What Is Bioaccumulation And Biomagnification
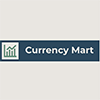
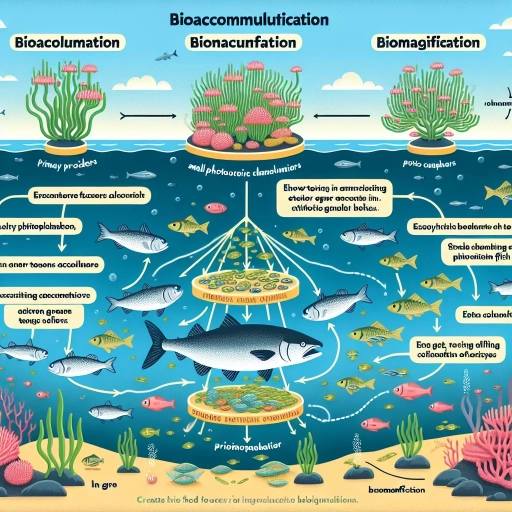
Bioaccumulation and biomagnification are critical concepts in environmental science that highlight the persistent and often detrimental impact of pollutants on ecosystems. Bioaccumulation refers to the process by which substances, such as heavy metals and pesticides, accumulate in an organism's body over time, often at concentrations higher than those found in the environment. Biomagnification, on the other hand, describes how these substances become more concentrated as they move up the food chain, posing significant risks to higher-level predators and ultimately to human health. Understanding these phenomena is crucial for assessing environmental health and developing effective mitigation strategies. This article delves into the intricacies of bioaccumulation, explores the mechanisms of biomagnification, and discusses the consequences and potential mitigation strategies. By grasping these concepts, we can better navigate the complex interactions between pollutants, organisms, and ecosystems. Let us begin by **Understanding Bioaccumulation**, the foundational process that sets the stage for the broader ecological impacts of bioaccumulation and biomagnification.
Understanding Bioaccumulation
Bioaccumulation is a critical environmental concept that highlights the gradual buildup of toxic substances within organisms, posing significant risks to both individual health and ecosystem balance. To fully grasp this phenomenon, it is essential to delve into three key aspects: the definition and mechanisms of bioaccumulation, the various types that occur, and the factors that influence this process. Understanding these elements provides a comprehensive view of how bioaccumulation affects organisms and ecosystems. Starting with the **definition and mechanisms**, we explore how bioaccumulation occurs through the absorption of substances from the environment, which can then be stored in tissues or metabolized. This foundational knowledge sets the stage for understanding the broader implications of bioaccumulation. By examining the different **types of bioaccumulation**, such as bioconcentration and biomagnification, we can see how these processes vary across different species and environments. Additionally, recognizing the **factors influencing bioaccumulation**, including chemical properties, environmental conditions, and biological characteristics, helps in predicting and mitigating its effects. In this article, we will begin by defining bioaccumulation and explaining its underlying mechanisms, providing a solid foundation for further exploration into its types and influencing factors.
Definition and Mechanisms
**Definition and Mechanisms** Bioaccumulation, a critical concept in environmental science, refers to the process by which substances, particularly pollutants and toxins, accumulate in living organisms over time. This phenomenon occurs when the rate of uptake of these substances exceeds the rate of elimination, leading to a gradual increase in their concentration within the organism. The mechanisms behind bioaccumulation are multifaceted and involve several key processes. At its core, bioaccumulation begins with the uptake of substances from the environment. For aquatic organisms, this often occurs through the ingestion of contaminated food or direct absorption from water. In terrestrial organisms, substances can be ingested through food or absorbed through skin contact. Once inside the organism, these substances may be metabolized, stored, or excreted. However, many pollutants are lipophilic (fat-soluble) and tend to accumulate in fatty tissues because they are not easily metabolized or excreted. One of the primary mechanisms driving bioaccumulation is the concept of bioconcentration. Bioconcentration occurs when an organism absorbs a substance directly from its environment at a rate that exceeds its ability to eliminate it. This process is particularly significant in aquatic ecosystems where fish and other aquatic organisms can absorb pollutants such as pesticides and heavy metals directly from the water. Another mechanism is biomagnification, which is closely related to bioaccumulation but involves the increase in concentration of substances as they move up the food chain. In this process, organisms at higher trophic levels accumulate higher concentrations of pollutants because they consume large quantities of lower-trophic-level organisms that already contain these substances. For example, a fish that eats smaller fish contaminated with mercury will accumulate higher levels of mercury than those smaller fish. The persistence and lipophilicity of a substance also play crucial roles in bioaccumulation. Persistent organic pollutants (POPs), such as DDT and PCBs, are particularly problematic because they do not break down easily in the environment and tend to accumulate in fatty tissues. Their lipophilic nature allows them to dissolve in fats and oils, making them difficult for organisms to eliminate. Understanding these mechanisms is essential for mitigating the adverse effects of bioaccumulation on ecosystems and human health. By recognizing how pollutants enter and accumulate within organisms, scientists can develop strategies to reduce exposure and promote environmental health. For instance, regulating the use of persistent pollutants and implementing measures to clean up contaminated environments can help reduce bioaccumulation rates. Additionally, public health initiatives aimed at reducing human exposure to bioaccumulated toxins through dietary advisories and safe handling practices are critical for protecting human well-being. In summary, bioaccumulation is a complex process driven by various mechanisms including bioconcentration, biomagnification, persistence, and lipophilicity. Recognizing these factors is vital for addressing the environmental and health impacts associated with this phenomenon. By understanding how substances accumulate in organisms, we can better manage our interactions with the environment to ensure a healthier planet for all living beings.
Types of Bioaccumulation
Bioaccumulation, a critical process in environmental science, refers to the gradual accumulation of substances, such as chemicals and heavy metals, within an organism over time. This phenomenon can occur through various pathways and is categorized into different types based on the mechanisms and sources of accumulation. **1. Bioconcentration:** This type of bioaccumulation occurs when an organism absorbs a substance directly from its environment, such as water or air, without any dietary intake. For example, fish may absorb pesticides or industrial chemicals from contaminated water, leading to their accumulation in the fish's tissues. Bioconcentration is particularly significant in aquatic ecosystems where organisms are constantly exposed to pollutants. **2. Biomagnification:** Often confused with bioaccumulation, biomagnification is a specific type where the concentration of a substance increases as it moves up the food chain. This process is most evident in aquatic food webs where smaller organisms like plankton and fish absorb pollutants from their environment. As larger predators consume these smaller organisms, the pollutants are transferred and concentrated, resulting in higher levels in top predators such as sharks, eagles, or polar bears. **3. Bioaccumulation from Sediments:** In some cases, bioaccumulation can occur through the ingestion of contaminated sediments. For instance, benthic organisms like mussels and clams filter feed on sediment particles that may contain pollutants like heavy metals or organic compounds. These substances then accumulate in the tissues of these organisms. **4. Trophic Transfer:** This type involves the transfer of accumulated substances through the food chain but is more specific to the transfer between different trophic levels rather than just an increase in concentration. For example, when a herbivore ingests plants contaminated with pesticides, these substances are transferred to carnivores that consume the herbivore. **5. Maternal Transfer:** Some substances can be transferred from mother to offspring during reproduction. This form of bioaccumulation is particularly concerning for species where maternal transfer can lead to high levels of contaminants in offspring even before they are exposed to their environment. For example, DDT and PCBs have been shown to be transferred from mother birds to their eggs, affecting the development and survival of the chicks. Understanding these different types of bioaccumulation is crucial for assessing environmental health and developing strategies for mitigating the impacts of pollutants on ecosystems. Each type highlights a unique pathway through which substances can accumulate within organisms, emphasizing the need for comprehensive approaches to environmental monitoring and pollution control. By recognizing these mechanisms, scientists and policymakers can better address the challenges posed by bioaccumulation and work towards preserving ecological balance and human health.
Factors Influencing Bioaccumulation
Bioaccumulation, the process by which substances, particularly pollutants, build up in organisms over time, is influenced by a complex array of factors. These factors can be broadly categorized into environmental, biological, and chemical aspects. **Environmental Factors** play a crucial role; for instance, the availability and concentration of the substance in the environment directly impact the rate of bioaccumulation. Water and soil quality, as well as the presence of other pollutants, can also affect how readily an organism absorbs a substance. Temperature and pH levels in the environment can alter the solubility and bioavailability of chemicals, thereby influencing their uptake by organisms. **Biological Factors** are equally significant. The species-specific characteristics of an organism, such as its metabolic rate, feeding habits, and lifespan, determine how efficiently it can accumulate substances. For example, organisms with slower metabolic rates may accumulate more substances over time because they have fewer mechanisms to break down or excrete these substances. Additionally, the position of an organism in the food chain (trophic level) is critical; higher trophic levels often exhibit higher levels of bioaccumulation due to biomagnification—the process where substances become more concentrated as they move up the food chain. **Chemical Properties** of the substance itself are also pivotal. Lipophilicity (the tendency to dissolve in fats, oils, and non-polar solvents) is a key factor; substances with high lipophilicity tend to accumulate more readily in fatty tissues of organisms. Molecular weight and volatility also influence bioaccumulation; smaller molecules can more easily penetrate biological membranes, while less volatile substances are less likely to be exhaled or otherwise eliminated from the body. Furthermore, the persistence of a chemical in the environment—its resistance to degradation—significantly affects its potential for bioaccumulation. Understanding these factors is essential for predicting and mitigating the impacts of bioaccumulation on ecosystems and human health. For instance, knowing that certain chemicals are highly lipophilic and persistent can inform policies on their use and disposal. Similarly, recognizing the importance of trophic level in bioaccumulation helps in identifying species that are most at risk and in developing strategies for monitoring and managing these risks. By considering these multifaceted influences, scientists and policymakers can better address the challenges posed by bioaccumulation and work towards a more sustainable and healthier environment.
Exploring Biomagnification
Biomagnification, a critical environmental phenomenon, highlights the accumulation of toxic substances within the food chain, posing significant risks to ecosystems and human health. This complex process involves the gradual increase in concentration of pollutants as they move from lower to higher trophic levels, making it a pressing issue that demands thorough understanding. To delve into this topic, we will explore three key aspects: the definition and process of biomagnification, illustrative examples and case studies, and the broader environmental impact. First, we will define biomagnification and explain the intricate mechanisms by which it occurs, including the role of bioaccumulation and trophic transfer. This foundational knowledge is essential for grasping the subsequent sections. Next, we will examine real-world examples and case studies that demonstrate the effects of biomagnification, such as the infamous Minamata disease caused by mercury contamination. Finally, we will discuss the far-reaching environmental consequences of biomagnification, including its impact on biodiversity and human health. By understanding these facets, we can better appreciate the urgency of addressing this environmental challenge. Let us begin by defining and explaining the process of biomagnification, which sets the stage for a deeper exploration of this critical issue.
Definition and Process
**Definition and Process** Biomagnification, a critical component of the broader phenomenon of bioaccumulation, refers to the process by which the concentration of a substance, particularly toxic chemicals like pesticides and heavy metals, increases in the tissues of organisms as it moves up the food chain. This occurs because these substances are not readily broken down or excreted by organisms and instead accumulate in their bodies over time. The definition of biomagnification underscores its role in environmental science, highlighting how even low levels of contaminants in the environment can become highly concentrated and harmful at higher trophic levels. The process of biomagnification begins with the uptake of contaminants by primary producers such as plants and algae. These organisms absorb the substances from their environment, which can include water, soil, or air. Herbivores then consume these primary producers, ingesting the accumulated contaminants. As these herbivores are eaten by carnivores, the concentration of the contaminants increases because the carnivores are consuming multiple herbivores, each containing a certain amount of the substance. This sequential consumption leads to a significant increase in the concentration of contaminants at each higher trophic level. For instance, in aquatic ecosystems, small fish may absorb mercury from contaminated water. Larger fish that feed on these smaller fish will accumulate higher levels of mercury in their bodies. When these larger fish are consumed by apex predators such as sharks or humans, the mercury concentration can reach dangerous levels. This cascade effect is what makes biomagnification particularly concerning, as it can lead to severe health impacts on top predators and even humans who consume them. Understanding the process of biomagnification is crucial for environmental management and public health. It informs policies on pollution control and highlights the importance of monitoring and regulating the release of harmful substances into the environment. By recognizing how biomagnification works, scientists and policymakers can take proactive steps to mitigate its effects, protecting both ecosystems and human health from the adverse impacts of bioaccumulated toxins. This knowledge also underscores the interconnectedness of ecosystems and the need for holistic approaches to environmental conservation. In exploring biomagnification, we gain insights into the complex dynamics of ecological systems and the critical role that each species plays in maintaining or disrupting these delicate balances.
Examples and Case Studies
**Examples and Case Studies** Biomagnification, a critical aspect of bioaccumulation, can be vividly illustrated through several compelling examples and case studies that highlight its impact on ecosystems and human health. One of the most iconic cases is the story of DDT (dichlorodiphenyltrichloroethane) in the food chain. In the 1950s and 1960s, DDT was widely used as an insecticide, leading to its accumulation in soil, water, and organisms. This chemical was particularly persistent and lipophilic, making it prone to biomagnification. For instance, DDT would accumulate in plankton, which were then consumed by small fish. These fish, in turn, were eaten by larger fish and eventually by birds such as eagles and ospreys. The concentration of DDT increased significantly at each trophic level, causing severe reproductive issues in these birds, including thinning of eggshells and reduced fertility. This example underscores how biomagnification can lead to devastating ecological consequences. Another significant case study involves mercury contamination in aquatic ecosystems. Mercury, particularly methylmercury, is a potent neurotoxin that accumulates in fish and other aquatic organisms. In the Minamata Bay in Japan, industrial effluent containing mercury was discharged into the water, leading to severe biomagnification. Fish and shellfish accumulated high levels of methylmercury, which were then consumed by humans. The resulting Minamata disease caused neurological damage, birth defects, and even death among local residents. This tragedy highlights the critical need for stringent regulations on industrial waste to prevent such biomagnification events. The Arctic ecosystem provides another stark example of biomagnification. Persistent organic pollutants (POPs) such as PCBs (polychlorinated biphenyls) and dioxins are transported to the Arctic via atmospheric and oceanic currents. These chemicals accumulate in phytoplankton and zooplankton, which are then consumed by larger organisms like seals and polar bears. The Inuit people, who rely heavily on these marine mammals for food, are particularly vulnerable to the effects of biomagnified POPs. Studies have shown that Inuit mothers have some of the highest levels of PCBs and dioxins in their breast milk, posing significant health risks to their infants. In addition to these examples, biomagnification has also been observed in terrestrial ecosystems. For instance, the accumulation of heavy metals like cadmium and lead in soil can lead to their uptake by plants, which are then consumed by herbivores. These herbivores may be eaten by carnivores, resulting in higher concentrations of these metals at higher trophic levels. This process has been documented in various agricultural regions where soil contamination has led to elevated levels of heavy metals in livestock and eventually in human consumers. These case studies collectively emphasize the importance of understanding and mitigating biomagnification. By recognizing how pollutants move through ecosystems and accumulate at higher trophic levels, we can implement more effective strategies for environmental protection and public health. This includes stricter regulations on industrial emissions, sustainable agricultural practices, and education campaigns aimed at reducing exposure to bioaccumulative substances. Ultimately, addressing biomagnification requires a multifaceted approach that involves scientific research, policy changes, and community engagement to safeguard both ecosystems and human well-being.
Environmental Impact
The environmental impact of bioaccumulation and biomagnification is a critical concern that underscores the intricate and often detrimental relationships between organisms and their ecosystems. Bioaccumulation, the process by which substances, particularly pollutants, build up in an organism's body over time, can have far-reaching consequences. When these substances are transferred from one trophic level to the next through the food chain, a phenomenon known as biomagnification occurs. This amplification of toxic substances can lead to severe health issues for top predators and even humans who consume contaminated food. One of the most striking examples of biomagnification is the case of mercury in aquatic ecosystems. Mercury, a potent neurotoxin, enters water bodies through industrial emissions and runoff. It is then absorbed by phytoplankton and other microorganisms, which are consumed by zooplankton. As larger fish feed on these smaller organisms, mercury accumulates in their bodies. This process continues up the food chain, resulting in high concentrations of mercury in apex predators like sharks and tuna. Humans who consume these fish are at risk of mercury poisoning, which can cause neurological damage, birth defects, and other serious health problems. Another significant environmental impact is seen with persistent organic pollutants (POPs), such as DDT and PCBs. These chemicals are highly stable and resist degradation, allowing them to persist in the environment for decades. They bioaccumulate in fatty tissues of organisms and biomagnify through the food chain, affecting not only wildlife but also human health. For instance, polar bears have been found with high levels of POPs due to their position at the top of the Arctic food chain, leading to reproductive issues and other health problems. The impact of bioaccumulation and biomagnification extends beyond individual species to entire ecosystems. For example, the decline of bald eagles in the United States during the mid-20th century was largely attributed to DDT accumulation in their bodies, which caused thinning of eggshells and reduced reproductive success. The banning of DDT led to a significant recovery of bald eagle populations, highlighting the importance of regulatory measures in mitigating these environmental impacts. Moreover, climate change exacerbates these issues by altering ecosystems and increasing the mobility of pollutants. Rising temperatures can increase the volatility of certain chemicals, making them more likely to be released into the atmosphere and subsequently deposited in remote areas. This can lead to increased bioaccumulation in sensitive ecosystems like the Arctic, where indigenous communities rely heavily on wildlife for sustenance. In conclusion, the environmental impact of bioaccumulation and biomagnification is multifaceted and far-reaching. It underscores the need for stringent regulations on industrial emissions, careful management of chemical use, and continued research into the dynamics of these processes. By understanding and addressing these issues, we can work towards preserving the health of our ecosystems and ensuring a safer environment for both wildlife and human populations.
Consequences and Mitigation Strategies
The consequences of environmental pollution are multifaceted and far-reaching, impacting both human health and ecological balance. Understanding these consequences is crucial for developing effective mitigation strategies. This article delves into three key areas: Health and Ecological Risks, Regulatory Measures and Policies, and Techniques for Reducing Bioaccumulation and Biomagnification. By examining the health and ecological risks associated with pollution, we can better comprehend the immediate and long-term effects on ecosystems and human populations. Regulatory measures and policies play a vital role in enforcing standards that limit pollution, while techniques for reducing bioaccumulation and biomagnification offer practical solutions to mitigate these harmful processes. Each of these aspects is interconnected, highlighting the need for a comprehensive approach to address environmental degradation. This article will first explore the Health and Ecological Risks posed by pollution, setting the stage for a deeper discussion on regulatory measures and mitigation techniques.
Health and Ecological Risks
Health and ecological risks are paramount concerns when discussing bioaccumulation and biomagnification. Bioaccumulation, the process by which substances gradually accumulate in an organism's body, and biomagnification, the increase in concentration of these substances as they move up the food chain, pose significant threats to both human health and ecosystem integrity. At the individual level, bioaccumulated toxins such as heavy metals (like mercury and lead), pesticides (like DDT), and industrial chemicals (like PCBs) can lead to a range of health issues. For instance, exposure to mercury can result in neurological damage, developmental delays in children, and cardiovascular problems. Similarly, long-term exposure to pesticides has been linked to increased risks of cancer, reproductive issues, and neurological disorders. These toxins can enter the human body through consumption of contaminated food, particularly fish and other seafood that have accumulated high levels of these substances. On an ecological scale, the impacts are equally profound. Biomagnification exacerbates the problem by concentrating these toxins in higher trophic levels, making apex predators like eagles, sharks, and polar bears particularly vulnerable. This can lead to population declines or even extinctions if left unchecked. For example, the decline of bald eagles in the 1960s was largely attributed to DDT biomagnification, which caused thinning of eggshells and reduced reproductive success. Ecosystems suffer broader disruptions as well; the loss of key species can destabilize food webs and alter nutrient cycling patterns, leading to cascading effects throughout entire ecosystems. Moreover, these risks are not limited to wildlife; they also have significant economic implications. Contaminated fish and seafood can lead to costly recalls and damage to fisheries industries. Additionally, the health impacts on humans translate into substantial medical expenses and lost productivity. Therefore, it is crucial to implement effective mitigation strategies to reduce bioaccumulation and biomagnification. These include stringent regulations on the release of harmful substances into the environment, enhanced monitoring of food chains for toxic levels, public education campaigns about safe consumption practices, and the development of cleaner technologies that minimize pollution. By addressing these issues proactively, we can protect both human health and ecological balance, ensuring a safer and more sustainable future for all.
Regulatory Measures and Policies
Regulatory measures and policies play a crucial role in mitigating the consequences of bioaccumulation and biomagnification, ensuring the protection of both human health and the environment. Governments and international organizations have implemented various regulations to control the release of pollutants into ecosystems. For instance, the Stockholm Convention on Persistent Organic Pollutants (POPs) aims to eliminate or restrict the production and use of substances known to bioaccumulate and biomagnify, such as DDT, PCBs, and dioxins. Similarly, the European Union's REACH (Registration, Evaluation, Authorisation, and Restriction of Chemicals) regulation requires thorough assessment of chemical substances before they are allowed on the market, ensuring that only safe chemicals are used in industrial processes. In addition to these international agreements, national laws and policies also contribute significantly. For example, the Clean Water Act in the United States sets standards for water quality and regulates the discharge of pollutants into water bodies, thereby reducing the amount of contaminants that can accumulate in aquatic organisms. The Safe Drinking Water Act further ensures that drinking water supplies are free from harmful levels of bioaccumulative substances. Moreover, regulatory bodies like the Environmental Protection Agency (EPA) in the U.S. and the European Chemicals Agency (ECHA) in Europe enforce strict guidelines on industrial practices to minimize environmental pollution. These agencies conduct regular monitoring and enforcement activities to ensure compliance with established standards. Public education campaigns are also integral to these policies, raising awareness about the dangers of bioaccumulation and biomagnification among consumers and industries alike. Economic incentives are another tool used by regulatory bodies to encourage compliance. For example, tax breaks or subsidies may be offered to companies that adopt cleaner technologies or reduce their emissions of bioaccumulative substances. Conversely, fines and penalties can be imposed on non-compliant entities, providing a financial deterrent against pollution. Furthermore, research funding allocated by governments supports scientific studies aimed at understanding the mechanisms of bioaccumulation and biomagnification. This research informs policy decisions by providing data on the most effective strategies for mitigating these phenomena. Collaborative efforts between scientists, policymakers, and industry stakeholders are essential for developing comprehensive regulatory frameworks that balance economic needs with environmental protection. In summary, robust regulatory measures and policies are vital for addressing the consequences of bioaccumulation and biomagnification. By setting strict standards, enforcing compliance, promoting public awareness, offering economic incentives, and supporting scientific research, these regulations help safeguard ecosystems and human health from the adverse effects of persistent pollutants. As our understanding of these phenomena evolves, so too must our regulatory approaches to ensure effective mitigation strategies are in place.
Techniques for Reducing Bioaccumulation and Biomagnification
Techniques for reducing bioaccumulation and biomagnification are crucial for mitigating the adverse environmental and health impacts associated with these processes. One of the most effective strategies is the implementation of stringent regulations and policies to limit the release of persistent organic pollutants (POPs) and other hazardous substances into the environment. Governments and international organizations can enforce strict emission standards, ban the use of certain chemicals, and promote sustainable practices in industries such as agriculture, manufacturing, and waste management. For instance, the Stockholm Convention has played a significant role in regulating POPs globally, leading to a reduction in their environmental presence. Another key technique involves the adoption of cleaner technologies and best management practices. Industries can transition to greener alternatives that minimize the production and release of bioaccumulative substances. For example, replacing traditional pesticides with integrated pest management (IPM) strategies can significantly reduce chemical residues in agricultural products. Similarly, implementing advanced wastewater treatment technologies can help remove contaminants from effluents before they enter water bodies. Public education and awareness campaigns are also vital in reducing bioaccumulation and biomagnification. Educating consumers about the risks associated with certain products and promoting eco-friendly choices can drive market demand towards safer alternatives. This can include labeling products that contain bioaccumulative substances, encouraging the use of organic produce, and supporting companies that adhere to sustainable practices. In addition, scientific research and monitoring programs are essential for understanding and addressing bioaccumulation and biomagnification. Continuous monitoring of environmental samples and biological tissues helps in identifying hotspots and tracking changes over time. This data can inform policy decisions and guide targeted interventions. Furthermore, research into new technologies and methods for degrading or removing bioaccumulative substances from the environment can provide innovative solutions. Bioremediation, which involves using living organisms or their enzymes to degrade pollutants, is another promising technique. Microorganisms such as bacteria and fungi can be engineered or naturally selected to break down persistent pollutants, thereby reducing their bioavailability. Phytoremediation, using plants to absorb and metabolize contaminants, is another effective method that can be applied in contaminated soil and water. Finally, adopting a circular economy approach can significantly reduce the generation of bioaccumulative substances at the source. This involves designing products and systems that are restorative and regenerative by design, aiming to keep resources in use for as long as possible, extract the maximum value from them in use, recover and regenerate materials at the end of each service life. By minimizing waste and promoting recycling, we can reduce the overall load of pollutants entering the environment. In conclusion, a multi-faceted approach combining regulatory measures, technological innovations, public awareness, scientific research, bioremediation techniques, and circular economy principles is necessary to effectively reduce bioaccumulation and biomagnification. These strategies not only protect ecosystems but also safeguard human health by minimizing exposure to harmful substances that accumulate in the food chain.