What Organelles Are Only In Plant Cells
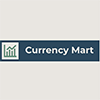
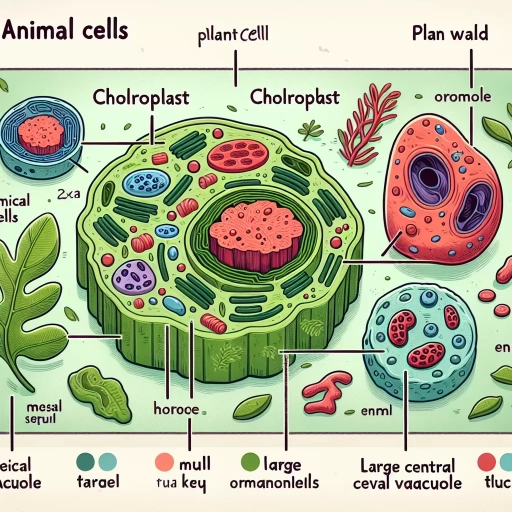
Plant cells, the fundamental building blocks of plant life, are distinguished by several unique organelles that set them apart from animal cells. These specialized structures play crucial roles in the survival, growth, and reproduction of plants. In this article, we will delve into the world of plant-specific organelles, exploring their distinct characteristics, functions, and importance. We will first identify the unique organelles found exclusively in plant cells, highlighting their structural and functional differences from those in animal cells. Next, we will examine the specific functions these organelles perform, such as photosynthesis, storage, and defense mechanisms. Finally, we will discuss the importance of these organelles in plant biology, including their impact on plant development and adaptation to various environments. By understanding these plant-specific organelles, we gain insights into the intricate mechanisms that enable plants to thrive. Let us begin by identifying the unique organelles in plant cells, which form the foundation of their specialized biological processes.
Unique Organelles in Plant Cells
Plant cells are distinguished by several unique organelles that play crucial roles in their survival, growth, and function. Among these, chloroplasts, vacuoles, and the cell wall stand out for their specialized contributions. Chloroplasts, for instance, are the powerhouses of photosynthesis, converting sunlight into energy that fuels the plant's metabolic processes. Vacuoles serve as storage compartments, regulating the cell's internal environment by managing water, nutrients, and waste products. The cell wall, composed primarily of cellulose, provides structural support and protection against external pressures. Each of these organelles works in harmony to ensure the plant cell operates efficiently. Understanding these components is essential for appreciating the intricate mechanisms that govern plant life. Let's delve deeper into the first of these vital organelles: chloroplasts. These tiny green structures are not only responsible for photosynthesis but also house genetic material and participate in various other cellular processes, making them a fascinating subject of study.
Chloroplasts
Chloroplasts are one of the most distinctive and crucial organelles found exclusively in plant cells, playing a pivotal role in the process of photosynthesis. These organelles are essentially the powerhouses of plant cells, responsible for converting light energy from the sun into chemical energy in the form of glucose. Structurally, chloroplasts are enveloped by a double membrane, with the inner membrane folded into a series of flattened sacs known as thylakoids. These thylakoids are stacked together to form grana, which house the pigment chlorophyll and other accessory pigments that capture light energy. The chloroplast's ability to perform photosynthesis is due to its unique composition and structure. The stroma, the fluid-filled space between the thylakoids, contains enzymes and other molecules necessary for the Calvin cycle, where carbon dioxide is fixed into organic compounds using the energy captured from light. Chloroplasts also contain their own DNA (plastome) and ribosomes, allowing them to synthesize some of their own proteins independently of the cell's nucleus. This autonomy is a testament to their evolutionary origin from cyanobacteria, which were engulfed by early eukaryotic cells millions of years ago. In addition to their primary function in photosynthesis, chloroplasts are involved in various other metabolic processes such as the synthesis of amino acids, lipids, and pigments like carotenoids and anthocyanins. These pigments not only contribute to the coloration of plants but also protect them from excessive light energy. Chloroplasts also play a role in regulating plant growth and development through signaling pathways that respond to environmental cues such as light intensity and duration. The dynamic nature of chloroplasts is evident in their ability to move within the cell in response to light conditions—a process known as phototropism. This movement ensures that chloroplasts are optimally positioned to capture light, thereby maximizing photosynthetic efficiency. Furthermore, chloroplasts can differentiate into other types of plastids depending on the cell's needs; for example, they can become amyloplasts for starch storage or chromoplasts for pigment synthesis. In summary, chloroplasts are indispensable components of plant cells, enabling plants to harness solar energy and convert it into a form that supports their growth and development. Their complex structure, autonomous genetic capabilities, and multifaceted roles underscore their significance as unique organelles that set plant cells apart from those of other eukaryotes. Understanding chloroplasts provides valuable insights into the intricate mechanisms that allow plants to thrive in diverse environments, highlighting their critical role in sustaining life on Earth.
Vacuoles
**Unique Organelles in Plant Cells** ### **Vacuoles** Vacuoles are one of the most distinctive and essential organelles found exclusively in plant cells, playing a pivotal role in their survival and function. These membrane-bound organelles are characterized by their large size and versatility, often occupying up to 90% of the cell's volume. Unlike animal cells, which have smaller, more numerous vacuoles, plant cells typically contain a single large central vacuole that dominates the cell's interior. The primary function of vacuoles is to store water, nutrients, and waste products. They act as reservoirs for essential minerals and ions, helping to maintain cellular homeostasis and regulate the cell's osmotic balance. This storage capacity is crucial for plant cells, as it allows them to maintain turgor pressure, which is necessary for plant growth and structural support. For instance, the water stored in vacuoles helps to keep the plant cell rigid, contributing to the overall structure and posture of the plant. In addition to their role in water storage, vacuoles are also involved in the detoxification process. They can sequester harmful substances such as heavy metals and xenobiotics, protecting the cell from potential damage. This detoxification mechanism is vital for plant survival, especially in environments where pollutants are prevalent. Vacuoles also serve as recycling centers within the cell. They contain digestive enzymes that break down and recycle cellular waste, including proteins, carbohydrates, and other macromolecules. This process helps in the efficient reuse of cellular components, which is particularly important for plants that often face nutrient limitations. Moreover, vacuoles play a significant role in plant defense mechanisms. They can store defense compounds such as alkaloids and glycosides that help protect the plant against pathogens and herbivores. For example, certain plants accumulate toxic compounds in their vacuoles to deter herbivores, thereby enhancing their survival chances. The structure of vacuoles is dynamic and can change in response to environmental conditions. During times of drought or stress, vacuoles can shrink or expand to help the cell conserve water and maintain cellular integrity. This adaptability underscores the critical importance of vacuoles in plant cell biology. In summary, vacuoles are multifunctional organelles that are central to the functioning of plant cells. Their roles in water storage, detoxification, recycling, and defense make them indispensable for plant survival and growth. The unique characteristics and functions of vacuoles highlight their significance as one of the key organelles that distinguish plant cells from animal cells.
Cell Wall
The cell wall is a unique and crucial organelle found exclusively in plant cells, playing a pivotal role in their structure, function, and survival. Unlike animal cells, which lack this rigid layer, the plant cell wall provides essential support and protection. Composed primarily of cellulose, a polysaccharide made from glucose molecules, the cell wall also contains other components such as hemicellulose, pectin, and sometimes lignin. These components work together to form a robust yet dynamic structure that maintains the cell's shape and prevents it from bursting under osmotic pressure. The primary function of the cell wall is to provide mechanical strength and rigidity, allowing plants to grow upright against gravity. This is particularly important for tall plants and trees, which would otherwise collapse under their own weight. Additionally, the cell wall acts as a barrier against pathogens and physical damage, safeguarding the delicate cellular contents within. It also plays a critical role in cell signaling and communication by influencing cell growth patterns and responses to environmental stimuli. The composition of the cell wall varies depending on the type of plant cell. For instance, primary cell walls are thinner and more flexible, found in growing cells where they allow for expansion. Secondary cell walls, on the other hand, are thicker and more rigid, often found in mature cells such as those in wood. The presence of lignin in secondary cell walls further enhances their strength but reduces their flexibility. Beyond its structural role, the cell wall is also involved in various physiological processes. It can regulate water and nutrient uptake by controlling the passage of substances through its matrix. Moreover, it participates in plant defense mechanisms by producing chemical signals that alert neighboring cells to potential threats. In terms of development, the formation of the cell wall is tightly regulated by a complex interplay of genetic and environmental factors. During cell division, new cell walls are synthesized through a process known as cytokinesis, ensuring that each daughter cell receives an intact wall. This process involves coordinated efforts from multiple organelles including the Golgi apparatus and endoplasmic reticulum. In summary, the cell wall is an indispensable organelle unique to plant cells that provides structural support, protection, and plays a multifaceted role in plant physiology. Its dynamic nature allows it to adapt to changing environmental conditions while maintaining the integrity of the plant cell. This specialized structure underscores the remarkable diversity and adaptability of plant cells compared to their animal counterparts.
Functions of Plant-Specific Organelles
Plant cells are equipped with a variety of organelles that perform specialized functions crucial for the survival and growth of plants. These organelles work in harmony to ensure the plant's overall health and productivity. One of the most critical functions is **Photosynthesis in Chloroplasts**, where chloroplasts convert light energy into chemical energy, producing the glucose that fuels plant growth. Additionally, **Storage and Recycling by Vacuoles** play a vital role in storing nutrients, waste products, and recycling cellular components, maintaining cellular homeostasis. Furthermore, **Structural Support by Cell Walls** provides the necessary rigidity and protection to plant cells, allowing them to withstand environmental stresses. Understanding these functions is essential for appreciating the intricate mechanisms that underpin plant biology. By delving into these specific roles, we can gain a deeper insight into how plants thrive in diverse environments. Let's begin by exploring one of the most fundamental processes: **Photosynthesis in Chloroplasts**.
Photosynthesis in Chloroplasts
Photosynthesis, the cornerstone of life on Earth, is predominantly carried out within the chloroplasts of plant cells. These organelles are specialized to harness light energy from the sun and convert it into chemical energy through a complex series of reactions. Chloroplasts are descendants of ancient cyanobacteria that were engulfed by early plant cells, a process known as endosymbiosis. Over time, these cyanobacteria evolved into chloroplasts, retaining their ability to perform photosynthesis while integrating with the host cell's metabolic processes. The structure of chloroplasts is highly adapted for their function. They contain two membranes: an outer membrane that allows certain molecules to pass through and an inner membrane that regulates the movement of substances. Inside the chloroplast, there is a gel-like substance called stroma, where the Calvin cycle takes place, and thylakoids, which are stacked into grana. Thylakoids are the site of the light-dependent reactions, where light is absorbed by pigments such as chlorophyll and converted into ATP and NADPH. The process of photosynthesis in chloroplasts can be divided into two main stages: the light-dependent reactions and the light-independent reactions (Calvin cycle). In the light-dependent reactions, light energy excites electrons in chlorophyll molecules, leading to the formation of ATP and NADPH. These energy-rich molecules are then used in the Calvin cycle to fix carbon dioxide into organic compounds, such as glucose, which serves as a primary source of energy and building blocks for plant growth. Chloroplasts also contain various pigments besides chlorophyll, including carotenoids and anthocyanins, which play roles in light absorption and protection against excessive light. The flexibility in pigment composition allows plants to adapt to different light conditions, ensuring optimal photosynthetic efficiency. In addition to their role in photosynthesis, chloroplasts are involved in other critical functions such as the synthesis of amino acids, lipids, and pigments. They also play a role in plant defense mechanisms by producing signaling molecules that trigger responses to pathogens and environmental stresses. The importance of chloroplasts extends beyond individual plant cells; they are crucial for sustaining life on Earth. By converting carbon dioxide into oxygen and organic compounds, chloroplasts support the food chain and maintain atmospheric balance. Understanding the intricacies of photosynthesis in chloroplasts not only deepens our appreciation for plant biology but also informs strategies for improving crop yields, developing more efficient solar cells, and addressing global challenges related to food security and climate change. In summary, chloroplasts are highly specialized organelles that enable plants to perform photosynthesis, a process essential for their survival and the sustenance of nearly all life forms. Their unique structure and function make them a fascinating subject of study, highlighting the intricate relationships between light, energy, and life.
Storage and Recycling by Vacuoles
**Storage and Recycling by Vacuoles** In plant cells, vacuoles play a pivotal role in storage and recycling, making them indispensable for the cell's overall health and function. These membrane-bound organelles are often the largest structures within a plant cell, occupying up to 90% of its volume. Vacuoles serve as multifunctional compartments that store a variety of substances, including water, salts, minerals, sugars, and even waste products. This storage capacity helps maintain cellular homeostasis by regulating the concentration of ions and nutrients within the cell. For instance, vacuoles can sequester excess ions to prevent toxicity, thereby protecting the cell from damage. Beyond storage, vacuoles are also involved in recycling processes. They contain various enzymes that break down and recycle cellular waste and damaged organelles. This process is crucial for cellular maintenance and renewal. Vacuoles can engulf and digest damaged or dysfunctional organelles through a process known as autophagy, which helps maintain cellular integrity and efficiency. Additionally, vacuoles participate in the degradation of proteins and other macromolecules, ensuring that the cell remains free from harmful by-products. The role of vacuoles in recycling is further underscored by their involvement in the breakdown of cellular components during times of stress or nutrient scarcity. For example, during periods of drought or nutrient deficiency, vacuoles can release stored nutrients back into the cytoplasm to support essential cellular functions. This adaptive mechanism allows plants to survive under adverse conditions by optimizing resource allocation. Moreover, vacuoles contribute to plant defense mechanisms by storing defense compounds such as alkaloids and phenolics. These compounds can be released rapidly in response to pathogen attacks or herbivore damage, providing an immediate line of defense against potential threats. The ability of vacuoles to store and release these compounds highlights their dynamic role in plant immunity. In summary, the functions of vacuoles in storage and recycling are fundamental to the survival and adaptability of plant cells. By managing the storage and release of essential nutrients, ions, and waste products, vacuoles ensure that plant cells operate efficiently under various environmental conditions. Their role in autophagy and defense further underscores their importance as critical organelles unique to plant cells.
Structural Support by Cell Walls
In the intricate world of plant cells, structural support is a critical function that ensures the integrity and stability of the cell, and this is primarily achieved through the cell wall. Unlike animal cells, which lack a rigid cell wall, plant cells are encased in a robust, dynamic structure composed of cellulose, hemicellulose, and pectin. This complex matrix provides mechanical strength and protection against external forces such as wind, rain, and pathogens. The cell wall acts as a scaffold that maintains cell shape and prevents excessive water uptake, thereby preventing the cell from bursting under turgor pressure. Additionally, it plays a pivotal role in plant growth by regulating cell expansion through the controlled loosening and tightening of its components. For instance, during cell elongation, enzymes like expansins and xyloglucan endotransglucosylase/hydrolases (XTHs) modify the cell wall structure to allow for increased cell size without compromising its strength. This dynamic interplay between cell wall components and enzymes is essential for plant development, enabling plants to grow upright and support their own weight as well as that of fruits and flowers. Furthermore, the cell wall serves as a barrier against pathogens and pests by incorporating defense-related compounds such as lignin and callose, which enhance its resistance to infection. In summary, the cell wall is a multifunctional organelle that provides structural support, regulates growth, and offers protection, making it an indispensable component of plant cells and a key factor in their ability to thrive in diverse environments.
Importance of Plant-Specific Organelles
Plant-specific organelles are crucial components of plant cells, playing pivotal roles in various aspects of plant biology. These specialized structures are essential for the overall health, survival, and adaptability of plants. The importance of plant-specific organelles can be understood through their role in plant growth and development, their impact on plant metabolism, and their adaptation to environmental conditions. For instance, organelles such as chloroplasts and amyloplasts are vital for photosynthesis and starch storage, respectively, which are fundamental to plant growth. Additionally, these organelles influence metabolic pathways that produce essential nutrients and energy for the plant. Furthermore, they enable plants to adapt to diverse environmental conditions by regulating stress responses and optimizing resource allocation. Understanding these functions is critical for advancing agricultural practices and improving crop resilience. By delving into the role of plant-specific organelles in plant growth and development, we can uncover the intricate mechanisms that underpin the life cycle of plants, setting the stage for a deeper exploration of their multifaceted importance. This begins with examining their role in plant growth and development, where these organelles orchestrate the complex processes that transform a seed into a mature plant.
Role in Plant Growth and Development
In the intricate world of plant biology, the role of plant-specific organelles in growth and development is paramount. These specialized organelles, unique to plant cells, play critical roles in various physiological processes that distinguish plants from other eukaryotic organisms. One of the most significant plant-specific organelles is the chloroplast, which is the site of photosynthesis. Chloroplasts contain chlorophyll and other pigments that capture light energy, converting it into chemical energy through the process of photosynthesis. This energy is then stored in the form of glucose, which serves as a primary source of energy and organic carbon for plant growth and development. Without chloroplasts, plants would be unable to produce their own food, rendering them dependent on external sources of nutrition. Another crucial organelle is the vacuole, which plays a multifaceted role in plant cell function. Vacuoles are large storage compartments that can occupy up to 90% of the cell's volume. They store water, nutrients, and waste products, helping to maintain cell turgor pressure and structural integrity. Additionally, vacuoles are involved in the regulation of ion balance and pH within the cell, ensuring optimal conditions for metabolic processes. They also contain enzymes that break down and recycle cellular components, contributing to cellular homeostasis and nutrient recycling. Plastids, including amyloplasts and chromoplasts, are also vital for plant growth. Amyloplasts are responsible for starch storage and are particularly important in tubers and seeds, where they serve as a reservoir of energy for germination and seedling establishment. Chromoplasts, on the other hand, contain pigments such as carotenoids and betalains that contribute to the coloration of flowers, fruits, and other plant parts, which is essential for attracting pollinators and dispersers. The endoplasmic reticulum (ER) in plant cells, while not unique to plants, has specialized functions that are critical for plant development. The ER is involved in lipid synthesis, protein folding, and transport within the cell. In plants, it also plays a role in the synthesis of cell wall components and the regulation of calcium ions, which are essential for signaling pathways that control various aspects of plant growth and response to environmental stimuli. Furthermore, peroxisomes in plant cells are involved in photorespiration—a process that occurs in C3 plants under high light conditions—and the breakdown of fatty acids. These organelles help mitigate oxidative stress by detoxifying harmful by-products of photosynthesis, ensuring that the plant can maintain optimal metabolic activity. In summary, the unique organelles found in plant cells are indispensable for their growth and development. From energy production through photosynthesis in chloroplasts to storage and homeostasis functions in vacuoles, each organelle contributes to the complex interplay of processes that allow plants to thrive. Understanding these organelles not only deepens our appreciation of plant biology but also has practical implications for agriculture, biotechnology, and environmental science. By recognizing the importance of these specialized structures, we can better manage and enhance plant productivity, resilience, and adaptability in diverse environments.
Impact on Plant Metabolism
The impact on plant metabolism due to the presence of plant-specific organelles is profound and multifaceted. These organelles, such as chloroplasts, vacuoles, and amyloplasts, play critical roles in various metabolic processes that are unique to plants. Chloroplasts, for instance, are the site of photosynthesis, where light energy is converted into chemical energy through the synthesis of glucose from carbon dioxide and water. This process not only provides the plant with its primary source of energy but also produces oxygen as a byproduct, which is essential for the survival of nearly all living organisms. The efficiency of photosynthesis directly influences the plant's growth rate, productivity, and overall health. Vacuoles, another plant-specific organelle, are involved in several key metabolic functions. They serve as storage compartments for nutrients, waste products, and even toxic substances that the plant might encounter. Vacuoles also play a crucial role in maintaining cellular turgor pressure by regulating the amount of water and ions within the cell. This turgor pressure is essential for plant structure and support, allowing plants to stand upright and resist environmental stresses. Additionally, vacuoles are involved in the breakdown and recycling of cellular components, contributing to cellular homeostasis and nutrient cycling within the plant. Amyloplasts, found in non-photosynthetic tissues like roots and tubers, are specialized for starch synthesis and storage. These organelles are vital for the plant's ability to store energy in the form of starch, which can be mobilized when needed. This stored energy is crucial for plant development, particularly during periods of rapid growth or when external resources are limited. The presence of amyloplasts ensures that plants can sustain their metabolic activities even under adverse conditions. Furthermore, these organelles interact dynamically with other cellular components to optimize metabolic pathways. For example, chloroplasts communicate with the nucleus and other organelles through complex signaling networks to adjust photosynthetic rates based on environmental conditions. Similarly, vacuoles coordinate with the cytosol and other organelles to manage ion fluxes and maintain cellular balance. In summary, the unique set of organelles found in plant cells significantly impacts plant metabolism by facilitating photosynthesis, storing nutrients and waste, maintaining cellular structure, and optimizing energy storage. These specialized organelles enable plants to thrive in diverse environments and underscore the importance of understanding their functions for improving crop productivity and resilience in agriculture. By recognizing the critical roles these organelles play in plant metabolism, scientists can develop targeted strategies to enhance plant performance and address global challenges related to food security and sustainability.
Adaptation to Environmental Conditions
Adaptation to environmental conditions is a crucial aspect of plant biology, and it is significantly facilitated by the presence of plant-specific organelles. These specialized organelles enable plants to thrive in diverse and often challenging environments. For instance, chloroplasts, which are unique to plant cells, are essential for photosynthesis—the process by which plants convert light energy into chemical energy. Chloroplasts contain pigments such as chlorophyll that absorb light, allowing plants to harness solar energy and produce the glucose they need for growth and development. This adaptation is pivotal in allowing plants to survive and reproduce in environments ranging from dense forests to arid deserts. Another key plant-specific organelle is the vacuole, which plays a critical role in maintaining cellular homeostasis and adapting to environmental stresses. Vacuoles can store water, ions, and various metabolites, helping plants regulate their internal environment despite external fluctuations. During droughts, for example, vacuoles can release stored water to maintain cellular turgor pressure, ensuring the plant remains functional. Additionally, vacuoles can sequester toxic substances, protecting the cell from damage caused by environmental pollutants or pathogens. Plastids, a broader category that includes chloroplasts, also contribute to environmental adaptation. Amyloplasts, a type of plastid found in root cells, store starch and help plants survive periods of low light or nutrient scarcity. Similarly, chromoplasts, which contain pigments like carotenoids and betalains, are responsible for the vibrant colors of fruits and flowers, attracting pollinators and seed dispersers—crucial for plant reproduction. The presence of these organelles also allows plants to respond dynamically to environmental cues. For example, the movement of chloroplasts within leaf cells (phototropism) ensures that they are optimally positioned to capture light, maximizing photosynthetic efficiency. This ability to adapt to changing light conditions is vital for plant survival and productivity. In summary, plant-specific organelles such as chloroplasts, vacuoles, and various types of plastids are indispensable for the adaptation of plants to their environmental conditions. These organelles enable plants to harness energy from sunlight, maintain cellular homeostasis, store nutrients, and respond to environmental stimuli—all of which are essential for their survival and success in diverse ecosystems. The importance of these organelles underscores the unique biological strategies that have evolved in plants to thrive in a wide range of environments.