theory, shaping the interpretation and study of atomic behavior for years to come. Finally, we enter the world of the quantum atom, an unpredictable and enigmatic perspective that challenges traditional physics and opens new, complex dimensions to the understanding of matter. Before all these advances, however, stood the original idea of the atom, an indivisible entity, as articulated by Democritus. His insight laid the groundwork for our modern exploration of the atomic world, making it the perfect starting point to our journey through the evolution of the atomic model.
The Ancient Atom: Democritus's Indivisible Particles
The concept of the atom dates back to ancient times, far beyond the realms of modern quantum physics. It is encompassed in the philosophical thoughts of a pre-Socratic Greek thinker - Democritus, hailed as the 'Father of Modern Science'. This genius philosopher was phenomenally ahead of his time when he put forth the idea of “atomos” or 'indivisible', which gave birth to the concept of atom. According to his hypothesis, the universe was composed of two elements: the physical 'atom' and the void or space in which these atoms moved. These atoms, according to Democritus, were indestructible, indivisible, eternal and homogenous. His theory proposed that these tiny 'atomos' hooked together and formed different materials based on their combination, arrangement, and position. This remarkable proposition, formulated centuries ago, underpins the current concept of molecular structure and formation. Even though he lacked experimental proof, Democritus's theoretical claims fashioned a path for future scientists who further evolved the atomic theory. His ideas got unnoticed in his time because they dovetailed poorly with popular Aristotelian beliefs. However, the validation of the atom's existence many centuries later signified Democritus's vision and scientific predilection. Nevertheless, it's fascinating to see certain parallels between Democritus's theory and the modern atomic model. The unvarying property and indestructibility of atoms find echo in the conservation of energy and mass principle in current Physics; and the void which he talked of can be compared to the spacious interstitial region in the atom. Democritus's atomic theory was, without a doubt, rudimentary. But the evolution of atom's conceptual model from this early Greek philosophy to the complex quantum mechanic model reflects the innate curiosity of mankind to perceive the wide-ranging mysteries of the universe. In retrospect, while we now know the atom is not 'indivisible' as per Democritus's original concept and consists of many subatomic particles - protons, neutrons, and electrons, Democritus's ancient theory still forms the bedrock of our understanding of matter today, demonstrating the enduring influence of his truly 'indivisible' contribution to modern science and atomic theory.
The Planetary Model: Rutherford's and Bohr's Contribution to Atomic Theory
In our exploration of the atomic model's evolution, two scientists, Ernest Rutherford and Niels Bohr, hold pivotal places. They contributed immensely to our understanding of what an atom looks like by proposing and refining the Planetary Model, which offers a conceptual simplification of atom's structure. Rutherford, known as the father of nuclear physics, conducted an experiment using alpha particles and gold foil, which uncovered an atom's nucleus, at its core, has both protons and neutrons. This nucleus, he proposed, was orbited by electrons much like planets around a sun, giving birth to the Planetary Model. However, this model raised questions, such as why electrons, despite their speedy orbits, didn't lose energy and collapse into the nucleus. Here, the brilliant physicist Niels Bohr stepped in and offered a refinement of Rutherford's proposition. Integrating quantum mechanics, Bohr suggested that electrons orbit the nucleus at certain discrete distances, or energy levels, and can only jump between these levels by gaining or losing specific amounts of energy. So, instead of spiraling into the nucleus, electrons remained stable in their orbits, resolving the issue in Rutherford’s model. But this didn't mark the end of our atomic exploration. Despite its significant implications, the Bohr-Rutherford model wasn't a perfect representation of an atom's intricacy, however, it provided a palatable framework that furthered our comprehension. As atomic theory continued to evolve past these luminaries, through the evolution of quantum mechanics and wave-particle duality, this Planetary Model was an essential stepping-stone in this ever-growing field. So, while the Planetary Model is, at its heart, a simplification, the significance of Rutherford's and Bohr's contributions to the atomic theory cannot be understated. They gave us the first vivid picture of an atom's internal structure and laid a solid foundation for the complex quantum mechanical models that followed. Their work remains iconic in physics, demonstrating the narrative of scientific progress: a tale of relentless questioning, ingenious solutions, and an ever-deepening grasp of the natural world.
Quantum Mechanics: A New Perspective on The Atom
Quantum mechanics, unfathomably complex yet relentlessly fascinating, heralds a revolutionary perspective on the atom, the foundational brick of the universe. This is the realm where conventional physics seems to step into the shadow, making way for a more nuanced, probabilistic representation of atomic behavior—one that challenges much of what we inferred from earlier atomic models. The quantum mechanical model, established by revered scientists such as Schrödinger, Heisenberg, and Dirac, is an awe-inspiring progression from the traditional concept of the atomic nucleus being orbited by electrons, like planets around a sun. Instead, this model invites us to visualize the atom's structure as an electron cloud—a fuzzy fog of probabilities, rather than definite particles following predictable paths. This shift in perspective is largely due to the Heisenberg Uncertainty Principle. It asserts that an electron's position and momentum cannot be simultaneously measured with absolute accuracy. This implies that, unlike the predictability of the orbits in Bohr's model, we can only estimate the likelihood of an electron's location within a certain boundary, termed as an orbital. Another profound aspect of quantum mechanics is the concept of wave-particle duality, which suggests that particles, including electrons, can exhibit both wave-like and particle-like properties. This was largely drawn from the Schrödinger Wave Equation, which enables us to predict the statistical behavior of atomic particles. The fact that an electron can potentially behave like a wave scattered around the nucleus, rather than a mere particle, enriches our understanding of atomic structure and its workings. From these quantum fluctuations also stems the concept of superposition, which asserts that a quantum particle can exist in multiple states simultaneously, until observed. This further corroborates the probabilistic nature of atomic particles in the quantum world. Hence, quantum mechanics radically changes our perspective of the atom. It transforms the atom from a static, deterministic system to a dynamic, probabilistic one. In doing so, it nudges us to inspect the cosmos through a fresher, slightly enigmatic lens, unravelling not just what an atom looks like, but equally intriguingly, what it acts like.