What Is The Sound Barrier
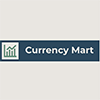
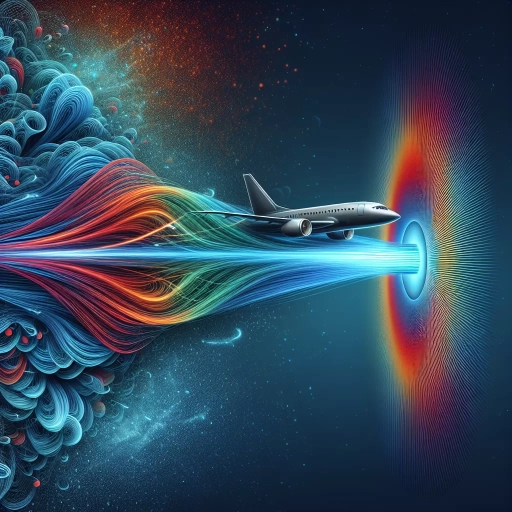
The sound barrier, a phenomenon that has captivated scientists and engineers for decades, represents the threshold beyond which an object can travel faster than the speed of sound. This barrier is not just a physical limit but also a technological and scientific challenge that has driven innovation in aviation and beyond. To fully grasp the significance of the sound barrier, it is essential to delve into three key aspects: understanding the concept itself, the scientific principles behind breaking it, and the impact and significance of achieving supersonic speeds. **Understanding the Concept of the Sound Barrier** is crucial as it lays the foundation for comprehending why this barrier exists and how it affects various fields. By exploring these facets, we can appreciate the complexity and importance of the sound barrier in modern technology. Let us begin by **Understanding the Concept of the Sound Barrier**.
Understanding the Concept of the Sound Barrier
Understanding the concept of the sound barrier is a multifaceted topic that involves delving into its definition and historical context, the physical principles that govern it, and the early attempts to break through it. The sound barrier, also known as the sonic boom, is a critical threshold in aerodynamics where an object breaks the speed of sound, producing a distinctive shockwave. To fully grasp this phenomenon, it is essential to start with its **Definition and Historical Context**, tracing back to the early 20th century when scientists and engineers first began to understand the limitations imposed by the speed of sound. This historical backdrop sets the stage for understanding the **Physical Principles Behind the Sound Barrier**, which involve complex interactions between air molecules and the object moving through them. Additionally, **Early Attempts to Break the Sound Barrier** highlight the pioneering efforts of aviators and engineers who pushed the boundaries of flight technology. By exploring these three aspects, we can gain a comprehensive understanding of the sound barrier and its significance in aviation and beyond. Let us begin by examining the **Definition and Historical Context** of this fascinating concept.
Definition and Historical Context
The concept of the sound barrier, also known as the sonic barrier, is deeply rooted in the historical context of aerodynamics and aviation. The term "sound barrier" refers to the point at which an object breaks through the speed of sound, approximately 768 miles per hour (1,236 kilometers per hour) at sea level in dry air at 59 degrees Fahrenheit (15 degrees Celsius). Historically, this barrier was a significant challenge for early aviators and engineers, who encountered severe aerodynamic issues when attempting to push aircraft beyond this speed. In the early 20th century, as aircraft design evolved and engines became more powerful, pilots began to approach the speed of sound. However, they encountered a phenomenon known as "compressibility" or "sonic boom," where air resistance increased dramatically, causing instability and making it difficult to control the aircraft. This led to a widespread belief that breaking the sound barrier was impossible. The historical context is marked by several key milestones. In 1947, Chuck Yeager, an American test pilot, successfully broke the sound barrier in the Bell X-1 rocket-powered aircraft. This achievement was a turning point in aviation history, proving that supersonic flight was feasible. Yeager's flight not only demonstrated the possibility of breaking the sound barrier but also paved the way for further research into supersonic flight. The definition of the sound barrier is closely tied to the physics of sound waves and air density. When an object moves at subsonic speeds (below the speed of sound), it creates a series of pressure waves that propagate through the air ahead of it. However, as an object approaches the speed of sound, these pressure waves become compressed and form a shockwave, which is what we hear as a sonic boom when the object breaks through the barrier. Understanding this concept is crucial for modern aviation and aerospace engineering. Supersonic aircraft must be designed to withstand the intense forces generated during transonic flight (the phase when an aircraft transitions from subsonic to supersonic speeds). Advances in materials science, aerodynamic design, and control systems have enabled the development of supersonic jets and missiles that can efficiently operate beyond the sound barrier. In summary, the sound barrier is a critical threshold in aerodynamics that marks the transition from subsonic to supersonic flight. Its historical context is defined by early challenges and breakthroughs, particularly Chuck Yeager's successful flight in 1947. The definition of this barrier is grounded in the physics of sound waves and air resistance, making it a fundamental concept in understanding high-speed flight.
Physical Principles Behind the Sound Barrier
The sound barrier, often referred to as the "sonic boom," is a phenomenon that occurs when an object breaks through the speed of sound, approximately 768 miles per hour (1,236 kilometers per hour) at sea level. The physical principles behind this barrier are rooted in aerodynamics and the behavior of air molecules. When an object moves at subsonic speeds, air molecules can move out of the way in time, creating a smooth flow around the object. However, as the object approaches the speed of sound, air molecules cannot move quickly enough to get out of the way, leading to a buildup of pressure and density in front of the object. This creates a shockwave that propagates through the air as a sharp, audible boom. At supersonic speeds, the shockwave forms a cone-shaped region behind the object known as the Mach cone. The angle of this cone depends on the Mach number, which is the ratio of the object's speed to the speed of sound. As the Mach number increases, the angle of the Mach cone decreases. When an aircraft breaks through the sound barrier, it essentially pushes through this wall of compressed air, generating intense pressure waves that produce the characteristic sonic boom. The transition from subsonic to supersonic flight involves overcoming significant aerodynamic resistance due to drag forces. As an aircraft approaches Mach 1 (the speed of sound), drag increases dramatically because of the formation of shockwaves. This increase in drag can be so severe that it requires powerful engines to push through this barrier efficiently. Once past Mach 1, drag decreases as the aircraft enters a more stable supersonic regime. Understanding these physical principles is crucial for designing aircraft capable of breaking through the sound barrier. Engineers must consider factors such as airfoil design, materials strength, and engine power to ensure that an aircraft can withstand the stresses associated with supersonic flight. Additionally, mitigating sonic booms is a significant challenge for supersonic aircraft designers aiming to reduce noise pollution and comply with regulatory standards. In summary, breaking through the sound barrier involves overcoming intense aerodynamic forces and understanding how air behaves at different speeds relative to sound. The formation of shockwaves and Mach cones are key aspects that define this phenomenon, making it both fascinating and challenging from an engineering perspective.
Early Attempts to Break the Sound Barrier
Early attempts to break the sound barrier were marked by significant challenges and innovative solutions. The concept of the sound barrier, also known as the "wall of sound," emerged in the early 20th century as aircraft began to approach the speed of sound (approximately 768 miles per hour at sea level). One of the pioneers in this field was Chuck Yeager, an American test pilot who made history on October 14, 1947, by becoming the first person to break the sound barrier in the Bell X-1 rocket-powered aircraft. However, Yeager's achievement was not without precedent; numerous other pilots and engineers had previously attempted to overcome this aerodynamic limit. In the 1930s and 1940s, several countries, including the United States, Germany, and Britain, conducted extensive research on supersonic flight. Theoretical work by scientists like Theodore von Kármán and Hugh Dryden laid the groundwork for understanding the physics behind sonic booms and shock waves. Practical attempts involved modifying existing aircraft to reduce drag and increase power. For instance, the British de Havilland DH.108 Swallow and the German Messerschmitt Me 163 Komet were among the early experimental planes designed to reach supersonic speeds. Despite these efforts, many pilots faced severe difficulties due to the intense forces generated when an object approaches Mach 1 (the speed of sound). The phenomenon known as "compressibility" caused air to behave more like a solid than a fluid at high speeds, leading to unpredictable aerodynamic behavior and often catastrophic consequences. Several pilots lost their lives in these early experiments, highlighting the risks involved in pushing the boundaries of flight technology. The breakthrough came with advancements in materials science and aerodynamic design. The development of swept-wing aircraft and the use of rocket propulsion allowed pilots to momentarily exceed the speed of sound. Chuck Yeager's successful flight in the Bell X-1, named "Glamorous Glennis" after his wife, was a culmination of these technological advancements and rigorous testing protocols. His achievement not only demonstrated that breaking the sound barrier was possible but also paved the way for future generations of supersonic aircraft and space exploration. In summary, early attempts to break the sound barrier were characterized by scientific curiosity, engineering innovation, and a willingness to take risks. From theoretical research to practical experimentation, these efforts laid the foundation for modern aviation and continue to inspire advancements in aerodynamics and flight technology. Understanding these historical milestones is crucial for appreciating the complexities and achievements associated with supersonic flight.
The Science Behind Breaking the Sound Barrier
Breaking the sound barrier is a complex phenomenon that has fascinated scientists and engineers for decades. At its core, it involves overcoming significant aerodynamic challenges, understanding the role of air density and temperature, and leveraging technological innovations in aircraft design. When an object approaches the speed of sound, it encounters a wall of compressed air that generates immense drag and heat, making it difficult to penetrate. To address these aerodynamic challenges, engineers have developed sophisticated solutions such as streamlined shapes and advanced materials. Additionally, air density and temperature play crucial roles in determining the speed of sound, with lower temperatures and higher altitudes affecting the barrier's location. Technological innovations, including the development of supersonic aircraft like the Concorde, have also been pivotal in achieving supersonic flight. By delving into these aspects, we can gain a deeper understanding of the science behind breaking the sound barrier. Let's start by examining the aerodynamic challenges and solutions that have been instrumental in this pursuit.
Aerodynamic Challenges and Solutions
Breaking the sound barrier poses significant aerodynamic challenges, primarily due to the dramatic changes in airflow behavior as an object approaches and exceeds the speed of sound. One of the most critical issues is the formation of shock waves, which occur when air cannot move out of the way quickly enough to accommodate the object's speed. These shock waves create intense pressure and temperature gradients that can lead to structural stress and instability. Additionally, as an object nears Mach 1 (the speed of sound), it encounters a phenomenon known as the "sound barrier," where drag increases exponentially due to the compression of air ahead of the object. This results in a substantial increase in energy required to push through this dense air layer. To overcome these challenges, engineers employ several aerodynamic solutions. One key strategy is the use of streamlined shapes that minimize drag by reducing the formation of shock waves. For instance, aircraft designed to break the sound barrier often have tapered noses and slender profiles to help air flow smoothly around them. Another crucial technique is the application of swept wings, which delay the onset of shock waves by allowing air to flow more gradually over the wing surface. Advanced materials also play a vital role in addressing these aerodynamic challenges. High-strength, lightweight materials such as titanium and advanced composites are used to construct aircraft that can withstand the stresses generated by supersonic flight. Furthermore, sophisticated computational fluid dynamics (CFD) and wind tunnel testing are essential tools for optimizing aerodynamic design. These methods allow engineers to simulate and test various configurations under controlled conditions, enabling them to refine their designs and predict performance accurately. In addition, active control systems are integrated into supersonic aircraft to manage airflow dynamically. These systems can adjust wing angles, deploy flaps, or use thrust vectoring to maintain stability and control during the transition through Mach 1. The development of afterburners in jet engines also helps by providing an additional boost of thrust necessary to overcome the increased drag encountered at transonic speeds. Finally, understanding the physics behind sonic booms is crucial for mitigating their impact. Sonic booms are caused by the shock waves produced when an object breaks the sound barrier, resulting in a loud noise on the ground. Researchers are working on shaping aircraft to reduce these booms by spreading out the shock waves over a longer distance, thereby reducing their intensity. In summary, breaking the sound barrier requires meticulous attention to aerodynamic design, advanced materials, sophisticated testing methods, and innovative control systems. By addressing these challenges through a combination of theoretical understanding and practical engineering solutions, scientists and engineers have successfully enabled aircraft to operate efficiently beyond Mach 1, pushing the boundaries of flight technology.
Role of Air Density and Temperature
The role of air density and temperature is crucial in understanding the science behind breaking the sound barrier. Air density, which varies with temperature and altitude, directly influences the speed of sound. At sea level, where air is denser due to higher pressure and lower altitude, the speed of sound is approximately 768 miles per hour (mph) or 1,236 kilometers per hour (km/h) at a temperature of 59 degrees Fahrenheit (15 degrees Celsius). However, as temperature increases, air molecules move faster and spread out, reducing air density and thereby increasing the speed of sound. Conversely, lower temperatures result in slower-moving molecules, higher air density, and a slower speed of sound. When an object approaches the speed of sound, it encounters significant resistance due to the buildup of pressure waves in front of it. This resistance, known as drag, becomes exponentially greater as the object nears Mach 1 (the speed of sound). To break through this barrier, an aircraft must generate enough thrust to overcome this intense drag. Here, temperature plays a critical role: at higher altitudes where temperatures are lower, air density decreases, making it easier for an aircraft to achieve supersonic speeds because there is less drag to overcome. Additionally, temperature gradients in the atmosphere can affect flight dynamics. For instance, flying through a layer of cold air can suddenly increase air density, making it harder for an aircraft to maintain supersonic speeds. Conversely, encountering a warm layer can reduce air density, potentially aiding in breaking through the sound barrier. In practical terms, military jets and experimental aircraft often take advantage of these atmospheric conditions to achieve supersonic flight. Pilots may ascend to higher altitudes where lower air densities make it easier to break the sound barrier before descending back into denser air layers. The precise calculation of air density and temperature profiles is essential for successful supersonic flight planning and execution. In summary, understanding how air density and temperature influence the speed of sound is vital for breaking through the sound barrier. By leveraging these atmospheric conditions strategically, aircraft can overcome the significant drag associated with approaching Mach 1 and achieve supersonic speeds efficiently. This intricate interplay between air properties and flight dynamics underscores the complex science behind supersonic aviation.
Technological Innovations in Aircraft Design
Technological innovations in aircraft design have been pivotal in overcoming the challenges associated with breaking the sound barrier. One of the most significant advancements has been in materials science, where the development of lightweight yet incredibly strong materials such as titanium and advanced composites has enabled the construction of aircraft capable of withstanding the intense stresses generated during supersonic flight. These materials allow for the creation of sleek, aerodynamic shapes that minimize drag while maintaining structural integrity. Another crucial innovation is the use of computer-aided design (CAD) and computational fluid dynamics (CFD). These tools enable engineers to simulate and optimize aircraft designs, predicting how different configurations will perform under various conditions, including supersonic flight. This has significantly reduced the need for physical prototypes and has accelerated the design process, allowing for more precise and efficient aircraft development. Advances in engine technology have also played a critical role. The development of high-bypass turbofan engines and afterburning turbojets has provided the necessary thrust-to-weight ratios to achieve and sustain supersonic speeds. These engines are designed to operate efficiently at high altitudes and speeds, ensuring that aircraft can break through the sound barrier without compromising performance or safety. Furthermore, innovations in aerodynamics have led to the design of unique features such as area ruling and variable geometry wings. Area ruling involves shaping the aircraft to minimize cross-sectional area where it is most critical, reducing drag during supersonic flight. Variable geometry wings allow for adjustments to be made in real-time to optimize performance across different speed regimes, enhancing stability and maneuverability. Additionally, advancements in avionics and control systems have been essential. Fly-by-wire systems and sophisticated flight control computers enable precise control over the aircraft, compensating for the instability that can occur during transonic flight. These systems also provide real-time data analysis and feedback, helping pilots navigate the complex conditions associated with breaking the sound barrier. Lastly, the integration of advanced sensors and monitoring technologies has improved safety and performance. These sensors continuously monitor various parameters such as temperature, pressure, and structural stress, providing critical feedback that helps in maintaining optimal operating conditions even at supersonic speeds. In summary, technological innovations in materials science, CAD/CFD, engine technology, aerodynamics, avionics, and sensor technologies have collectively contributed to the successful design of aircraft capable of breaking the sound barrier. These advancements have not only enabled faster flight but also ensured that such speeds can be achieved safely and efficiently.
Impact and Significance of Breaking the Sound Barrier
The breaking of the sound barrier, achieved by Chuck Yeager in 1947, has had profound impacts across various domains, transforming the landscape of aviation and beyond. This milestone not only paved the way for significant advancements in military and commercial aviation but also had far-reaching psychological and societal implications. The ability to fly at supersonic speeds has revolutionized military tactics, enabling faster response times and enhanced strategic capabilities. In the commercial sector, it has opened up possibilities for reducing travel times, potentially transforming global connectivity. Additionally, the psychological and societal impacts of this achievement have been substantial, inspiring generations and redefining human perceptions of speed and technological capability. As we look to the future, ongoing research and innovations in supersonic flight promise even more exciting developments. This article will delve into these aspects, starting with the military and commercial aviation advancements that have been the direct result of breaking the sound barrier.
Military and Commercial Aviation Advancements
The advancements in military and commercial aviation have been pivotal in the history of flight, particularly in the context of breaking the sound barrier. The achievement of supersonic flight marked a significant milestone, transforming both military and commercial aviation sectors. In the military realm, breaking the sound barrier enabled the development of faster and more agile aircraft, such as the Lockheed F-104 Starfighter and the North American F-100 Super Sabre, which significantly enhanced air superiority and tactical capabilities. These supersonic jets allowed for quicker response times, improved reconnaissance, and enhanced combat effectiveness, giving nations a strategic edge in defense. In commercial aviation, while supersonic flight did not become a mainstream reality due to economic and environmental constraints, the technological innovations derived from breaking the sound barrier have had lasting impacts. The Concorde, a joint project between British Aerospace (now BAE Systems) and Aérospatiale (now Airbus), was the only commercial supersonic jet to enter service. Although it was retired in 2003 due to rising maintenance costs and declining demand, its development pushed the boundaries of aerodynamics, materials science, and engine technology. These advancements have trickled down to subsonic commercial aircraft, improving fuel efficiency, reducing noise levels, and enhancing overall performance. Moreover, the research and development efforts associated with supersonic flight have led to numerous spin-off benefits. For instance, the use of advanced materials like titanium and composites has become standard in modern aircraft construction, contributing to lighter yet stronger structures. Additionally, the sophisticated avionics and control systems developed for supersonic aircraft have been adapted for use in subsonic planes, enhancing safety and operational efficiency. The impact of breaking the sound barrier extends beyond the immediate technological advancements. It has inspired generations of engineers, scientists, and pilots, fostering a culture of innovation within the aviation industry. The psychological barrier of achieving supersonic flight has also driven human curiosity and ambition, pushing the boundaries of what is considered possible in aerospace engineering. In conclusion, the advancements in military and commercial aviation following the breaking of the sound barrier have been profound. These achievements have not only transformed the capabilities of military aircraft but also influenced the design and operation of commercial planes. The legacy of supersonic flight continues to shape the future of aviation, driving innovation and pushing the limits of human ingenuity.
Psychological and Societal Implications
The psychological and societal implications of breaking the sound barrier are profound and multifaceted. Historically, the achievement marked a significant milestone in human ingenuity and technological advancement, boosting national morale and pride. When Chuck Yeager first broke the sound barrier in 1947, it symbolized the triumph of human determination over seemingly insurmountable physical limitations. This event had a profound psychological impact on the public, inspiring a sense of awe and wonder at the capabilities of science and engineering. It also underscored the importance of innovation and risk-taking, encouraging future generations to push beyond perceived boundaries. Societally, breaking the sound barrier opened up new avenues for military and commercial aviation. The development of supersonic aircraft enabled faster travel times, which had significant economic implications by reducing travel durations and increasing the efficiency of global connectivity. This, in turn, facilitated international trade, tourism, and diplomacy, contributing to global economic growth and cultural exchange. Furthermore, the technological advancements that arose from this achievement trickled down into various other fields, such as medicine, materials science, and computing, driving broader societal progress. However, there were also negative societal impacts to consider. The noise pollution generated by supersonic flights became a significant concern, leading to environmental regulations and public health debates. Additionally, the high costs associated with developing and maintaining supersonic aircraft limited their accessibility to a select few, exacerbating existing social inequalities. Despite these challenges, the psychological and societal implications of breaking the sound barrier remain a testament to human resilience and the transformative power of technological innovation. It continues to inspire new generations of scientists, engineers, and explorers, driving us towards even greater achievements in the pursuit of pushing beyond the boundaries of what is thought possible.
Future Perspectives in Supersonic Flight
As we look to the future of supersonic flight, several key perspectives emerge that highlight its potential impact and significance. One of the most promising areas is the development of sustainable supersonic aircraft. Companies like Boom Supersonic and Aerion are working on designs that not only break the sound barrier but also aim to reduce environmental impact through more efficient engines and materials. These innovations could lead to a new era of commercial supersonic travel, significantly reducing travel times between continents and revolutionizing global connectivity. Another critical aspect is the advancement in materials science and aerodynamics. New materials such as advanced composites and smart skins are being developed to withstand the stresses associated with supersonic flight while minimizing drag and maximizing efficiency. Additionally, computational fluid dynamics and wind tunnel testing are becoming more sophisticated, allowing for more precise design and optimization of supersonic aircraft. Regulatory frameworks are also evolving to accommodate the resurgence of supersonic flight. Organizations like the Federal Aviation Administration (FAA) and the International Civil Aviation Organization (ICAO) are revising guidelines to address noise pollution and other concerns associated with supersonic travel. These changes will be crucial in paving the way for commercial supersonic flights over land, which have been restricted since the Concorde's retirement. Furthermore, technological advancements in noise reduction are pivotal. Supersonic aircraft generate significant sonic booms that have historically limited their use over populated areas. Researchers are exploring novel designs and technologies to mitigate these booms, such as variable geometry wings and specialized nose shapes. Successful implementation of these technologies could make supersonic flight more acceptable for overland routes. The economic implications of supersonic travel are also noteworthy. Faster travel times could significantly boost productivity and efficiency in business and tourism sectors, potentially leading to substantial economic gains. Moreover, the development of supersonic aircraft could stimulate innovation across various industries, from aerospace engineering to materials science. Finally, the psychological and social impacts of supersonic travel should not be underestimated. The ability to travel at speeds exceeding Mach 1 could redefine our perception of distance and time, fostering greater global connectivity and cultural exchange. It could also inspire new generations of scientists, engineers, and explorers by pushing the boundaries of what is thought possible. In summary, the future of supersonic flight is marked by significant technological, regulatory, economic, and social advancements. As these elements converge, we are likely to see a resurgence in commercial supersonic travel that not only breaks the sound barrier but also transforms our world in profound ways.