What Is A Dpsp
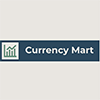
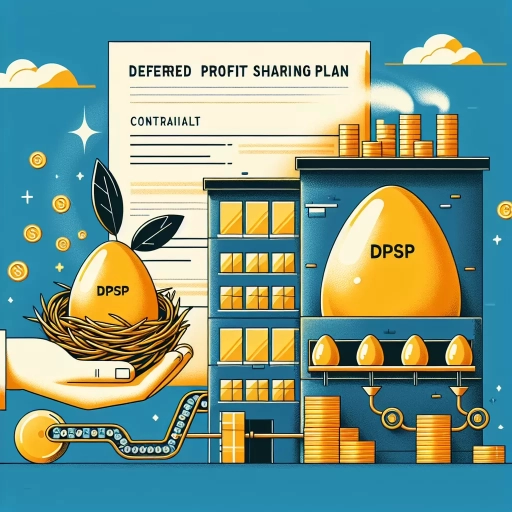
In the realm of modern technology, the term "DPS" (Data Processing System) has become increasingly significant, especially in industries that rely heavily on efficient data management and analysis. A DPS is a sophisticated system designed to handle large volumes of data, ensuring that information is processed accurately, securely, and swiftly. This article delves into the multifaceted world of DPS, exploring its fundamental principles, diverse applications, and technical intricacies. We will begin by **Understanding the Basics of DPS**, where we will dissect the core components and functionalities that define these systems. Following this foundational overview, we will examine the **Applications and Uses of DPS**, highlighting how these systems are utilized across various sectors to drive innovation and efficiency. Finally, we will delve into the **Technical Aspects and Implementation** of DPS, discussing the advanced technologies and methodologies involved in their setup and operation. By the end of this journey, readers will have a comprehensive understanding of what a DPS is and how it operates, starting with the essential basics that underpin its operation.
Understanding the Basics of DPS
In the realm of technology and gaming, the term "DPS" (Damage Per Second) has become a cornerstone metric for measuring performance and efficiency. Understanding the basics of DPS is crucial for both developers and users, as it influences game design, player strategy, and overall user experience. This article delves into the fundamental aspects of DPS, providing a comprehensive overview that begins with a **Definition and Acronym Breakdown**, where we dissect the meaning and significance of DPS in various contexts. We then explore the **Historical Context and Evolution** of DPS, tracing its origins and development over time to understand how it has become an integral part of modern gaming and technology. Finally, we examine the **Key Components and Terminology** associated with DPS, highlighting the essential elements that contribute to its calculation and application. By grasping these foundational concepts, readers will gain a deeper understanding of what DPS entails and how it impacts their interactions with digital environments. This article aims to equip you with the knowledge necessary for **Understanding the Basics of DPS**.
Definition and Acronym Breakdown
Understanding the basics of a Distributed Power System (DPS) begins with a clear grasp of its definition and the breakdown of its acronym. A Distributed Power System is an electrical power distribution system where power generation is decentralized, meaning that instead of relying on a single, large power plant, multiple smaller power sources are spread out across different locations. This approach contrasts with traditional centralized power systems, which depend on a few large power plants to supply electricity to a wide area. The acronym "DPS" itself is straightforward: **D**istributed, indicating the decentralized nature of the system; **P**ower, referring to the electrical energy being generated and distributed; and **S**ystem, signifying the network or infrastructure through which this power is managed and delivered. This decentralized model offers several advantages, including increased reliability, reduced transmission losses, and enhanced flexibility in meeting varying energy demands. In a DPS, various types of power sources can be integrated, such as solar panels, wind turbines, fuel cells, and even small-scale conventional generators. These sources can be located closer to the points of consumption, reducing the need for long-distance transmission lines and minimizing energy losses during transmission. Additionally, DPS allows for greater resilience against power outages because if one source fails or is offline, other sources can continue to supply power. The decentralized nature of DPS also fosters community-based energy solutions and can support microgrids, which are local energy systems that can operate in isolation from the main grid during emergencies. This makes DPS particularly beneficial for remote or rural areas where traditional grid connectivity might be limited or unreliable. Furthermore, DPS aligns well with modern trends towards renewable energy and sustainability. By incorporating a mix of renewable energy sources, DPS can significantly reduce greenhouse gas emissions and contribute to a cleaner, more sustainable energy future. Overall, understanding the definition and acronym breakdown of DPS is essential for appreciating its role in transforming how we generate, distribute, and consume electrical power.
Historical Context and Evolution
Understanding the basics of a Data Processing System (DPS) requires a deep dive into its historical context and evolution. The concept of data processing has its roots in the early 20th century, when manual methods were the norm. Clerks and accountants spent countless hours performing calculations and recording data by hand, a process that was both time-consuming and prone to errors. The advent of mechanical calculators in the late 19th and early 20th centuries marked the first significant step towards automating these tasks. However, it wasn't until the invention of the first electronic computers during World War II that the true potential of automated data processing began to unfold. The 1950s saw the introduction of the first commercial computers, such as UNIVAC I, which revolutionized business operations by enabling faster and more accurate data processing. This era also witnessed the development of programming languages like COBOL and FORTRAN, which facilitated the creation of software tailored to specific business needs. As technology advanced, so did the complexity and efficiency of DPS systems. The 1960s and 1970s brought about the rise of mainframe computers, which centralized data processing within large organizations. This period also saw significant improvements in storage technology with the introduction of magnetic tapes and later, disk storage. The advent of personal computers in the 1980s democratized access to data processing capabilities, allowing smaller businesses and individuals to leverage DPS for their needs. This decade also saw the emergence of relational databases, pioneered by companies like Oracle, which further enhanced data management and retrieval capabilities. The 1990s and 2000s were marked by the proliferation of the internet and cloud computing, transforming DPS into a global, interconnected network. Cloud-based services like Amazon Web Services (AWS) and Microsoft Azure enabled scalable, on-demand access to computing resources, significantly reducing costs and increasing flexibility. In recent years, advancements in artificial intelligence (AI), machine learning (ML), and big data analytics have further evolved DPS systems. These technologies enable real-time processing, predictive analytics, and automated decision-making processes. The integration of IoT devices has expanded the scope of DPS to include real-time data from various sources, enhancing operational efficiency across industries. Today’s DPS systems are not just about processing data but also about deriving actionable insights that drive business strategy and innovation. Understanding this historical context is crucial for appreciating the current state of DPS technology and its future potential in transforming how we manage and utilize data.
Key Components and Terminology
Understanding the basics of a Distributed Power System (DPS) hinges on grasping its key components and terminology. At the heart of any DPS are **power sources**, which can include traditional fossil fuel-based generators, renewable energy sources like solar panels and wind turbines, and even energy storage systems such as batteries. These power sources feed into **power conversion systems**, which transform raw energy into usable electrical power. This process often involves **inverters** for converting DC power to AC, and **rectifiers** for the reverse conversion. **Distribution networks** play a crucial role in DPS, ensuring that the generated power is efficiently transmitted to various loads. These networks can be wired or wireless and may include **transformers** to adjust voltage levels for safe and efficient transmission. **Load management systems** are essential for optimizing energy distribution, ensuring that power is allocated based on demand and availability. This often involves sophisticated **control systems** that monitor and adjust the flow of power in real-time. Key terminology in DPS includes **peak demand**, which refers to the maximum amount of power required by the system at any given time, and **base load**, the minimum amount of power needed to meet continuous demand. **Efficiency** is another critical term, measuring how effectively the system converts input energy into usable output. **Reliability** and **resilience** are also vital, indicating the system's ability to maintain operation under normal conditions and recover from disruptions. **Scalability** is a significant advantage of DPS, allowing systems to be easily expanded or contracted based on changing energy needs. This flexibility is often achieved through **modular design**, where components can be added or removed as necessary. **Grid stability** is maintained through advanced **power quality management**, which ensures that the electrical supply remains within acceptable voltage and frequency limits. In addition, **energy storage** technologies like batteries and supercapacitors are integral to modern DPS, enabling the system to buffer against fluctuations in supply and demand. **Smart grid technologies** further enhance DPS by integrating advanced sensors, IoT devices, and data analytics to optimize performance and predict potential issues before they occur. Understanding these components and terms provides a solid foundation for appreciating the complexities and benefits of Distributed Power Systems. By leveraging these elements, DPS can offer reliable, efficient, and adaptable solutions for a wide range of applications, from residential to industrial settings. This foundational knowledge is essential for anyone looking to delve deeper into the world of DPS and its role in shaping the future of energy distribution.
Applications and Uses of DPS
In the modern era, Distributed Power Systems (DPS) have emerged as a pivotal technology, transforming various sectors with their versatility and efficiency. DPS, which involves the distribution of power generation across multiple sources, offers a robust solution to energy needs by enhancing reliability, reducing costs, and improving environmental sustainability. This article delves into the diverse applications and uses of DPS, highlighting its significant impact in three key areas: Industrial and Manufacturing Settings, where DPS ensures continuous operation and optimizes energy consumption; Healthcare and Medical Applications, where reliable power is crucial for life-saving equipment and patient care; and Environmental Monitoring and Control, where DPS supports the operation of critical monitoring systems and sustainable energy solutions. By understanding these applications, readers will gain a comprehensive insight into the multifaceted benefits of DPS. To fully appreciate these advancements, it is essential to first grasp the fundamental principles underlying DPS, which will be explored in detail in the section titled "Understanding the Basics of DPS."
Industrial and Manufacturing Settings
In industrial and manufacturing settings, the applications and uses of Distributed Power Systems (DPS) are multifaceted and critical for ensuring operational efficiency, reliability, and sustainability. DPS solutions, which include a combination of power generation, distribution, and control systems, play a pivotal role in modern industrial environments. For instance, in manufacturing facilities, DPS can provide redundant power sources to prevent downtime and ensure continuous production. This is particularly crucial in sectors such as automotive, aerospace, and pharmaceuticals where any interruption can lead to significant financial losses and compromise product quality. Moreover, DPS can integrate various energy sources including renewable options like solar and wind power, thereby reducing dependence on traditional fossil fuels and lowering carbon footprints. This not only aligns with corporate sustainability goals but also helps in complying with stringent environmental regulations. Additionally, advanced DPS systems often come equipped with smart grid technologies that enable real-time monitoring and control of energy usage. This allows for optimized energy consumption patterns, predictive maintenance scheduling, and swift response to any anomalies or failures within the power network. The flexibility of DPS is another key advantage in industrial settings. These systems can be scaled up or down according to the specific needs of the facility, making them highly adaptable to changing production demands. For example, during peak production periods or when new machinery is introduced, DPS can easily accommodate increased power requirements without the need for extensive infrastructure upgrades. Furthermore, DPS enhances safety by providing backup power during outages or emergencies. In hazardous environments such as chemical plants or oil refineries, reliable backup power ensures that critical safety systems remain operational even when primary power sources fail. This safeguard against potential disasters underscores the importance of DPS in maintaining operational safety standards. In summary, the integration of DPS in industrial and manufacturing settings is transformative. It offers a robust framework for reliable power supply, energy efficiency, sustainability, flexibility, and enhanced safety protocols. As industries continue to evolve with technological advancements and increasing regulatory pressures, the role of DPS will only become more pivotal in ensuring that operations remain resilient and efficient.
Healthcare and Medical Applications
In the realm of healthcare and medical applications, Distributed Power Systems (DPS) play a pivotal role in ensuring the reliability, efficiency, and safety of critical medical infrastructure. One of the most significant applications of DPS in healthcare is the provision of uninterrupted power supply to life-saving equipment. Hospitals and medical facilities rely heavily on DPS to power devices such as ventilators, dialysis machines, and cardiac monitors, which are essential for patient care and treatment. These systems ensure that even during power outages or grid failures, these critical devices continue to function without interruption, thereby safeguarding patient lives. Moreover, DPS enhances the operational efficiency of healthcare facilities by providing a stable and consistent power supply. This is particularly important in surgical suites where precise and continuous power is crucial for the success of surgical procedures. The use of DPS also extends to diagnostic imaging technologies like MRI and CT scanners, which require high-quality power to produce accurate images. By minimizing power fluctuations and ensuring a clean power supply, DPS helps in maintaining the integrity of diagnostic results. In addition to supporting medical equipment, DPS contributes to the overall infrastructure of healthcare facilities. It powers data centers that store patient records, medical research data, and other critical information. This ensures that healthcare providers have access to vital information at all times, facilitating better decision-making and patient care. Furthermore, DPS supports communication systems within hospitals, enabling seamless communication between healthcare professionals, which is essential for coordinated care. The integration of DPS with renewable energy sources such as solar and wind power is another emerging trend in healthcare. This not only reduces the carbon footprint of healthcare facilities but also provides a sustainable solution for power generation. For remote or disaster-stricken areas where traditional grid power may be unavailable, DPS can be combined with renewable energy to create self-sustaining medical facilities. In summary, the applications of DPS in healthcare are multifaceted and indispensable. By providing reliable, efficient, and sustainable power solutions, DPS ensures the continuity of critical medical services, enhances operational efficiency, supports advanced diagnostic technologies, and contributes to the overall infrastructure of healthcare facilities. As the healthcare sector continues to evolve with new technologies and challenges, the role of DPS will remain vital in maintaining high standards of patient care and medical excellence.
Environmental Monitoring and Control
Environmental monitoring and control are critical components in the broader spectrum of applications and uses of Distributed Power Systems (DPS). DPS, which involves the decentralized generation and distribution of power, plays a pivotal role in ensuring sustainable and efficient energy management. In the context of environmental monitoring, DPS systems can be integrated with various sensors and monitoring devices to track key environmental parameters such as air quality, water quality, soil health, and noise pollution. These real-time data streams enable precise monitoring of environmental conditions, allowing for swift action to mitigate any adverse impacts. For instance, DPS can power remote weather stations and air quality monitoring stations in rural or hard-to-reach areas, providing vital data on atmospheric conditions. This data is crucial for predicting weather patterns, tracking climate changes, and issuing timely alerts for natural disasters like hurricanes or wildfires. Additionally, DPS can support the operation of water quality sensors in rivers, lakes, and oceans, helping to detect pollutants and ensure compliance with regulatory standards. In terms of control, DPS systems can be used to manage and regulate environmental impacts more effectively. For example, they can power smart grids that optimize energy distribution based on real-time demand, reducing energy waste and lowering greenhouse gas emissions. Moreover, DPS can integrate with renewable energy sources like solar and wind power, promoting a cleaner energy mix and reducing reliance on fossil fuels. The use of DPS in environmental control also extends to industrial settings. By powering advanced pollution control technologies such as scrubbers and electrostatic precipitators, DPS helps industries comply with stringent environmental regulations. Furthermore, DPS can support the operation of waste management systems, including recycling facilities and waste-to-energy plants, thereby reducing landfill waste and promoting a circular economy. In summary, the integration of DPS with environmental monitoring and control systems enhances our ability to protect the environment while ensuring efficient and sustainable energy use. By leveraging the decentralized nature of DPS, we can achieve more accurate monitoring, better control over environmental impacts, and a significant reduction in our ecological footprint. This synergy between DPS and environmental management underscores the multifaceted benefits of adopting distributed power systems in various sectors.
Technical Aspects and Implementation
In the realm of modern technology, understanding the technical aspects and implementation of complex systems is crucial for optimal performance and efficiency. This article delves into the essential components that underpin successful system deployment, focusing on three critical areas: Hardware and Software Requirements, Installation and Calibration Procedures, and Troubleshooting and Maintenance Tips. By examining the hardware and software prerequisites, readers will gain insight into the foundational elements necessary for system functionality. The installation and calibration procedures section will provide step-by-step guidance on setting up these systems effectively. Finally, troubleshooting and maintenance tips will equip users with the knowledge to resolve common issues and ensure long-term reliability. These detailed explanations are designed to transition seamlessly into a deeper understanding of the basics of Distributed Power Systems (DPS), enabling readers to grasp the fundamental principles that drive these advanced technologies. By mastering these technical aspects, individuals can enhance their proficiency in managing and optimizing complex systems, ultimately leading to improved operational outcomes.
Hardware and Software Requirements
When delving into the technical aspects and implementation of a Data Processing System (DPS), it is crucial to meticulously outline both the hardware and software requirements. These components are the backbone of any efficient data processing system, ensuring that data is handled, processed, and stored effectively. ### Hardware Requirements The hardware infrastructure of a DPS includes several key components. **Servers** are essential for housing the system's core processing units, memory, and storage. High-performance servers with multiple CPU cores, ample RAM, and robust storage solutions such as SSDs or SANs are necessary to handle large volumes of data efficiently. **Network Infrastructure** is another critical aspect; high-speed Ethernet connections or even fiber-optic links ensure rapid data transfer between different nodes within the system. **Storage Systems**, including hard drives or solid-state drives, must be capable of storing vast amounts of data securely and reliably. Additionally, **Backup Systems** such as tape drives or cloud storage solutions are vital for ensuring data integrity and recovery in case of failures. **Power Supply Units (PSUs)** and **Uninterruptible Power Supplies (UPS)** are also essential to maintain continuous operation even during power outages. ### Software Requirements On the software front, several layers of technology come into play. **Operating Systems** such as Linux or Windows Server provide the foundational platform for running various applications. **Database Management Systems (DBMS)** like MySQL, Oracle, or MongoDB are critical for organizing and retrieving data efficiently. **Programming Languages** such as Python, Java, or C++ are used to develop custom applications tailored to specific data processing needs. **Data Processing Frameworks** like Apache Hadoop, Apache Spark, or TensorFlow facilitate distributed processing and machine learning tasks on large datasets. **Security Software** including firewalls, intrusion detection systems, and encryption tools protect sensitive data from unauthorized access and breaches. Finally, **Monitoring Tools** such as Nagios or Prometheus help in tracking system performance and identifying potential issues before they escalate. ### Integration and Compatibility Ensuring seamless integration between hardware and software components is paramount. Compatibility checks must be conducted to verify that all hardware elements can support the required software applications without performance degradation. Regular updates and patches for both hardware firmware and software applications are necessary to maintain optimal performance and security. In summary, the hardware and software requirements for a DPS are interdependent and must be carefully selected to ensure robustness, scalability, and security. By investing in high-quality hardware infrastructure and leveraging appropriate software technologies, organizations can build a reliable DPS that meets their data processing needs efficiently and securely. This balanced approach not only enhances operational efficiency but also safeguards against potential failures and security threats.
Installation and Calibration Procedures
When it comes to the technical aspects and implementation of a Distributed Power Supply System (DPSS), the installation and calibration procedures are crucial for ensuring optimal performance, reliability, and safety. The process begins with a thorough site survey to identify the best locations for the power supply units, taking into account factors such as accessibility, environmental conditions, and proximity to the load. Once the sites are selected, the installation phase involves mounting the units securely, connecting them to the power grid, and integrating them with other system components like control panels and monitoring systems. Proper grounding and bonding are essential to prevent electrical shocks and ensure safe operation. This includes connecting all metal parts of the equipment to a common grounding point and ensuring that all connections are secure and free from corrosion. The next step is to perform initial power-up tests to verify that each unit is functioning correctly. This involves checking for proper voltage output, current draw, and any signs of malfunction or overheating. Calibration follows closely after installation. This involves adjusting the settings of each power supply unit to match the specific requirements of the system. For instance, voltage and current limits may need to be set according to the specifications of the load devices. Additionally, calibration may involve synchronizing multiple power supply units to operate in parallel or series configurations, ensuring seamless operation during peak demand periods. Advanced DPSS systems often include sophisticated control and monitoring features that require software configuration. This may involve setting up communication protocols, configuring alarm thresholds, and integrating with existing SCADA (Supervisory Control and Data Acquisition) systems. Regular maintenance checks are also integral to the calibration process, ensuring that all components are in good working condition and that any potential issues are identified and addressed promptly. Finally, thorough documentation of the installation and calibration procedures is vital for future reference and troubleshooting. This includes detailed records of all settings, configurations, and test results. By following these meticulous steps, organizations can ensure that their DPSS operates efficiently, reliably, and safely, meeting the high standards required in critical applications such as data centers, healthcare facilities, and industrial plants. Effective installation and calibration not only enhance system performance but also contribute significantly to reducing downtime and extending the lifespan of the equipment.
Troubleshooting and Maintenance Tips
When it comes to ensuring the optimal performance of a Distributed Power Supply System (DPSS), troubleshooting and maintenance are crucial components. Effective troubleshooting involves a systematic approach to identify and resolve issues promptly, minimizing downtime and maintaining system reliability. Start by monitoring key parameters such as voltage, current, and temperature through integrated sensors or external monitoring tools. Regularly inspect physical connections for signs of wear or corrosion, as these can lead to intermittent failures. Utilize diagnostic software or built-in diagnostic modes to analyze system logs and error codes, which can pinpoint specific faults. For preventive maintenance, schedule periodic checks to ensure all components are functioning within specified tolerances. Clean dust from heat sinks and fans to maintain efficient cooling, and replace filters in air-cooled systems regularly. Perform firmware updates to leverage the latest improvements and bug fixes. It is also essential to maintain a spare parts inventory to expedite repairs when necessary. In addition, implementing redundancy in critical components can significantly enhance system availability. This includes having backup power modules, redundant communication links, and duplicate control units. Regularly test these redundant systems to ensure they are operational and can seamlessly take over in case of a failure. Training personnel on troubleshooting techniques and maintenance procedures is another vital aspect. This includes hands-on training sessions and access to detailed technical documentation. By empowering maintenance teams with the right skills and resources, you can reduce the time spent on troubleshooting and ensure that any issues are resolved efficiently. Finally, documenting all maintenance activities and troubleshooting steps taken can help in identifying recurring issues and improving overall system design. This historical data can be invaluable for future upgrades or when troubleshooting similar problems in other systems. By combining proactive maintenance with effective troubleshooting strategies, you can ensure your DPSS operates at peak performance, providing reliable power distribution while minimizing operational costs and downtime.